Nutritional Physiology Lecture Notes (PDF)
Document Details
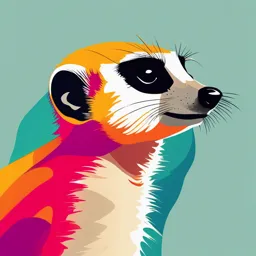
Uploaded by ProtectiveMoose7610
Wageningen University & Research
Tags
Summary
These lecture notes cover nutritional physiology, focusing on the balance between nutritional input and physiological output. The document details metabolic pathways, fluxes, and energetic efficiencies, along with the role of the microbiome and enzymes in digestion. It also describes the post-prandial and post-absorptive phases, and details the structure and function of the digestive system.
Full Transcript
Nutritional Physiology Lecture 1 (NP01): Mass Flow of Nutrients Nutritional physiology: Balance between nutritional input and physiological output. The metabolism balances this via metabolic pathways, fluxes (metabolic rate) or changes energetic efficiencies to generate either ATP or heat. Disbalan...
Nutritional Physiology Lecture 1 (NP01): Mass Flow of Nutrients Nutritional physiology: Balance between nutritional input and physiological output. The metabolism balances this via metabolic pathways, fluxes (metabolic rate) or changes energetic efficiencies to generate either ATP or heat. Disbalance might lead to differences in body composition. The body gets rid of products that it can’t use as faeces or urine. Most nutrients are used for energy and the rest product is CO2 that you breathe out, indigestible nutrients leave the body as faeces and are not absorbed. After you ingest food, you have no more influence on its fate, because your body will take care of it. Nutritional Input: total daily intake, meal size or pattern, nutrient composition. Physiological Output: physiological status, health status, environment. Metabolic rate. Microbial fermentation: undigested parts can be fermented by your microbiome and might provide energy for you body, but will never produce amino acids. All the amino acids have to come from your food. Enzymes: lower the activation energy, to facilitate cleaving of nutrients. You need a lot less heat/energy. Many enzymes are used in our GI tract digestion. The digestive system does not digest itself because enzyme activity is restricted to presence of food, regulation, pro-enzymes (zymogens), non-digestible mucus wall coating and a high replacement rate of mucosal cells. Anabolism: synthesis of body constituents. Synthesis of body protein. Catabolism: release of energy from food or body constituents. ATP and heat is produced. Post prandial phase (PPM): input > needs. Anabolic or catabolic disposal of the food. Digestion, absorption and storage of nutrients in liver, muscles and fats. DIT: Diet induced thermogenesis. PPM increases heat production (5-20%), dependent on ingested nutrients (proteins > carbohydrates > fat). The metabolizable energy on food products is not corrected for energetic costs of the DIT in the PPM. 1 Post absorptive phase: input < needs. Energy needs to be mobilized. Protein degradation, glycogen break down, fat breakdown. White adipose tissue provides glycerol and fatty acids. Proteins provide amino acids. A net catabolic situation, coming from internal organs. Homeostasis: ability of an organism to remain in balance. Counteracting factors that disturb vital functions, within the limits of the metabolic scope. Glucose concentration, blood pH, oxygen supply and body temperature (shivering and sweating). As long as you are in balance, there is no weight gain or loss. Depends on the intake, output and environment. Digestive systems: are similar between different species. It’s an outside world organ that is inside of your body. Used to transfer and digest nutrients from food. Undigested materials are excreted in the end and never enter the body. Integrated secretory behaviour and motor behaviour determine the digestion and absorption in the digestive tract. 1. Mouth: Digestion starts with chewing food. Lipase, amylase in mouth already start digestion. 2. Oesophagus: Transports to the stomach via sphincters. 3. Stomach: gastric acid, pepsin, gastric lipase, some digestion. Alcohol absorption. Has an enormous muscle layer. 4. Small intestine: pancreatic amylase, lipase, phospholipase, trypsin, chymotrypsin, elastin, dipeptidases, disaccharidases, absorption of monosaccharides, amino acids, fatty acids, glycerol, fats and water. Enhances full digestion of food to enhance absorption of nutrients. Segmentation and peristalsis help motility of the food bolus. Villi. a. Duodenum: 25 cm. The most digestion and absorption, CCK, secretin b. Jejunum: 2 meters c. Ileum: 3 meters Villi: absorptive enterocyte lining of the lumen of the small intestine, finger like projections. The villi are lined with a single layer of enterocytes, with mucus secreting goblet cells in between. Last part of digestion and absorption of nutrients. There are blood vessels and lymphatics in villi. Together, plicae (longitudinal foldings), villi and microvilli create a large complete absorptive area (250-300m2, 1 or 2 tennis fields). Microvilli: finger like foldings on the villi that enhance the surface area. This brush border contains digestive brush border enzymes, so the nutrients can be directly taken up Formula to calculate. 