Biological Macromolecules PDF
Document Details
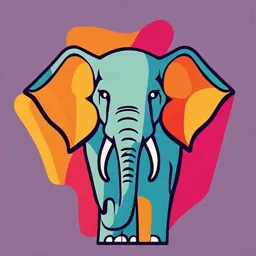
Uploaded by GenialSalmon3599
null
false
null
Tags
Summary
This document provides an overview of biological macromolecules, discussing important topics such as their role in living organisms, dehydration and hydrolysis reactions, carbohydrates, lipids and more, with examples and figures.
Full Transcript
3 Biological Macromolecules In this section, we will be exploring the essential role that large molecules play in the functioning and health of living organisms. From providing the building blocks for our bodies to serving as sources of energy and genetic information, macromolecules are vital to lif...
3 Biological Macromolecules In this section, we will be exploring the essential role that large molecules play in the functioning and health of living organisms. From providing the building blocks for our bodies to serving as sources of energy and genetic information, macromolecules are vital to life as we know it. But while macromolecules are necessary for proper function, an imbalance of any of them can negatively affect our health. For exam- ple, too much fat can lead to cardiovascular problems, while excess protein can strain the kidneys. Maintaining a balanced diet is impor- tant to ensure that we get the right amount of each macromolecule for optimal health. It is also important to pay attention to the amount of sugar con- sumed. While small amounts of sugar can be beneficial for providing quick energy, consuming too much of it can lead to weight gain and an increased risk of type 2 diabetes. On the other hand, cutting out sugar entirely can also be harmful, as it can lead to a lack of energy and nutrient deficiencies. Striking the right balance is key to maintaining good health. As we delve deeper into this chapter, we will examine the different types of macromolecules and their specific functions in the body. So let’s get started on our journey to understanding these essential macromolecules! 3.1 Dehydration Synthesis and Hydrolysis Dehydration reactions, also known as condensation reactions, are anabolic chemical reactions that involve the removal of a single EnterMedSchool.com 39 Alpha Version water molecule from two or more molecules, which are then com- bined to form a larger molecule. The reaction yields a single water molecule as a byproduct and connects the two molecules together. Dehydration reactions are important in biology because they help cells to create new macromolecules, such as proteins, nucleic acids, and carbohydrates. Figure 5: In the dehydration synthesis reaction depicted above, two molecules of glucose are linked together to form the disaccharide maltose. In the process, a water molecule is formed. (Credit: open- stax.org) For example, when two glucose molecules are linked together through a dehydration reaction, a disaccharide called maltose is formed, as shown in the figure above. Similarly, when two amino acids are joined together, a dehydration reaction occurs, and a bond called a peptide bond is formed between them to create a new molecule called a dipeptide. Peptide bonds can be strung together to form a long chain of amino acids, called a protein. Hydrolysis reactions, on the other hand, are catabolic chemical re- actions that involve the addition of water molecules to break down larger molecules into smaller ones. Hydrolysis reactions are impor- tant in biology because they help cells to break down and use the energy stored in macromolecules such as sugars, proteins, and fats. Hydrolysis reactions are also important for breaking down other macromolecules such as nucleic acids and proteins. Nucleic acids, which are made up of nucleotides, can be broken down into indi- EnterMedSchool.com 40 Alpha Version vidual nucleotides through hydrolysis reactions. Similarly, proteins can be broken down into individual amino acids through hydrolysis reactions. Figure 6: In the hydrolysis reaction shown here, the disaccharide maltose is broken down to form two glucose monomers with the addition of a water molecule. Note (Credit: openstax.org) When our bodies digest food, they use hydrolysis reactions to break down carbohydrates, proteins, and fats into smaller molecules that can be used for energy. For example, the hydrolysis of maltose, shown in the figure above, breaks the disaccharide down into two glucose monomers with the addition of a water molecule. In summary, dehydration and hydrolysis reactions are important chemical processes in biology. Dehydration reactions involve the removal of a single water molecule to create a larger molecule, while hydrolysis reactions involve the addition of water molecules to break down larger molecules into smaller ones. These reactions are cru- cial for the synthesis and breakdown of macromolecules in living organisms. 3.2 Carbohydrates Carbohydrates are one of the most important biomolecules found in living organisms. They are the primary source of energy for our bodies and play a critical role in providing structural support. Car- EnterMedSchool.com 41 Alpha Version bohydrates are made up of carbon, hydrogen, and oxygen atoms, and they are classified into three main types based on the number of monomers they contain: monosaccharides, disaccharides, and polysaccharides. Monosaccharides, such as glucose, fructose, and galactose, are sim- ple sugars that cannot be broken down into smaller sugars. They are joined together through dehydration synthesis, a process we discussed earlier, to form more complex carbohydrates. Disaccharides, like sucrose (table sugar), are made up of two monosac- charides that are linked together by a glycosidic bond. Disaccharides are an important source of energy for our bodies and are often found in sweet foods like candy and soda. Polysaccharides, such as starch, glycogen, and cellulose, are complex carbohydrates made up of many monosaccharide units. They are used for energy storage and provide structural support to cells and tissues. Plants store energy in the form of starch, while animals store energy in the form of glycogen. Cellulose, on the other hand, provides structural support to the cell walls of plants. Insects have a hard outer skeleton made of chitin, a polysaccha- ride that contains nitrogen. Chitin provides structural support and protection to insects, making it an important component of their exoskeletons. In summary, carbohydrates are an essential biomolecule found in liv- ing organisms, providing energy and structural support. They come in different forms, from simple sugars to complex polysaccharides, and play important roles in the functioning of our bodies and the environment around us. Carbohydrates are organic molecules that contain carbon, hydro- gen, and oxygen atoms in the general formula Cn (H2 O)n. The simplest EnterMedSchool.com 42 Alpha Version Figure 7: Monosaccharides are classified based on the position of their carbonyl group and the number of carbons in the backbone. Aldoses have a carbonyl group (indicated in green) at the end of the carbon chain, and ketoses have a carbonyl group in the middle of the carbon chain. Trioses, pentoses, and hexoses have three-, five-, and six-carbon backbones, respectively. Note (Credit: openstax.org) EnterMedSchool.com 43 Alpha Version