Materials Engineering Lecture Notes PDF
Document Details
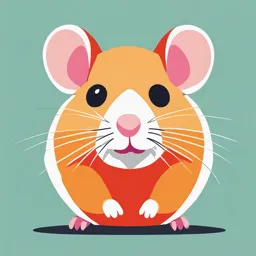
Uploaded by GlamorousVirginiaBeach
AKTU
Tags
Summary
This document provides an overview of imperfections in solids, specifically crystal defects. It discusses different types of crystal defects, including point defects like vacancies and interstitials, as well as substitutional defects and other imperfections such as grain boundaries. The notes cover how these defects influence the mechanical, electrical, and optical properties of solids.
Full Transcript
Materials Engineering Unit I Topics Covered Lecture Notes 2 Crystal Imperfection Imperfections in Solids or Crystal defects: Irregularity in the arrangement of constituent particles in solids is called...
Materials Engineering Unit I Topics Covered Lecture Notes 2 Crystal Imperfection Imperfections in Solids or Crystal defects: Irregularity in the arrangement of constituent particles in solids is called crystal defect or imperfection in solids. Crystal defect is imperfection in the regular geometrical arrangement of the atoms in a crystalline solid. These imperfections result from deformation of the solid, rapid cooling from high temperature, or high-energy radiation (X-rays or neutrons) striking the solid. Located at single points, along lines, or on whole surfaces in the solid, these defects influence its mechanical, electrical, and optical behaviour. Can be divided according to their geometry and shape 0-D or point defects 1-D or line defects (dislocations) 2-D (external surface) 3-D (grain boundaries, crystal twins, twists, stacking faults, voids and precipitates) 0-D or Point Defects: A. Vacancy Defects B. Interstitial Defects C. Substitutional Defects D. Schottky Defects E. Frenkel Defects (a)Vacancy Defects: When some lattice sites left vacant while the formation of crystal, the defect is called Vacancy Defects. In vacancy defects, an atom is missing from its regular atomic site. Because of missing of atom the density of substance decreases, i.e. because of vacancy defects. The vacancy defect develops on heating of substance. (b)Interstitial Defects: Sometime in the formation of lattice structure some of the atoms occupy interstitial site, the defect arising because of this is called Interstitial Defects. In interstitial defect, some atoms occupy sites at which; generally there is no atom in the crystal structure. An interstitial defect occurs when an atom takes the interstitial position of the lattice structure. This interstitial atom may be of the same crystal or of a foreign material. Accordingly, interstitial defect can be of two types: Self-Interstitial Defect—occurs when atom of the same crystalline solid occupies the interstitial position leaving its original lattice site. Interstitial Defect—occurs when a foreign atom occupies the interstitial position. Although extra atom occupies the empty interstitial space, the size of the atom is usually larger than that of the empty space. Thus the surrounding atoms are compressed and distorted. Presence of substantial number of interstitial atoms can change the mechanical and thermal properties of the solid. However, this is sometime beneficial, and thus interstitial defects can be applied in a controlled way to enhance various properties of the solid. For example, in steel production, carbon is added with iron. Because of the interstitial defects, the number of atoms becomes larger than the number of lattice sites. Increase in number of atoms increases the density of substance, i.e. interstitial defects increase the density of substance. (c) Substitutional Defect: Substitutional Defect occurs when the original atom in the lattice site of a crystalline solid is replaced by a different type of atom (i.e. foreign atom). Unlike interstitial defect, foreign atom should occupy the lattice site only and not the interstitial position. The foreign atom may be of same size or different (either larger or smaller). Depending on the size of the substituted foreign atom, the neighboring atoms may remain either in tension or in compression. Substitutional Atom is a Point Defect. Here a foreign atom replaces the original atom and occupies the lattice site in the crystal solid. Where Substitutional defects can be found? Substitutional defects can be found in brass, where zinc atoms replace copper atoms. In semiconductor materials also. Causes of Substitutional defect in solids: Presence of foreign atoms as natural impurities within the solid. Deliberate addition, such as during iron to steel conversion, heat treatment, sputtering, etc. Diffusion, caused by close contact between two different materials. Effects of Substitutional defects in solids: If the foreign atom is smaller in size as compared to the original atom of the solid, the neighbouring atoms will remain in tensile stress. If the foreign atom is larger in