Embryology Lesson 4: Week 4 Development PDF
Document Details
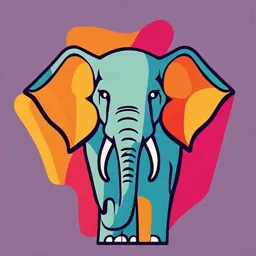
Uploaded by SereneChrysoprase640
University of Kurdistan Hewlêr
Tags
Related
- Embryology: Early Development Weeks 2-4 PDF
- Lecture 5 - Third to Eighth Weeks Development - Embryonic Period - 22 April 2024 PDF
- Fetal Growth & Development Stages (Weekly) PDF
- Tercera semana de desarrollo embrionario (PDF)
- 4-8 Week Development of Human Embryo - Organogenesis PDF
- Embriologija Skripta PDF
Summary
This document provides a detailed overview of week 4 of embryonic development, covering organ system development, and nervous system development, including neurulation and neural crest cells. It also touches on brain development and the formation of primary and secondary brain vesicles.
Full Transcript
Week 4 of Embryonic Development: Detailed Overview of Organ and System Development The fourth week of embryonic development is a pivotal period where the groundwork for the human body and organ systems is established. Below is an extensive, detailed account of each organ and system mentioned in the...
Week 4 of Embryonic Development: Detailed Overview of Organ and System Development The fourth week of embryonic development is a pivotal period where the groundwork for the human body and organ systems is established. Below is an extensive, detailed account of each organ and system mentioned in the initial overview. 1. Morphological Changes: Folding of the Embryo Cranial Folding The cranial region of the embryo bends ventrally due to the rapid growth of the neural tube and somites. The forebrain develops prominently, positioning itself above the primitive heart. Structures like the oropharyngeal membrane and developing stomodeum (future mouth) move into their definitive positions. Caudal Folding At the tail end, the caudal region folds ventrally, driven by the lengthening of the neural tube. The connecting stalk (future umbilical cord) shifts ventrally to align with the gut tube and other embryonic structures. Lateral Folding Simultaneously, the lateral edges of the embryonic disc fold inward. This process closes off the intraembryonic coelom, giving rise to the primitive thoracic and abdominal cavities. The folding process also encloses the gut tube, which will later differentiate into the gastrointestinal tract. Development of the Nervous System: Detailed Explanation The nervous system is one of the earliest systems to begin developing in the embryo, and its proper formation is critical for overall embryonic development. This process includes neurulation, the migration of neural crest cells, and the differentiation of the brain and spinal cord. 1. Neurulation Neurulation is the process by which the neural plate forms the neural tube, which later develops into the central nervous system (CNS), comprising the brain and spinal cord. This begins during the third week of embryonic development and is completed by the fourth week. Steps of Neurulation: 1. Formation of the Neural Plate: o The ectoderm above the notochord thickens to form the neural plate under the influence of signaling molecules like Sonic Hedgehog (SHH) from the notochord. o This marks the first visible step of CNS development. 2. Shaping and Folding: o The lateral edges of the neural plate elevate to form the neural folds, with a central depression called the neural groove. o The folds approach each other and begin to fuse. 3. Closure of the Neural Tube: o The neural tube closes first in the cervical region (future neck) and progresses bidirectionally (both cranially and caudally). o The cranial and caudal ends of the tube remain temporarily open as neuropores: ▪ Cranial neuropore closes around day 25. ▪ Caudal neuropore closes around day 27. 4. Formation of Brain and Spinal Cord: o The closed cranial portion forms the brain, while the caudal portion forms the spinal cord. Clinical Correlations: Failure of neural tube closure can lead to: o Spina Bifida: Incomplete closure of the caudal neuropore. o Anencephaly: Incomplete closure of the cranial neuropore, resulting in a lack of brain development. 