carbohydrates are monosaccharides, which are single sugars that cannot be broken down into simpler sugars. Monosaccharides have a general formula of Cn H2n On , where n is typically 3 to 7. Monosaccha- rides can be classified into three categories based on the number of carbon atoms they contain: trioses (3 carbons), tetroses (4 carbons), and pentoses (5 carbons). The most common monosaccharides are hexoses, which have six carbon atoms. Disaccharides are formed when two monosaccharides are joined together through a glycosidic bond, which is formed by a dehydra- tion reaction between two monosaccharides. During this reaction, a molecule of water is removed as two monosaccharides are linked together to form a single disaccharide. A glycosidic bond is a covalent bond that links two monosaccha- rides together. This bond is formed when a hydroxyl group (-OH) on one monosaccharide reacts with the anomeric carbon of another monosaccharide, which is a carbon atom that is part of a cyclic hemiacetal structure. The reaction involves the removal of a water molecule, which creates a covalent bond between the two monosac- charides. The resulting molecule is a disaccharide, which is a sugar that consists of two monosaccharides linked together. Common disaccharides include sucrose, lactose, and maltose. Su- crose, also known as table sugar, is composed of glucose and fruc- tose, while lactose, found in milk, is composed of glucose and galac- tose. Maltose, found in germinating grains, is composed of two glucose molecules linked together. Remember: To find a chemical formula of a disaccharide we need to add both molecules together and deduce a single water molecule. Polysaccharides are long chains of monosaccharides that are joined together by glycosidic bonds. They can be classified into two cate- EnterMedSchool.com 44 Alpha Version Figure 8: Sucrose is formed when a monomer of glucose and a monomer of fructose are joined in a dehydration reaction to form a glycosidic bond. In the process, a water molecule is lost. By conven- tion, the carbon atoms in a monosaccharide are numbered from the terminal carbon closest to the carbonyl group. In sucrose, a gly- cosidic linkage is formed between carbon 1 in glucose and carbon 2 in fructose. (Credit: openstax.org) EnterMedSchool.com 45 Alpha Version Figure 9: Common disaccharides include maltose (grain sugar), lactose (milk sugar), and sucrose (table sugar). Note (Credit: open- stax.org) EnterMedSchool.com 46 Alpha Version gories: homopolysaccharides and heteropolysaccharides. Homopolysac- charides are composed of a single type of monosaccharide, while heteropolysaccharides are composed of multiple types of monosac- charides. Polysaccharides are important for energy storage and structural support in living organisms. The most common kinds of polysaccharides are glycogen, cellulose, amylose, and amylopectin. Glycogen is like a storage unit for glucose in our body that’s made up of individual glucose molecules. The glucose monomers are linked via α-1,4 linkages and there is branching linked via α-1,6 linkages. It’s found in the liver and muscles and acts like starch but in animals. When our blood sugar gets too low, glycogen is broken down to release glucose in a process called glycogenolysis. Cellulose is a natural material found in plant cell walls that gives them structure and support. It’s the most common type of biopoly- mer in nature and makes up things like wood and paper. Cellulose is made up of glucose molecules that are linked together via by β 1-4 glycosidic linkages. In contrast, starch, a plant storage carbohydrate made of amylose and amylopectin, is made with glucose linked via α-1,4 or α-1,6 glycosidic linkages. Amylose consists of unbranched chains (only α-1,4), while amylopectin has additional α-1,6 linkages that are a result of branching (so it has both α-1,4 and α-1,6 linkages). 3.3 Amino Acids Amino acids are small molecules that serve as the building blocks of proteins, which are essential for life. Proteins have many functions in our bodies, such as helping our muscles move and supporting our immune system. Amino acids play a crucial role in determining the structure and function of proteins, and their unique properties allow them to form different types of proteins with specific functions. A EnterMedSchool.com 47 Alpha Version Figure 10: In cellulose, glucose monomers are linked in unbranched chains by β 1-4 glycosidic linkages. Because of the way the glucose subunits are joined, every glucose monomer is flipped relative to the next one resulting in a linear, fibrous structure.(Credit: openstax.org) EnterMedSchool.com 48 Alpha Version Figure 11: Amylose and amylopectin are two different forms of starch. Amylose is composed of unbranched chains of glucose monomers connected by α-1,4 glycosidic linkages. Amylopectin is composed of branched chains of glucose monomers connected by α-1,4 and α-1,6 glycosidic linkages. Because of the way the subunits are joined, the glucose chains have a helical structure. Glycogen (not shown) is similar in structure to amylopectin but more highly branched. Note (Credit: openstax.org) EnterMedSchool.com 49 Alpha Version balanced and varied diet is important for obtaining the different types of amino acids needed for protein synthesis in our bodies.. Amino acids are small molecules that serve as the building blocks of proteins, which are essential for life. Each amino acid has a specific structure consisting of a central chiral carbon atom, called alpha carbon. A chiral carbon is a carbon atom that is bonded to four different substituents. In addition to the alpha carbon, amino acids have an amino group (-NH2) this is the ”Amino” part in ”Amino Acid”. The second group is a carboxyl group (-COOH) which is made up of carbon, oxygen, and hydrogen atoms and serves as the acidic part of the amino acid. The third group is the variable side chain (-R). The unique structure of each amino acid is determined by the nature of its side chain. The side chain can be a simple hydrogen atom, or it can be a complex structure that contains functional groups such as hydroxyl (-OH), sulfhydryl (-SH), or amino (-NH2). These functional groups give the amino acids their unique chemical and physical properties, which play a crucial role in the formation of proteins and their functions in our bodies. The last substituent bonded to the alpha carbon is just a single hydrogen atom, which completes the four bonds carbon loves to have. An important exemption to the rule is the amino acid Glycine. Glycine has a single Hydrogen atom as its R group, so Glycine’s carbon isn’t a chiral carbon. Great! Now that we understand the basic building block, let’s talk about the four structural levels of proteins. The primary structure of a protein is the linear sequence of amino acids that make up the protein chain (the order of amino acids). The EnterMedSchool.com 50 Alpha Version Figure 12: Amino acids have a central asymmetric carbon to which an amino group, a carboxyl group, a hydrogen atom, and a side chain (R group) are attached. (Credit: openstax.org) specific sequence of amino acids determines the unique character- istics and function of the protein. The secondary structure of a protein is the way the protein folds up into a regular pattern or shape, such as an alpha helix or beta sheet. These shapes are stabilized by hydrogen bonds between the amino acids. The tertiary structure of a protein is the way the protein folds up even further to create a three-dimensional shape. This structure is critical for the protein’s function, as it determines the way that the EnterMedSchool.com 51 Alpha Version Figure 13: There are typically 20 common amino acids commonly found in proteins, each with a different R group (variant group) that determines its chemical nature.