size as compared to the original atom of the solid, the neighbouring atoms will remain in compressive stress. So, presence of foreign atom may distort the original lattice structure. Presence of substantial number of foreign atoms can change the mechanical and thermal properties of the solid. However, this is sometime beneficial, and thus substitutional defects can be applied in a controlled way to enhance various properties of the solid. (d) Schottky Defect: It is one type of Point Defect that occurs in ionic crystals. Schottky defect occurs when oppositely charged atoms (cation and anion) leave their corresponding lattice sites and create a pair of Vacancy Defects. Since both cation and anion leave the lattice sites at the same time, so overall electrical neutrality of the crystal is maintained; however, density reduces because of the vacancies. Example of materials where Schottky defect can be found: Sodium Chloride (NaCl) Potassium Chloride (KCl) Potassium Bromide (KBr) Silver Bromide (AgBr) Cerium Dioxide (CeO2) Thorium Dioxide (ThO2) Features of Schottky Defect: Schottky defects occur in ionic crystals where the size of anion is almost same with the size of the cation. One anion and one cation leave the crystal at the same time to create Schottky defect. None of them occupies the interstitial site again. One Schottky defect leads to the formation of two vacancies. Electrical neutrality of the whole crystal is maintained. Density of the crystal decreases for Schottky defects as vacancies are created. (e) Frenkel Defect: Frenkel Defect is one type of Point Defect; in fact, it is a combination of both Vacancy and Interstitial type of point defects. Usually, this type of defect is observed in ionic solids, where size of anion is substantially larger than the size of cation. Basically, a Frenkel Defect is one type of point defect where an atom (better to say ion, especially cation) leaves its original lattice site and occupies an interstitial position on the same crystal. When an atom leaves the original lattice site then Vacancy Defect is created. Alternatively, when an atom (of same crystal or foreign crystal) occupies the interstitial site then Interstitial Defect is created. Since, in Frenkel Defect, one atom leaves the original lattice site and occupies an interstitial site in the same crystal, so Frenkel Defect is a pair of Vacancy & Self-interstitial Defects. Example of Materials Where Frenkel Defect can be Found: Zinc Sulfide (ZnS) Silver Chloride (AgCl) Silver Bromide (AgBr) Features of Frenkel Defect: In Frenkel Defect, neither any atom leaves the solid crystal nor do any foreign atoms occupy any interstitial position. So the overall density of the crystal solid remains unchanged. This type of defect is usually observed in ionic crystals, where size of anion is substantially larger than the size of cation. For example: zinc sulphide (ZnS), silver chloride (AgCl), etc. The smaller ions, usually cation, move from original lattice site to interstitial position; while, the larger ions (anion) remain in their corresponding lattice sites. It does not affect the chemical property of the solid. Electrical neutrality is also maintained in this defect. Difference between Frenkel Defect and Schottky Defect: 1. Although both—Schottky and Frenkel defects occur in ionic materials, Frenkel defect occurs if size of anion is quite large as compared to that of the cation; whereas, Schottky defect occurs if difference in size between cation and anion is small. 2. In Frenkel defect, only the smaller ion (cation) leaves its original lattice site; whereas, the anion remains in corresponding lattice sites. However, in Schottky defect, both cation and anion leave the solid crystal. 3. Unlike Frenkel defect where one atom shifts from original lattice site to the interstitial position, in Schottky defect two atoms leave the solid crystal. So one vacancy and one self-interstitial occur in Frenkel defect; whereas, two vacancies occur in Schottky defect. 4. The number of atoms present in the crystal before and after Frenkel defect remains same. However, one Schottky defect leads to the reduction of two atoms from the crystal. 