2. Neural Crest Cells Neural crest cells are a group of multipotent cells that arise at the junction of the neural plate and ectoderm during neurulation. These cells undergo epithelial-to-mesenchymal transition (EMT) and migrate extensively throughout the embryo, contributing to various structures. Contributions of Neural Crest Cells: 1. Peripheral Nervous System: o Sensory and autonomic ganglia (e.g., dorsal root ganglia, sympathetic and parasympathetic ganglia). o Schwann cells (supportive cells of peripheral nerves). o Satellite cells. 2. Craniofacial Structures: o Cartilage and bones of the face and skull. o Connective tissues in the craniofacial region. 3. Endocrine and Cardiac Structures: o Adrenal medulla: Chromaffin cells that produce adrenaline. o Parts of the heart, including the conotruncal septum, which divides the outflow tract into the aorta and pulmonary artery. 4. Pigment Cells: o Melanocytes: Cells responsible for skin pigmentation. 5. Other Structures: o Portions of the meninges (coverings of the brain and spinal cord). o Smooth muscle cells of certain blood vessels. Clinical Correlations: Abnormal neural crest cell migration or differentiation can result in: o Hirschsprung Disease: Lack of enteric ganglia in the intestinal wall. o Cleft palate: Failure of craniofacial structures to fuse properly. o Neurofibromatosis: Tumors of neural crest-derived Schwann cells. 3. Brain Development The cranial end of the neural tube expands and differentiates into the brain. This occurs in stages, starting with the formation of primary brain vesicles during the fourth week, which further divide into secondary vesicles by the fifth week. Primary Brain Vesicles: 1. Forebrain (Prosencephalon): o Expands into two secondary vesicles: ▪ Telencephalon: Forms the cerebral hemispheres and lateral ventricles. ▪ Diencephalon: Forms the thalamus, hypothalamus, epithalamus, and the third ventricle. 2. Midbrain (Mesencephalon): o Remains as a single vesicle and forms: ▪ Structures of the brainstem (e.g., tectum and tegmentum). ▪ Pathways involved in visual and auditory processing. 3. Hindbrain (Rhombencephalon): o Divides into: ▪ Metencephalon: Forms the pons and cerebellum. ▪ Myelencephalon: Forms the medulla oblongata. Structural Development: 1. Cerebral Hemispheres: o Begin to expand and grow over the midbrain and diencephalon. o Form the cortex responsible for higher cognitive functions like memory, reasoning, and voluntary movements. 2. Brainstem and Spinal Cord: o The brainstem develops from the midbrain and hindbrain regions, responsible for vital reflexes and autonomic functions. o The spinal cord forms from the caudal neural tube and grows in length to match the elongation of the body. Clinical Correlations: Disorders of brain development include: o Holoprosencephaly: Failure of the prosencephalon to divide into hemispheres. o Microcephaly: Reduced brain size, often linked to genetic or environmental factors (e.g., Zika virus). Summary of Key Processes: Process Structure Formed Clinical Correlation Neurulation Neural tube → Brain and spinal cord Spina bifida, anencephaly Neural Crest Peripheral nerves, craniofacial bones, Hirschsprung disease, cleft Cells melanocytes, adrenal medulla palate Brain Holoprosencephaly, Forebrain, midbrain, hindbrain Development microcephaly The coordinated development of these processes ensures the proper formation of the central and peripheral nervous systems, as well as structures vital for craniofacial and systemic function. Development of the Musculoskeletal System: Detailed Explanation The musculoskeletal system develops primarily from mesodermal germ layers during embryogenesis, with significant contributions from somites. These paired blocks of mesoderm are arranged segmentally along the embryo's axis and play a pivotal role in forming the vertebrae, ribs, skeletal muscles, and dermis. 1. Formation of Somites Origination: Somites originate from the paraxial mesoderm, which lies adjacent to the neural tube and notochord. During the third week of development, the paraxial mesoderm segments into paired structures called somites along the cranial-to-caudal axis. Somite Development: The first somites appear in the occipital region around day 20, and somite formation continues at a rate of about 3–4 pairs per day until the end of the fifth week. A human embryo typically has 42–44 pairs of somites, but some undergo resorption, leaving 37 pairs. Somite Segmentation: Somites are arranged in the following regions:. Occipital (4 pairs): Contribute to the base of the skull.. Cervical (8 pairs): Form the vertebrae of the neck.. Thoracic (12 pairs): Form thoracic vertebrae and ribs.. Lumbar (5 pairs): Form lumbar vertebrae.. Sacral (5 pairs): Form the sacrum.. Coccygeal (8–10 pairs): Contribute to the coccyx. 