(Credit: openstax.org) EnterMedSchool.com 52 Alpha Version protein interacts with other molecules in the cell. Tertiary structure is stabilized by various interactions such as hydrogen bonds, disulfide bridges, van der Waals interactions, and hydrophobic interactions. Finally, the quaternary structure of a protein is the way multiple protein subunits come together to form a larger complex. This structure is found in proteins that have more than one polypeptide chain. Quaternary structure is important for the function of many proteins, such as enzymes, which often require multiple subunits to work properly. 3.3.1 Primary Structure The primary structure of a protein is the linear sequence of amino acids that make up the protein. It’s called the primary structure because it’s the first level of organization of the protein. Amino acids are joined together in a protein by a type of chemical bond called a peptide bond. When an amino acid is joined to an- other amino acid, the carboxyl group of one amino acid reacts with the amino group of the other amino acid, and a water molecule is released. This reaction is another example of a dehydration reaction which we discussed earlier. The reaction creates a bond between the two amino acids, and the result is a long chain of amino acids. The primary structure of a protein is important because it will even- tually determine the three-dimensional shape of the protein, which in turn determines the protein’s function. Different sequences of amino acids can fold into different shapes, and each shape is suited for a specific function. For example, some proteins are enzymes that help chemical reactions happen more quickly, while others are structural proteins that help hold tissues together. It is important to mention that each subsequent protein level relies on the previous EnterMedSchool.com 53 Alpha Version Figure 14: Peptide bond formation is a dehydration synthesis reac- tion. The carboxyl group of one amino acid is linked to the amino group of the incoming amino acid. In the process, a molecule of water is released. (Credit: openstax.org) levels. To understand the primary structure better, imagine that a protein is like a necklace made up of small beads. Each bead represents an amino acid, and the beads are joined together by peptide bonds, which are like the string that holds the beads in place. The sequence of beads on the necklace represents the primary structure of the protein, just like the sequence of amino acids in a protein represents the primary structure of the protein. EnterMedSchool.com 54 Alpha Version Just like a necklace can be made up of different kinds of beads, a protein can be made up of different kinds of amino acids. And just like a necklace can be made in different ways, with the beads arranged in different patterns, a protein can have a different primary structure depending on the sequence of amino acids. Figure 15: Bovine serum insulin is a protein hormone made of two peptide chains, A (21 amino acids long) and B (30 amino acids long). In each chain, primary structure is indicated by three-letter abbre- viations that represent the names of the amino acids in the order they are present. The amino acid cysteine (cys) has a sulfhydryl (SH) group as a side chain. Two sulfhydryl groups can react in the presence of oxygen to form a disulfide (S-S) bond. Two disulfide bonds connect the A and B chains together, and a third helps the A chain fold into the correct shape. Note that all disulfide bonds are the same length, but are drawn different sizes for clarity. (Credit: openstax.org) In sickle cell anemia, a change in just one amino acid out of 600 in the hemoglobin protein causes a major difference in structure and function. This single amino acid change is caused by a mutation in the gene that codes for the hemoglobin protein, which alters the EnterMedSchool.com 55 Alpha Version primary structure of the protein. This change in primary structure causes the hemoglobin protein to clump together, resulting in the characteristic sickle shape of red blood cells. This can lead to a variety of health problems, including reduced oxygen delivery to tissues and organ damage. Figure 16: The beta chain of hemoglobin is 147 residues in length, yet a single amino acid substitution leads to sickle cell anemia. In normal hemoglobin, the amino acid at position seven is glutamate. In sickle cell hemoglobin, this glutamate is replaced by a valine. (Credit: openstax.org) 3.3.2 Secondary Structure The secondary structure of a protein is determined by the way that the chain of amino and carboxyl groups, known as the backbone of the peptide, interact with each other and form hydrogen bonds. EnterMedSchool.com 56 Alpha Version There are two main types of secondary structures: Alpha helices and Beta sheets. An alpha helix is a long, spiral structure that’s held together by hydrogen bonds between the amino acids. It looks a bit like a twisted ladder. Beta sheets are flat structures made up of several parallel strands of amino acids held together by hydrogen bonds. They can be either parallel, with the strands running in the same direction, or anti-parallel, with the strands running in opposite directions. Let’s go back to our necklace analogy: Imagine that you want to fold the necklace into a specific shape. You can do this by twisting the necklace into a spiral, like an alpha helix, or by laying the necklace flat and arranging the beads in parallel or antiparallel strands, like a beta-sheet. The way that you fold the necklace represents the secondary structure of the protein, just like the way that a protein folds into an alpha helix or a beta sheet represents the secondary structure of the protein. EnterMedSchool.com 57 Alpha Version Figure 17: The α-helix and β -pleated sheet are secondary structures of proteins that form because of hydrogen bonding between carbonyl and amino groups in the peptide backbone. Certain amino acids have a propensity to form an α-helix, while others have a propensity to form a β -pleated sheet. (Credit: openstax.org) 3.3.3 Tertiary Structure The tertiary structure of a protein is determined by the interac- tions between the side chains (R groups), of the amino acids. Side chains are the groups of atoms that are attached to the alpha car- bon of each amino acid, and they can be different for each amino acid. Some side chains are attracted to water and are said to be EnterMedSchool.com 58 Alpha Version hydrophilic, while others are repelled by water and are said to be hydrophobic. Key Takeaways Remember: The Tertiary structure of a protein is important be- cause it helps determine the overall three-dimensional shape of the protein, which in turn determines the protein’s function. Different