5. Density of the solid crystal before and after Frenkel defect remains same as no atom leaves the solid. However, Schottky defect reduces density of the solid. LINE DEFECTS Line imperfections, or dislocations, in crystalline solids are defects that produce lattice distortions centred about a line. A dislocation is simply the edge of an extra inserted fractional plane of atoms. Normally the symbol ︎⏊︎ (inverted T) is used to represent a positive dislocation (extra fractional plane) and ︎T is used to represent a negative dislocation (missing fractional plane). Schematic presentation of a dislocation; the last row of atoms (dark) in the inserted fractional plane An edge dislocation is a defect where an extra half-plane of atoms is introduced mid way through the crystal, distorting nearby planes of atoms. When enough force is applied from one side of the crystal structure, this extra plane passes through planes of atoms breaking and joining bonds with them until it reaches the grain boundary. A schematic example of an edge dislocation is shown below : Model of a simple cubic lattice and representation of an edge dislocation The arrangement of atoms around an edge dislocation can be simulated by the following sequence of operations. Suppose that all the bonds across the surface ABCD are broken and the faces of the crystal are separated so that an extra half-plane of atoms can be inserted in the slot, as illustrated in above figure. The faces of the slot will be displaced by one atom spacing, but the only large disturbance of the atoms from their normal position relative to their neighbours is close to the line DC. The deflection and distortion of the interatomic bonds decrease with increasing distance from the line. This line DC is called a positive edge dislocation. A negative edge dislocation would be obtained by inserting the extra plane of atoms below plane ABCD. BURGUERS VECTOR AND BURGERS CIRCUIT The most useful definition of a dislocation is given in terms of the Burgers circuit. A burgers circuit is any atom-to-atom path taken in a crystal containing dislocations which forms a closed loop. Such a path, MNOPQ, is illustrated in the following figure: fig. A: Burger circuit round an edge dislocation fig. B: The circuit in a perfect crystal If the same atom-to-atom sequence is made in a dislocation-free crystal, as in figure B, and the circuit does not close, then the first circuit, the one showed in figure A, must enclose one or more dislocations. The vector required to complete the circuit is called the Burgers vector. The Burgers vector of an edge dislocation is normal to the line of the dislocation. The Burgers vector of a screw dislocation is parallel to the line of the dislocation. In the most general case the dislocation line lies at an arbitrary angle to its Burgers vector and the dislocation line has a mixed edge and screw character. However, the Burgers vector of a single dislocation has fixed length and direction, and is independent of the position and orientation of the dislocation line. Screw Dislocation: A screw dislocation can be visualized by cutting a crystal along a plane and slipping one half across the other by a lattice vector, the halves fitting back together without leaving a defect. If the cut only goes part way through the crystal, and then slipped, the boundary of the cut is a screw dislocation. It comprises a structure in which a helical path is traced around the linear defect (dislocation line) by the atomic planes in the crystal lattice. In pure screw dislocations, the Burgers vector is parallel to the line direction. Screw dislocations can be produced by a tearing of the crystal parallel to the slip direction. If a screw dislocation is followed all the way around a complete circuit, it would show a slip pattern similar to that of a screw thread. A screw dislocation is much harder to visualize. Imagine cutting a crystal along a plane and slipping one half across the other by a lattice vector, the halves fitting back together without leaving a defect. The motion of a screw dislocation is also a result of shear stress, but the defect line movement is perpendicular to direction of the stress and the atom displacement, rather than parallel. Difference / Comparison between Edge Dislocation and Screw Dislocation: Edge Dislocation Screw Dislocation 1. E d g e d i s l o c a t i o n o c c u r s d u e t o t h e 1. Screw dislocation provides easy crystal introduction or elimination of an extra row of growth because of additional unit cells and atoms. atoms can be added to the screw. 2. Shear, Tensile and Compressive stress fields 2. Only Shear stress fields are present in the may be present in the Edge dislocation. Screw dislocation. 3. In this dislocation, Burger’s vectors always 3. In this dislocation, Burger’s vectors always perpendicular to the dislocation line. parallel to the dislocation line. 4. Region of lattice disturbance extends along an 4. Region of lattice disturbance is in two edge inside a crystal. separate planes and cross each other at right angles. 5. This dislocation occurs due to climb and glide 5. This dislocation occurs only due to glide motion. motion. 