2. Differentiation of Somites Each somite undergoes differentiation into three distinct regions, each giving rise to specific tissues: A. Sclerotome (Vertebrae and Ribs) The sclerotome forms from the ventral and medial portions of each somite. Sclerotomal cells migrate medially toward the notochord and neural tube to form:. Vertebrae: ▪ The cells condense around the notochord to form the vertebral bodies. ▪ They also surround the neural tube to form the vertebral arches.. Ribs: ▪ In the thoracic region, sclerotome cells extend laterally to develop into costal processes, which become ribs. Key Process: Re-segmentation: Each sclerotome divides into cranial and caudal halves. Adjacent halves fuse to form a single vertebra, ensuring proper alignment of muscles and nerves between vertebrae. Clinical Correlation: Abnormal sclerotome development can result in: o Spinal defects like spina bifida (failure of vertebral arch closure). o Scoliosis (lateral curvature of the spine). B. Dermatome (Dermis of the Skin) The dermatome forms from the lateral portion of the somite. Dermatome cells migrate to specific regions and differentiate into the dermis (the connective tissue layer of the skin) in the corresponding segmental regions of the body. Clinical Correlation: The dermatome pattern corresponds to segmental innervation by spinal nerves. Disorders of dermatome migration or differentiation can lead to abnormalities in skin structure or segmental sensory deficits. C. Myotome (Skeletal Muscles) The myotome develops from the dorsal portion of the somite and gives rise to skeletal muscles of the trunk and limbs. Myotomal cells migrate and divide into two major groups:. Epaxial Muscles: ▪ Located dorsally, these muscles form the deep muscles of the back. ▪ Innervated by the dorsal primary rami of spinal nerves.. Hypaxial Muscles: ▪ Located ventrally, these muscles give rise to: ▪ Intercostal and abdominal wall muscles. ▪ Limb muscles. ▪ Innervated by the ventral primary rami of spinal nerves. Muscle Formation in Limbs: Myotomal cells from the cervical and lumbar regions migrate into the limb buds. These cells differentiate into: o Flexor muscles (ventral side of the limb). o Extensor muscles (dorsal side of the limb). Clinical Correlation: Disruptions in myotome development or migration can lead to: o Congenital muscular dystrophies. o Poland syndrome: Absence of chest wall muscles. 3. Axial Skeleton Formation The axial skeleton includes the vertebrae, ribs, and sternum, and develops from the sclerotome. The notochord persists as the nucleus pulposus of the intervertebral discs. 4. Appendicular Skeleton Formation The bones of the limbs develop from the lateral plate mesoderm. Limb buds consist of mesenchyme surrounded by ectoderm. The mesenchyme condenses and differentiates into cartilage models, which later ossify to form bones. Summary of Somite Contributions Somite Component Structure Formed Region Sclerotome Vertebrae, ribs, and intervertebral discs Axial skeleton Dermatome Dermis of the skin Segmental body regions Myotome Skeletal muscles of back, trunk, and limbs Epaxial and hypaxial muscles The segmentation and differentiation of somites are tightly regulated processes that lay the foundation for the musculoskeletal system's structure and function. Abnormalities in somite development can have widespread effects on the vertebral column, musculature, and skin. Cardiovascular System: Detailed Overview The cardiovascular system is the first functional organ system to form during embryonic development, with the heart beginning to beat around day 22. Its development in the fourth week is critical for establishing a functional circulatory system to sustain the rapidly growing embryo. Below are the detailed processes of Heart Tube Formation and Early Circulation: Heart Tube Formation The heart initially forms as a straight tubular structure that develops from the mesoderm in the cardiogenic region of the embryo. The key steps include: 1. Primary Heart Field: o The cardiogenic mesoderm migrates cranially during gastrulation and positions itself above the oropharyngeal membrane. o This mesoderm gives rise to the two endocardial tubes that will later fuse into the heart tube. 2. Formation of the Single Heart Tube: o Around day 19, two endocardial tubes form from angiogenic cell clusters in the lateral plate mesoderm. o These two tubes merge at the midline (due to lateral embryonic folding) to form a single primitive heart tube by day 21. 