Tertiary structures can fold into different shapes, and each shape is suited for a specific function. Now, imagine that each bead has a small group of atoms attached to it, representing the side chains of the amino acids. Some of these groups are hydrophilic, meaning that they’re attracted to water, while others are hydrophobic, meaning that they’re repelled by water. As the protein folds up into its three-dimensional shape, the hy- drophilic side chains will tend to be on the outside of the protein, where they can interact with water, while the hydrophobic side chains will tend to be on the inside of the protein, where they’re shielded from water. This helps stabilize the protein’s tertiary struc- ture and gives the protein its overall three-dimensional shape. There are several types of side chains in amino acids. Disulfide bridges, also known as disulfide bonds, are covalent bonds that form between two cysteine residues in a protein. These bonds are a type of chemical bond that forms when two sulfur atoms are bonded together by a covalent bond. Disulfide bonds play a key role in stabilizing the tertiary structure of proteins, which refers to the three-dimensional shape of the protein. It’s important not to confuse the terms ”cysteine” and ”cystine”. Cys- teine is an amino acid, while cystine is formed when two cysteine EnterMedSchool.com 59 Alpha Version amino acids are joined together by a disulfide bond. Figure 18: The tertiary structure of proteins is determined by a variety of chemical interactions. These include hydrophobic interactions, ionic bonding, hydrogen bonding and disulfide linkages. (Credit: openstax.org) 3.3.4 Quaternary Structure The quaternary structure of a protein is the way that multiple pro- teins come together to form a larger complex. It’s called the Qua- ternary structure because it’s the fourth level of organization of the protein, after the primary structure, the secondary structure, and the tertiary structure. Quaternary structures can be very complex and can involve many different proteins coming together in different ways and shapes. For example, some Quaternary structures are made up of multiple copies of the same protein, while others are made up of different EnterMedSchool.com 60 Alpha Version types of proteins. The Quaternary structure of a protein is important because it deter- mines the overall function of the protein complex. Different Quater- nary structures can perform different functions, and each function is important for the proper functioning of the cell or organism. Hemoglobin is a protein that is made out of four different proteins attached together by hydrophobic interactions, hydrogen bonding, and salt bridges. Hemoglobin is found in red blood cells and is mainly responsible for carrying oxygen from the lungs to the rest of the body. Hemoglobin is a good example of a protein with a Quaternary structure. Hemoglobin is made up of four subunits, each of which is a separate protein. These subunits are called alpha, beta, gamma, and delta, and they’re arranged in a specific way to form the overall structure of hemoglobin. There are several types of hemoglobin, including fetal hemoglobin and adult hemoglobin. Fetal hemoglobin has a higher affinity for oxygen than adult hemoglobin, which allows it to bind more readily to oxygen and transport it to the developing fetus. After birth, the body switches to producing adult hemoglobin, which has a lower affinity for oxygen but is more efficient at releasing oxygen to the tissues. 3.3.5 Hemoglobin Cooperative Binding Hemoglobin cooperative binding refers to the way that hemoglobin binds to oxygen. When oxygen binds to one subunit of hemoglobin, it changes the shape of the protein and makes it easier for the other subunits to bind to oxygen as well. This process is known as cooperative binding, because the binding of one oxygen molecule to EnterMedSchool.com 61 Alpha Version Figure 19: The four levels of protein structure can be observed in these illustrations. (credit: modification of work by National Human Genome Research Institute) (Modified by: openstax.org) EnterMedSchool.com 62 Alpha Version a hemoglobin subunit cooperates with the binding of other oxygen molecules to the other subunits. The cooperative binding of oxygen to hemoglobin allows the protein to bind more oxygen at lower partial pressures of oxygen. This is important because it enables the hemoglobin to extract oxygen from the air in the lungs and deliver it to the tissues where it is needed, even when the partial pressure of oxygen is low. Cooperative binding is a key feature of hemoglobin and is impor- tant for the efficient transport of oxygen throughout the body. It allows the hemoglobin to bind and release oxygen in a way that is responsive to the changing oxygen needs of the body’s tissues, ensuring that they receive a constant supply of oxygen. Therefore, cooperative binding is the result of the Quartanery struc- ture of the Hemoglobin protein! To understand how hemoglobin is structured, imagine that you are a chef in a busy kitchen. Each ingredient has a specific role to play in the recipe. One ingredient might provide flavor, while another might add texture. But while each ingredient can be used independently, they also work together to create a finished dish, and if there is any change in one of the ingredients, it will affect how much of the other ingredients you use as well. Similarly, the subunits of hemoglobin work together to perform the vital task of transporting oxygen throughout the body, just like how ingredients work together to create a tasty dish. 3.3.6 Globular and Fibrous Proteins There are two main categories of protein structure: Globular proteins and Fibrous proteins. EnterMedSchool.com 63 Alpha Version Globular proteins are spherical in shape and are usually soluble in water. They are found in the cytoplasm of cells and perform various functions, including acting as enzymes and transporting molecules within the body. Examples of globular proteins include hemoglobin, and lactoglobulin, which is found in milk. Fibrous proteins, on the other hand, are long and slender and are usually insoluble in water. They are found in tissues such as hair, nails, and skin and provide structural support. Examples of fibrous proteins include collagen, which is found in connective tissue, and keratin, which is found in hair and nails. 