6. There are two types of Edge dislocation, 6. Screw dislocation does not show any type like positive and negative. edge dislocation. Surface / Interfacial defects: Interfacial defects can be defined as boundaries that have two dimensional imperfections in crystalline solids, and have different crystal structures and/or crystallographic orientations on either side of them. They refer to the regions of distortions that lie about a surface having thickness of a few atomic diameters. For example: external surfaces, grain boundaries, twin boundaries, stacking faults, etc. These defects arise from the clustering of line defects into a plane. External surface: The environment of an atom at a surface differs from that of an atom in the bulk; especially the number of neighbours (coordination) at surface is less. Surface atoms are not bonded to the maximum number of nearest neighbours, and are therefore in a higher energy state than the atoms at interior positions. The bonds of these surface atoms that are not satisfied give rise to a surface energy. To reduce the energy, materials tend to minimize, if possible, the total surface area. This result in relaxation (the lattice spacing is decreased) or reconstruction (the crystal structure changes). Grain boundaries: Crystalline solids are, usually, made of number of grains separated by grain boundaries. Grain boundaries are several atoms distances wide, and there is mismatch of orientation of grains on either side of the boundary as shown in figure. When this misalignment is slight, on the order of few degrees (< 10 ̊), it is called low angle grain boundary. These boundaries can be described in terms of aligned dislocation arrays. If the low grain boundary is formed by edge dislocations, it is called tilt boundary, and twist boundary if formed of screw dislocations. Both tilt and twist boundaries are planar surface imperfections in contrast to high angle grain boundaries. For high angle grain boundaries, degree of disorientation is of large range (> 15 ̊). Grain boundaries are chemically more reactive because of grain boundary energy. In spite of disordered orientation of atoms at grain boundaries, polycrystalline solids are still very strong as cohesive forces present within and across the boundary. : Twin boundaries: It is a special type of grain boundary across which there is specific mirror lattice symmetry. Twin boundaries occur in pairs such that the orientation change introduced by one boundary is restored by the other. The region between the pair of boundaries is called the twinned region. Twins which forms during the process of recrystallization are called annealing twins, whereas deformation twins form during plastic deformation. Twinning occurs on a definite crystallographic plane and in a specific direction, both of which depend on the crystal structure. Annealing twins are typically found in metals that have FCC crystal structure (and low stacking fault energy), while mechanical/deformation twins are observed in BCC and HCP metals. Annealing twins are usually broader and with straighter sides than mechanical twins. Twins do not extend beyond a grain boundary. Tilt Boundary: A pure tilt boundary causes a constant angle of tilt between lattice planes of the same type in adjacent regions of the crystal. It is composed of a regular array of edge dislocations of the same sign in the plane of the boundary. If the Burgers vector of the dislocations is, b, and their spacing is, D, then the angle of tilt is given by, b θ = D Stacking faults: They are faults in stacking sequence of atom planes. Stacking sequence in an FCC crystal is ABC ABC ABC..., and the sequence for HCP crystals is AB AB AB.... When there is disturbance in the stacking sequence, formation of stacking faults takes place. Two kinds of stacking faults in FCC crystals are: (a) ABC AC ABC...where CA CA represent thin HCP region which is nothing but stacking fault in FCC, (b) ABC ACB CABC is called extrinsic or twin stacking fault. Three layers ACB constitute the twin. Thus stacking faults in FCC crystal can also be considered as submicroscopic twins. This is why no microscopic twins appear in FCC crystals as formation of stacking faults. Phase Boundaries: Phase boundaries exist in multiphase materials, wherein a different phase exists on each side of the boundary; furthermore, each of the constituent phases has its own distinctive physical and/or chemical characteristics. Phase boundaries play an important role in determining the mechanical characteristics of some multiphase metal alloys. Bulk or Volume or 3-Dimensional defects: Volume defects as name suggests are defects in 3-dimensions. These include pores, cracks, foreign inclusions and other phases. These defects are normally introduced during processing and fabrication steps. All these defects are capable of acting as stress raisers, and thus deleterious to parent metal’s mechanical behaviour. However, in some cases foreign particles are added purposefully to strengthen the parent material. The procedure is called dispersion hardening where foreign particles act as obstacles to movement of dislocations, which facilitates plastic deformation. Pores are detrimental because they reduce effective load bearing area and act as stress concentration sites.