3. Heart Tube Segments: o The heart tube elongates and begins to show segmentation: ▪ Sinus venosus: The inflow region, receiving blood from vitelline, umbilical, and cardinal veins. ▪ Primitive atrium: Forms the future atria. ▪ Primitive ventricle: Forms the left ventricle. ▪ Bulbus cordis: Contributes to the right ventricle and outflow tracts. ▪ Truncus arteriosus: Develops into the aorta and pulmonary trunk. 4. Cardiac Looping: o By day 23, the heart tube begins to undergo looping to establish its correct spatial orientation (right-left asymmetry). o The process involves: ▪ Bending of the tube into an S-shaped structure. ▪ The primitive atrium and sinus venosus move dorsally and cranially. ▪ The primitive ventricle and bulbus cordis shift ventrally and caudally. 5. First Heartbeat: o The heart begins to beat rhythmically by day 22, driven by spontaneous contractions of cardiac muscle cells. o Although initially uncoordinated, this activity allows the heart to pump blood through primitive vascular circuits. Early Circulation By the end of the fourth week, the heart is actively pumping blood through a simple circulatory system that links the embryo to the yolk sac, placenta, and developing vascular system. 1. Primitive Blood Vessels: o The vasculature forms through vasculogenesis (formation of blood vessels from angioblasts) and angiogenesis (sprouting of new vessels from existing ones). o These vessels connect to the heart tube, creating the initial circulatory circuits. 2. Circulatory Pathways: o Vitelline Circulation: ▪ Links the embryo to the yolk sac, facilitating nutrient uptake and waste removal. ▪ Blood flows from the yolk sac into the embryo through the vitelline veins and returns via the vitelline arteries. o Umbilical Circulation: ▪ Connects the embryo to the placenta, allowing oxygen and nutrient exchange with maternal blood. ▪ Oxygenated blood is transported via the umbilical vein, while deoxygenated blood returns through the umbilical arteries. o Embryonic Circulation: ▪ The embryo’s tissues are supplied with blood via paired dorsal aortae and cardinal veins. 3. Hematopoiesis: o Blood cell formation begins in the yolk sac during the third week and contributes to early circulatory function. o Primitive blood cells (erythroblasts) help transport oxygen in the absence of fully functional lungs. Clinical Significance 1. Cardiac Looping Defects: o Abnormalities in heart tube looping can lead to congenital heart defects such as dextrocardia or heterotaxy syndrome. 2. Vulnerability to Teratogens: o During this period, the heart is highly susceptible to teratogens, which can disrupt its formation and result in congenital anomalies like ventricular septal defects (VSDs) or atrial septal defects (ASDs). 3. Foundation for Advanced Structures: o The primitive structures formed in week 4 serve as the scaffolding for later division into the four-chambered heart and the development of specialized structures like valves. The fourth week of embryonic cardiovascular development lays the groundwork for the complex circulatory system that will support the growing embryo and later sustain independent life postnatally. Extensive detail of 9. Limb Bud Formation Upper Limb Buds: Appear as small protrusions on the lateral sides of the embryo by day 26. Lower Limb Buds: Begin to form shortly thereafter. These buds contain mesenchymal tissue from the somatic lateral plate mesoderm and ectoderm, which coordinate to establish the basic limb structure. Limb Bud Formation: Week 4 of Embryonic Development The formation of limb buds during week 4 of embryonic development marks the beginning of limb morphogenesis, where the basic framework for the upper and lower limbs is established. This process is highly orchestrated and involves coordinated interactions between mesodermal and ectodermal tissues, signaling pathways, and genetic regulation. Below is a detailed account of the Upper Limb Buds and Lower Limb Buds, including the cellular and molecular processes involved. Upper Limb Bud Formation 1. Timing and Location: o The upper limb buds appear around day 26 as small protrusions on the lateral sides of the embryo at the level of somites C5 to T1. o These buds are positioned near the cervical region of the embryo and are the first signs of future upper limb development. 