3.4 Lipids Lipids are a diverse group of organic molecules that are important for a variety of biological functions. They include fats, waxes, sterols, and certain types of oils. Lipids are characterized by their ability to dissolve in nonpolar solvents, such as ether or olive oil, but not in water. Remember: The saying ”like-dissolves-like” refers to the idea that a substance will dissolve more readily in a solvent that is similar to itself in terms of polarity. Polar substances have an uneven distribution of charge and are more likely to dissolve in polar solvents, while nonpolar substances have an even distribution of charge and are more likely to dissolve in nonpolar solvents. Here are two examples of polar and nonpolar substances: Polar substance: Water (H2O) is a polar substance because it has a partially positive charge on the hydrogen atoms and a partially negative charge on the oxygen atom. This uneven distribution of charge makes it more likely to dissolve polar substances. Nonpolar substance: Hexane (C6H14) is a nonpolar substance because it has an even distribution of charge. It is more EnterMedSchool.com 64 Alpha Version likely to dissolve nonpolar substances. In general, polar substances tend to dissolve polar substances and nonpolar substances tend to dissolve nonpolar substances, following the ”like-dissolves-like” rule. One of the most important roles of lipids is as an energy store. Fats and oils are composed of glycerol and fatty acids, which can be broken down by the body to release large amounts of energy. Lipids are also important structural components of cell membranes, where they help to maintain the integrity and fluidity of the membrane. Sterols, such as cholesterol, are important components of cell mem- branes and are also precursors to hormones and other signaling molecules. Waxes are used for waterproofing and protection in plants and animals, and some lipids, such as the pigment carotenoids, are important for pigmentation and protection from UV radiation. Some common types of lipids include: Triglycerides (fats and oils) Phospholipids (components of cell membranes) Sterols (such as cholesterol) Waxes (such as beeswax and plant cutin) Carotenoids (pigments found in plants and animals) 3.4.1 Saturated and Unsaturated Fats Before we dive into the different concepts related to lipids, it would be best to understand the concept of saturation and unsaturation. When all of the carbon-carbon bonds in the fatty acid chain are single bonds, the fatty acid is said to be ”saturated.” Saturated fatty acids have the maximum number of hydrogen atoms attached to each carbon in the chain and are therefore solid at room temper- EnterMedSchool.com 65 Alpha Version ature. Examples of saturated fatty acids include palmitic acid and stearic acid, which are found in animal fats. In contrast, when one or more carbon-carbon double bonds are present in the fatty acid chain, the fatty acid is said to be ”unsaturated.” Unsaturated fatty acids have fewer hydrogen atoms attached to each carbon in the chain and are therefore liquid at room temperature. Examples of unsaturated fatty acids include oleic acid and linoleic acid, which are found in vegetable oils. The degree of saturation in lipids affects their melting point, which is the temperature at which they change from a solid to a liquid. Saturated lipids have higher melting points than unsaturated lipids, because the strong interactions between the saturated fatty acid chains make it more difficult for the molecules to move and become liquid. In contrast, the presence of double bonds in unsaturated fatty acids creates a kink in the fatty acid chain, which prevents the molecules from packing closely together and lowers the melting point of the lipid. This is why unsaturated fats are usually liquid at room temperature, while saturated fats are solid. To understand the concept better, imagine a group of people stand- ing in a crowded room. The people are like the fatty acid molecules in a triglyceride molecule, and the room is like the triglyceride molecule. If the people are tightly packed together, it will be harder for them to move and break free from each other. This is like a triglyceride molecule with saturated fatty acids, which have a higher melting point and boiling point because the fatty acid molecules are more tightly packed together and have fewer double bonds. On the other hand, if the people are not as tightly packed together and there is more space between them, it will be easier for them EnterMedSchool.com 66 Alpha Version to move and break free from each other. This is like a triglyceride molecule with unsaturated fatty acids, which have a lower melting point and boiling point because the fatty acid molecules are not as tightly packed together and have more double bonds. Lipids can be classified as saturated, monounsaturated, or polyun- saturated depending on the number of double bonds in the fatty acid chains. Saturated lipids contain only saturated fatty acids, while unsaturated lipids contain one or more unsaturated fatty acids. Mo- nounsaturated lipids have one double bond in the fatty acid chain, while polyunsaturated lipids have two or more double bonds. The degree of saturation in lipids is important for their function in the body. Saturated fats, for example, are typically solid at room temperature and tend to be associated with an increased risk of heart disease. In contrast, unsaturated fats are usually liquid at room temperature and are associated with a reduced risk of heart disease. The degree of saturation also affects the fluidity of the cell membrane, as lipids with more double bonds in their fatty acid chains are more flexible and fluid than lipids with fewer double bonds. 3.4.2 Triglycerides Triglycerides, also known as triacylglycerols or neutral fats, are a type of lipid that plays an important role in the body. Triglycerides are called triglycerides because they are composed of three fatty acid molecules bonded to a single glycerol molecule. The prefix ”tri-” means three, and ”glyceride” refers to the glycerol molecule. The fatty acids are attached to the glycerol molecule by ester bonds, creating a molecule that has three fatty acid chains hanging off it. Triglycerides can be either saturated or unsaturated, and the degree EnterMedSchool.com 67 Alpha Version Figure 20: Saturated fatty acids have hydrocarbon chains connected by single bonds only. Unsaturated fatty acids have one or more dou- ble bonds. Each double bond may be in a cis or trans configuration. In the cis configuration, both hydrogens are on the same side of the hydrocarbon chain. In the trans configuration, the hydrogens are on opposite sides. A cis double bond causes a kink in the chain. (Credit: openstax.org) EnterMedSchool.com 68 Alpha Version of saturation affects their physical properties. Triglycerides are the main form of fat stored in the body, and they are also found in foods such as vegetable oils, butter, and lard. In the body, triglycerides serve as an important source of energy, and they are broken down into their component parts and used by cells for energy production. The glycerol molecule is converted to glucose, which can be used by cells for energy, while the fatty acid molecules are broken down into smaller molecules called ketone bodies, which can also be used for energy. Excessive consumption of triglycerides can lead to obesity and other health problems, such as heart disease and diabetes. However, triglycerides also play a role in the structure and function of cell membranes. They are one of the main components of the phospho- lipid bilayer, which forms the basic structure of the cell membrane. The fatty acid chains in the triglycerides help to make the mem- brane more fluid and flexible, allowing the cell to carry out its many functions. Triglycerides can be found in various parts of the human body, such as adipose tissue, which is the tissue that stores fat. Adipose tissue is found throughout the body, but it is especially concentrated in areas such as the abdomen, hips, and thighs. Triglycerides are also present in the bloodstream and can be measured using a blood test. High