2. Cellular Composition: o The mesenchymal core: ▪ Derived from the somatic lateral plate mesoderm. ▪ Provides the precursor cells for skeletal elements, connective tissues, and some musculature. o The ectodermal covering: ▪ The ectoderm thickens at the distal end of the limb bud to form the apical ectodermal ridge (AER) by the fifth week. ▪ The AER is critical for limb outgrowth and differentiation. 3. Signaling Pathways: o Fibroblast Growth Factors (FGFs): ▪ FGFs secreted by the AER stimulate proliferation and growth of the underlying mesenchyme. o Sonic Hedgehog (SHH): ▪ Produced by the zone of polarizing activity (ZPA) located at the posterior base of the limb bud. ▪ SHH establishes the anterior-posterior axis of the limb (e.g., thumb vs. little finger). o Wnt Signaling: ▪ Coordinates the dorsal-ventral patterning of the limb bud. 4. Initial Structural Formation: o The limb bud grows as mesenchymal cells proliferate under the influence of signals from the AER and ZPA. o These cells later differentiate into the cartilage models of bones (e.g., humerus, radius, ulna) and other components of the upper limb. Lower Limb Bud Formation 1. Timing and Location: o The lower limb buds begin to form a few days later than the upper limb buds, around day 28, at the level of somites L1 to S2. o Positioned near the lumbar region of the embryo, these buds are the precursors to the lower limbs. 2. Cellular Composition: o Similar to the upper limb buds, the lower limb buds contain: ▪ Mesenchyme from the somatic lateral plate mesoderm. ▪ An ectodermal covering that eventually forms the AER. 3. Signaling Pathways: o The molecular mechanisms for lower limb development mirror those of the upper limb, with FGFs, SHH, and Wnt signaling playing key roles. o Differences in limb identity (e.g., upper vs. lower limb) are specified by T-box genes (TBX5 for upper limbs, TBX4 for lower limbs) and Pitx1. 4. Initial Structural Formation: o Mesenchymal proliferation within the lower limb buds contributes to the development of the future bones (e.g., femur, tibia, fibula), connective tissues, and musculature. o The growth of the lower limb bud is slightly delayed compared to the upper limb, reflecting the overall developmental sequence. Coordinated Limb Development 1. Mesoderm Contribution: o Somatic Lateral Plate Mesoderm: ▪ Provides mesenchymal cells for the skeletal and connective tissue components of the limbs. o Paraxial Mesoderm (Somites): ▪ Contributes to the myogenic precursors that migrate into the limb buds to form skeletal muscles. 2. Ectoderm Contribution: o The ectoderm not only covers the limb buds but also plays a pivotal role in signaling and patterning. o The formation of the AER is critical for limb outgrowth, as it provides key signals to maintain mesenchymal proliferation. 3. Three Axes of Limb Patterning: o Proximal-Distal Axis: ▪ Controlled by FGFs from the AER. ▪ Determines structures like the shoulder (proximal) vs. hand (distal). o Anterior-Posterior Axis: ▪ Regulated by SHH from the ZPA. ▪ Establishes digit identity (e.g., thumb vs. little finger). o Dorsal-Ventral Axis: ▪ Governed by Wnt signaling from the ectoderm. ▪ Dictates the dorsal (e.g., back of hand) vs. ventral (e.g., palm) orientation. Clinical Significance 1. Congenital Limb Defects: o Disruptions in limb bud formation can lead to various congenital abnormalities, such as: ▪ Amelia: Complete absence of a limb. ▪ Meromelia: Partial absence of a limb. ▪ Polydactyly: Extra digits due to altered SHH signaling. ▪ Syndactyly: Fused digits resulting from improper apoptosis. o Mutations in key signaling pathways or environmental factors (e.g., teratogens) can interfere with normal development. 