levels of triglycerides in the blood can be an indication of vari- ous health problems, such as metabolic disorders or cardiovascular disease. In summary, triglycerides are an essential component of the hu- man body, serving as an important source of energy and playing a role in the structure and function of cell membranes. They can be found in various parts of the body, including adipose tissue and the EnterMedSchool.com 69 Alpha Version bloodstream, and are present in many common foods. Figure 21: Triacylglycerol is formed by the joining of three fatty acids to a glycerol backbone in a dehydration reaction. Three molecules of water are released in the process. (Credit: openstax.org) EnterMedSchool.com 70 Alpha Version 3.4.3 Phospholipids Phospholipids are a type of lipid that is essential for the structure and function of cell membranes. They are composed of a glycerol backbone covalently bonded to two fatty acid chains and a phos- phate group attached to the glycerol backbone. The phosphate group is negatively charged, which gives phospholipids their unique properties. The importance of phospholipids in the human body cannot be overstated. They are the main building blocks of cell membranes and help to regulate what goes in and out of cells. In addition, phos- pholipids also play a role in cell signaling and are involved in various biological processes such as blood clotting and inflammation. Phospholipids are found in many different parts of the body, in- cluding cell membranes, nerve tissue, and the brain. They are also present in foods such as egg yolks and soybeans. Deficiencies in phospholipids have been linked to various health conditions, such as neurological disorders and liver disease. Therefore, ensuring an adequate intake of phospholipids is important for overall health and well-being.. As mentioned earlier, the general structure of a phospholipid con- sists of a glycerol molecule with two fatty acid chains and a phos- phate group attached to the third carbon of the glycerol. The phos- phate group is negatively charged, which gives phospholipids their unique properties. The fatty acid chains are long, nonpolar hydrocarbon chains that are hydrophobic (water-fearing). They consist of a carboxylic acid group (-COOH) at one end and a methyl group (-CH3) at the other end. The fatty acid chains vary in length and degree of saturation, EnterMedSchool.com 71 Alpha Version Figure 22: A phospholipid is a molecule with two fatty acids and a modified phosphate group attached to a glycerol backbone. The phosphate may be modified by the addition of charged or polar chemical groups. (Credit: openstax.org) EnterMedSchool.com 72 Alpha Version which affects the fluidity and permeability of the cell membrane. In contrast, the phosphate group and the glycerol molecule are hydrophilic (water-loving) due to their polar nature. The phosphate group contains a negatively charged phosphate ion (-PO4) and is of- ten attached to another polar or charged molecule, such as choline or serine, forming a phosphatidylcholine or phosphatidylserine, re- spectively. This polar head group faces outward and interacts with the aqueous environment, while the hydrophobic fatty acid tails face inward, away from the water. The combination of the hydrophilic head group and hydrophobic tails makes phospholipids amphipathic, meaning they have both hy- drophilic and hydrophobic properties. This unique property allows phospholipids to spontaneously form bilayers, with the hydropho- bic tails facing inward and the hydrophilic heads facing outward. The phospholipid bilayer forms the basis of all cell membranes and organelles, providing a barrier between the inside and outside of the cell while allowing for selective transport of molecules.. The phospholipid bilayer serves several functions, including acting as a barrier to prevent unwanted molecules from entering or exiting the cell, as well as facilitating the transport of specific molecules across the membrane. It also plays a role in cell signaling and cell recognition. The bilayer’s fluid nature allows it to be flexible, allow- ing the cell to change shape, and its selective permeability allows specific molecules to pass through. This selective permeability is essential for regulating the transport of ions and molecules into and out of the cell, which is crucial for maintaining the cell’s internal environment. In addition to phospholipids, the bilayer also contains other lipids, such as cholesterol, and proteins, which are embedded in the mem- EnterMedSchool.com 73 Alpha Version Figure 23: The phospholipid bilayer is the major component of all cel- lular membranes. The hydrophilic head groups of the phospholipids face the aqueous solution. The hydrophobic tails are sequestered in the middle of the bilayer. (Credit: openstax.org) EnterMedSchool.com 74 Alpha Version brane. The proteins can act as transport channels, enzymes, or receptors, among other functions. The lipid composition of the bi- layer varies among different cell types and organelles, and the ratio of different lipid species can affect membrane fluidity and stability. For example, the presence of cholesterol in the bilayer can affect the fluidity of the membrane, making it more or less permeable to different molecules. Phospholipid bilayers are found in various parts of the human body, as mentioned earlier, and their functions vary depending on the cell type and location. In the plasma membrane of cells, the bilayer acts as a barrier to protect the cell and regulate the transport of molecules. In the myelin sheath, the bilayer acts as an insulating layer that allows for the rapid conduction of nerve impulses. Overall, the phospholipid bilayer is a critical component of all cell membranes, serving various functions that are essential for the survival of the cell and the organism as a whole. Lipid bilayers can form vesicles through a process called self-assembly. When lipids are placed in an aqueous solution, they will sponta- neously arrange themselves into a bilayer structure. This is because the hydrophobic tails of the lipids are attracted to one another, while the hydrophilic heads interact with the surrounding water molecules. The resulting structure is a stable, dynamic membrane that can enclose a small volume of water or other solution. The phospholipid bilayer acts like a wall in that it separates the inside of the cell from the outside environment, much like a wall separates the inside of a house from the outside. The proteins and structures on the surface of the phospholipid bilayer can be thought of as plants growing on the wall’s surface. The roots of the plants are anchored in the hydrophobic interior of the wall, while the plants EnterMedSchool.com 75 Alpha Version Figure 24: Fats are molecules that have both hydrophobic and hy- drophilic properties, meaning they are amphiphilic. The long hy- drocarbon tail of a fat molecule repels water, while the glycerol part of the molecule is attracted to water. When fats are in water, they form a structure called a micelle, where the hydrophilic heads of the fat molecules are on the outer surface and the hydrophobic tails are on the inside. This arrangement protects the hydrophobic tails from the water and allows the hydrophilic heads to interact with the surrounding water molecules. (Credit: openstax.org) themselves are on the outside, exposed to the environment. These proteins and structures perform various functions, such as transport- ing molecules across the membrane, communicating with other cells, and interacting with the environment. Sometimes these plants don’t have roots, and they just climb and hold to the wall itself, while many others are anchored in the wall and sometimes the roots even go all the way to the other side! EnterMedSchool.com 76 Alpha Version 3.4.4 Sterols Sterols are a unique class of lipids that play important structural and biological roles in cells. They have a fused ring structure composed of four rings, three of which are six-membered and one of which is a five-membered ring. The structure of the fused rings is cru- cial to their function in cell membranes, where they help regulate membrane fluidity and permeability. Cholesterol, the most well-known sterol, is an essential component of the human body and is found in animal cells. Its structure is similar to other sterols, with a fused ring structure and a hydrocarbon tail. The hydroxyl (-OH) group on the steroid ring makes cholesterol amphipathic, meaning it has both hydrophilic and hydrophobic regions. The unique structure of cholesterol allows it to interact with the hydrophobic tails of phospholipids in cell membranes, playing an important role in maintaining membrane fluidity and rigidity. In addition to its structural role, cholesterol is also a precursor for the synthesis of steroid hormones and bile acids. Cholesterol is synthesized in the liver and is also obtained from the diet. It plays a number of important roles in the body, includ- ing serving as a precursor for the synthesis of hormones, such as testosterone and estrogen, and bile acids as mentioned, which are necessary for the digestion and absorption of fats. Other important sterols include ergosterol, which is found in fungi and plant cells, and stigmasterol, which is found in plants. These sterols have different biological functions, but they share a similar chemical structure to cholesterol. Sterols have many important functions in the body. They are in- EnterMedSchool.com 77 Alpha Version volved in the synthesis of hormones and other biomolecules, they help to regulate cell membrane fluidity and structure, and they play a role in the absorption and metabolism of fats. They are also important for maintaining the overall health of cells and tissues. 3.4.5 Waxes Waxes are complex lipids that have unique physical and chemical properties due to their intricate structure. Their high molecular weight and hydrophobic nature make them water-resistant, which is important for their function in waterproofing and protection. Waxes are composed of a long-chain fatty acid and a long-chain alcohol that are esterified through an ester bond. The length and degree of saturation of these chains can vary depending on the organism and the specific function of the wax. For example, plant waxes often contain very long-chain fatty acids and alcohols, which contribute to their water-repelling properties. In contrast, animal waxes such as beeswax contain shorter-chain fatty acids and alcohols. Waxes can be found in a variety of biological structures such as the cuticle of plants, the feathers of birds, and the exoskeletons of insects. One of the most important functions of waxes is to provide a pro- tective barrier for the surface of an organism. In plants, waxes are found on the surface of leaves, stems, and other plant organs, where they help to reduce water loss and protect against insect damage and fungal infections. In animals, waxes are found on the surface of the skin and in the ear canal, where they help to keep the skin and ears moist and prevent them from becoming dry and irritated. Waxes also play a role in the regulation of body temperature in animals. In birds and mammals, waxes are found in the feathers and fur, respectively, and they help to insulate the body and maintain EnterMedSchool.com 78 Alpha Version a constant body temperature. In insects, waxes are found on the surface of the exoskeleton, where they help to prevent desiccation and regulate body temperature. In addition to their protective and regulatory functions, waxes also have a number of other biological roles. For example, some waxes are used as signaling molecules, and others are involved in the synthesis of hormones and other biomolecules. Overall, waxes are important for the function and survival of a wide range of organisms. 3.5 Nucleic Acids Nucleic acids are some of the most important biomolecules in living organisms. They play a critical role in the transmission and expres- sion of genetic information, allowing cells to pass on their genetic instructions to the next generation of cells. This genetic informa- tion not only determines an organism’s physical traits, but also the functions and behaviors of its cells. In addition to their role in ge- netic transmission, nucleic acids are also involved in many other cellular processes, including DNA repair, RNA synthesis, and protein synthesis. Deoxyribonucleic acid (DNA) and ribonucleic acid (RNA) are the two main types of nucleic acids. DNA is found primarily in the nucleus of cells, and it contains the genetic information that is passed down from one generation to the next. RNA, on the other hand, is synthe- sized from DNA and plays a crucial role in the production of proteins, which are essential for the structure and function of cells. The study of nucleic acids has revolutionized our understanding of genetics and has led to numerous discoveries in medicine and biotechnology. In the cell, DNA can be found in the nucleus and in the organelles EnterMedSchool.com 79 Alpha Version (chloroplasts, and mitochondria) of eukaryotes. In prokaryotic cells, the DNA is free, it is not enclosed in a membranous envelope (can be found most commonly as singular bacterial chromosomes or as plasmids). 3.5.1 Purines and Pyrimidines Purines and pyrimidines are two types of nitrogenous bases that are found in nucleotides, the building blocks of nucleic acids such as DNA and RNA. Purines are larger molecules than pyrimidines and consist of a six-membered ring fused to a five-membered ring. The purine bases that are found in nucleic acids are adenine (A) and guanine (G). Pyrimidines, on the other hand, are smaller molecules and consist of a single six-membered ring. The pyrimidine bases that are found in nucleic acids are cytosine (C), thymine (T), and uracil (U). In DNA, thymine is present instead of uracil. The difference in size between purines and pyrimidines has impor- tant implications for the structure of DNA and RNA. Because purines are larger than pyrimidines, they pair more easily with pyrimidines, which helps to maintain the structural stability of DNA and RNA. In DNA, A always pairs with T, and G always pairs with C, while in RNA, A pairs with U, and G pairs with C. This base pairing is crucial for the proper functioning of DNA and RNA as genetic information carriers. Understanding the differences between purines and pyrimidines is essential for understanding the molecular basis of genetics and the processes that underlie the transmission of genetic information