2. Prosthetic and Regenerative Research: o Understanding limb development provides insights into regenerative medicine, prosthetic advancements, and tissue engineering. Summary of Week 4 Limb Development The upper limb buds appear by day 26, while lower limb buds form by day 28. The mesenchymal core and ectodermal covering interact through molecular signaling pathways to shape the early limb structures. Limb bud growth is tightly regulated along three axes: proximal-distal, anterior-posterior, and dorsal-ventral. These processes lay the foundation for the differentiation of bones, muscles, and other limb tissues, which continues in subseq Pharyngeal Arches: Detailed Development and Derivatives The pharyngeal arches are a series of tissue structures that appear in the developing embryo during the fourth week of gestation. These arches play a crucial role in the development of the head, neck, and face. They are paired structures that form on both sides of the embryonic pharynx, contributing to various skeletal, muscular, and vascular components of these regions. Each pharyngeal arch contains: 1. A core of mesoderm and neural crest cells. 2. An outer covering of ectoderm. 3. An inner lining of endoderm. 4. Its own artery, nerve, cartilage, and muscular component. The First Pharyngeal Arch (Mandibular Arch) Appearance: The first pharyngeal arch is visible by the end of the fourth week. Key Contributions: It divides into two processes:. Maxillary Process: Contributes to the upper jaw.. Mandibular Process: Contributes to the lower jaw. Derivatives: 1. Skeletal Structures: o Maxillary Process: ▪ Forms the maxilla, zygomatic bone, and part of the temporal bone. o Mandibular Process: ▪ Forms the mandible (via intramembranous ossification). o Meckel’s Cartilage: ▪ The cartilaginous template of the mandibular process; parts of it degenerate while contributing to: ▪ The malleus and incus of the middle ear. 2. Muscular Structures: o Muscles of Mastication: ▪ Masseter, temporalis, medial pterygoid, and lateral pterygoid. o Other Muscles: ▪ Mylohyoid, anterior belly of the digastric, tensor tympani, and tensor veli palatini. 3. Nerve Supply: o Mandibular division of the Trigeminal Nerve (CN V3): ▪ Provides motor innervation to the muscles of mastication and sensory innervation to the mandibular region. 4. Arterial Supply: o The first aortic arch partially persists to contribute to the maxillary artery. The Second Pharyngeal Arch (Hyoid Arch) Appearance: Shortly after the first arch, the second pharyngeal arch develops by the end of the fourth week. Key Contributions: The second arch contributes to the formation of the hyoid apparatus and structures associated with the ear. Derivatives: 1. Skeletal Structures: o Reichert’s Cartilage: ▪ Gives rise to: ▪ The stapes (middle ear). ▪ The styloid process of the temporal bone. ▪ The lesser horn and upper part of the body of the hyoid bone. ▪ The stylohyoid ligament. 2. Muscular Structures: o Muscles of Facial Expression: ▪ Frontalis, orbicularis oculi, orbicularis oris, buccinator, platysma, and others. o Other Muscles: ▪ Stapedius, stylohyoid, and the posterior belly of the digastric. 3. Nerve Supply: o Facial Nerve (CN VII): ▪ Provides motor innervation to the muscles of facial expression and sensory components to parts of the ear. 4. Arterial Supply: o The second aortic arch gives rise to: ▪ The stapedial artery (transient in humans) and parts of the hyoid artery. Pharyngeal Arch Components Each pharyngeal arch contributes distinct elements to the developing head and neck: 1. Mesoderm: o Forms muscles and arteries. 2. Neural Crest Cells: o Differentiate into bones, cartilage, and connective tissues. 3. Endoderm: o Lines the internal structures (e.g., pharyngeal pouches). Clinical Correlations 1. First Arch Syndromes: o Treacher Collins Syndrome: ▪ Results from abnormal development of the first arch neural crest cells. ▪ Leads to facial malformations such as hypoplasia of the zygomatic bones and mandible. o Pierre Robin Sequence: ▪ Characterized by micrognathia, glossoptosis, and cleft palate. 2. Second Arch Abnormalities: o Congenital Stapes Fixation: ▪ Can result from improper development of Reichert’s cartilage, leading to hearing loss. Summary First Pharyngeal Arch: o Key structures: Maxilla, mandible, malleus, incus. o Muscles: Muscles of mastication. o Nerve: CN V3. Second Pharyngeal Arch: o Key structures: Hyoid bone (upper part), stapes, styloid process. o Muscles: Muscles of facial expression. o Nerve: CN VII. These arches form the foundational structures for the head and neck, and their development is tightly regulated by genetic and signaling mechanisms.