from one generation to the next. EnterMedSchool.com 80 Alpha Version 3.5.2 DNA and RNA DNA, or deoxyribonucleic acid, is the genetic material that carries the hereditary information in all living organisms. It is a double- stranded molecule that consists of a sugar-phosphate backbone and four nitrogenous bases: adenine (A), cytosine (C), guanine (G), and thymine (T). These four bases pair up with each other through hydrogen bonds: A pairs with T, and C pairs with G. The sequence of these bases carries the genetic information that is passed down from one generation to the next. DNA is wrapped around proteins called histones to form chromatin. The chromatin can be further compacted into chromosomes during cell division. When the DNA is tightly packed, it is called heterochro- matin, and when it is more open and accessible, it is called euchro- matin. Heterochromatin is usually not actively transcribed, while euchromatin is more accessible to enzymes involved in transcription and is usually actively transcribed. The information encoded in DNA is used to synthesize proteins and other molecules that are essential for life processes. The process of transcription involves the conversion of the DNA code into RNA, and translation involves the conversion of RNA into proteins. These processes are tightly regulated to ensure the correct expression of genes and the production of the necessary molecules at the appropriate times and in the appropriate amounts. DNA replication, or the process of copying the DNA, is also essential for cell division and the transmission of genetic information to daughter cells.. RNA, or ribonucleic acid, is a single-stranded molecule made up of a ribose sugar-phosphate backbone and four nitrogenous bases: adenine (A), cytosine (C), guanine (G), and uracil (U). The nitroge- nous bases in RNA are the same as those in DNA, except that uracil EnterMedSchool.com 81 Alpha Version replaces thymine in RNA. RNA plays a central role in the transfer of genetic information from DNA to the ribosomes, where proteins are synthesized. This process is known as the central dogma of molecular biology. In the first step of the central dogma, DNA is transcribed into RNA by an enzyme called RNA polymerase. The RNA molecule then carries the genetic information from the nucleus to the ribosomes, where it serves as a template for the synthesis of proteins in a process called translation. There are four main types of RNA: messenger RNA (mRNA), ribo- somal RNA (rRNA), transfer RNA (tRNA), and microRNA (miRNA). mRNA carries genetic information from DNA to ribosomes, which are responsible for synthesizing proteins in a cell. When a cell re- quires a particular protein to be made, the gene that codes for it is ”turned on” and mRNA is synthesized in the nucleus. The base se- quence of mRNA is similar to the coding sequence of the DNA it was transcribed from, except that the RNA contains uracil (U) instead of thymine (T). In the cytoplasm, mRNA interacts with ribosomes and other cellular machinery to translate the genetic code into a protein. rRNA, which is a component of ribosomes, helps to catalyze the chemical reactions involved in protein synthesis. tRNA molecules bring amino acids to the ribosome, where they are assembled into a protein chain. Finally, miRNA is a small, non-coding RNA molecule that can regu- late gene expression by binding to mRNA and preventing its trans- lation into protein. In addition to its role in protein synthesis, RNA also plays a catalytic role in certain chemical reactions. These specialized RNA molecules, known as ribozymes, have been shown to catalyze reactions such as peptide bond formation and RNA splicing. Overall, RNA is a versatile EnterMedSchool.com 82 Alpha Version and essential molecule in the functioning of cells and the expression of genetic information. DNA and RNA are nucleic acids composed of nucleotides that differ in their sugar component. The sugar in DNA is deoxyribose, while in RNA, it is ribose. The primary difference between these sugars is the presence (or absence) of a hydroxyl group on the second carbon atom. The carbon atoms of the sugar molecule are designated as 1′, 2′, 3′, 4′, and 5′, with 1′ being read as “one prime”. Each nucleotide contains a phosphate residue connected to the hydroxyl group of the 5′ carbon of one sugar and the hydroxyl group of the 3′ carbon of the next sugar in the nucleotide sequence. This linkage is called a 5′-3′ phosphodiester linkage, and its formation involves the removal of two phosphate groups. It is not formed by simple dehydration reactions like other linkages in macromolecules mentioned earlier. A polynucleotide is a chain of nucleotides linked together by phos- phodiester bonds. DNA and RNA are polynucleotides that contain many thousands of these phosphodiester linkages. The sequence of the nitrogenous bases in DNA and RNA carries genetic information that encodes the instructions for the synthesis of proteins and other molecules. The central dogma of molecular biology describes the flow of genetic information from DNA to RNA to protein. EnterMedSchool.com 83 Alpha Version Figure 25: Steroids such as cholesterol and cortisol are composed of four fused hydrocarbon rings. (Credit: openstax.org) EnterMedSchool.com 84 Alpha Version Figure 26: A ribosome has two parts: a large subunit and a small sub- unit. The mRNA sits in between the two subunits. A tRNA molecule recognizes a codon on the mRNA, binds to it by complementary base pairing, and adds the correct amino acid to the growing pep- tide chain. (Credit: openstax.org) EnterMedSchool.com 85 Alpha Version Figure 27: In a double stranded DNA molecule, the two strands run antiparallel to one another so that one strand runs 5′ to 3′ and the other 3′ to 5′. The phosphate backbone is located on the outside, and the bases are in the middle. Adenine forms hydrogen bonds (or base pairs) with thymine, and guanine base pairs with cytosine. (Credit: openstax.org) EnterMedSchool.com 86 Alpha Version Figure 28: Native DNA is an antiparallel double helix. The phosphate backbone (indicated by the curvy lines) is on the outside, and the bases are on the inside. Each base from one strand interacts via hydrogen bonding with a base from the opposing strand. (credit: Jerome Walker/Dennis Myts) EnterMedSchool.com 87 Alpha Version Figure 29: A nucleotide is made up of three components: a nitroge- nous base, a pentose sugar, and one or more phosphate groups. Carbon residues in the pentose are numbered 1′ through 5′ (the prime distinguishes these residues from those in the base, which are numbered without using a prime notation). The base is attached to the 1′ position of the ribose, and the phosphate is attached to the 5′ position. When a polynucleotide is formed, the 5′ phosphate of the incoming nucleotide attaches to the 3′ hydroxyl group at the end of the growing chain. Two types of pentose are found in nu- cleotides, deoxyribose (found in DNA) and ribose (found in RNA). Deoxyribose is similar in structure to ribose, but it has an H instead of an OH at the 2′ position. Bases can be divided into two categories: purines and pyrimidines. Purines have a double ring structure, and pyrimidines have a single ring.. (Credit: openstax.org) EnterMedSchool.com 88 Alpha Version