5. Colon: absorption of water and ions, bacterial fermentation, storage of waste. Absence of villi. a. Ascending b. Transverse c. Descending 2 Accessory digestion organs - Teeth: grinding of the food into smaller particles - Tongue: swallowing - Salivary glands: release enzymes into the mouth - Exocrine pancreas: produces enzymes into the lumen - Endocrine pancreas: produces hormones linked to metabolism - Liver: production of bile acids - Gall bladder: storage of bile acids. Releases its’ content into the duodenum for excretion. Gastrointestinal tract layers (lumen to outside) - Mucosa: secretion and absorption - Submucosa: vascular layer, support. - Muscularis: segmental contractions, circular/longitudinal layers. Important for movement of the food through the GI tract. Circular muscles contract constantly, mixing the contents and enhancing the absorption, pushing them forward. - Serosa: protective layer, outside of the small intestine. Gut retention: time between free amino acid in the lumen and appearing in the circulation. Also applies for fat. There is a delay in the uptake via the enterocytes, they can retain it. Communication: chemical and mechanical sensors send signals to the brain and spine, activate the enteric nervous system and change motility, secretion and blood flow. - Enteric Nervous System (ENS): senses interior of the GI tract, producing signals for movement etc. - Central Nervous System (CNS): muscular layers send signals to the nervous system, feedback and feedforward. - Endocrine: via the circulation, hormones travel to target cells. Gastrin. - Neurocrine: neurons sensing presence, muscle contractions. Neurotransmitters activating target cells. GRP, acetylcholine. - Paracrine: local communication, cell senses and produces molecules that affect the neighbouring cells. Segmentation: circular muscles segment the intestines by squeezing so the bolus moves forward. This can also segment the food and divide it into smaller parts. This happens 12-16x per minute. Circular muscles behind the bolus contract, while those in front of the bolus relax. Diffusion - Simple: passive diffusion - Facilitated: receptor-mediated. No ATP needed. - Active transport: ATP needed to pump the nutrient into the cell. This way it traps the nutrient into the cell. Migrating Motor Complex (MMC): 40 min rest, 40 min peristalsis, 10 min end of peristalsis. Flushes the content, prevents bacterial stasis. Cleaning of non-digestible parts. Digestion moves through the GI tract while organs sequentially respond. Transit time: 1-5 hours in stomach, 1.5 hours in the small intestine, 1-2 days in the large intestine. 3 Lecture 2 (NP02): Cephalic, Oral and Gastric phase (Meal anticipation) 1. Cephalic phase: phase when you see, hear, smell or think about food. Saliva production goes up. Dorsal vagal complex integrates all signals from the senses. There is no direct contact with the food yet, so no taste is generated yet. A meal that you don’t like almost won’t have any effect, a meal that you really like will give a very high response. Neurons are activated, stomach muscles relax and increase volume to get ready to receive food (motor). Pepsinogen enzymes are released and gastrin hormone is produced (secretory). The gallbladder is contracted. Pancreas enzymes are released into the duodenum by relaxation of the sphincter of Oddi. Gut receptors send signals to the hypothalamus, balancing food intake. Metabolic signals also send signals to the brain. Stomach pH goes down by producing stomach acids. a. Dorsal Vagal Complex (DVC): releases acetylcholine in the cephalic phase b. Acetylcholine: causes increase in stomach acid and pepsinogen release, which is activated to pepsin by stomach acids. c. Pepsin: digests proteins to oligopeptides, which give an enhancement signal via the gastrin releasing peptide (GRP), which releases gastrin into the circulation and stimulates the DVC again to release acetylcholine via the vagal efferent. d. Gastrin: causes more pepsinogen release and release of stomach acid. NPY: Ghrelin is released from the stomach and stimulates NPY neurons, which increase food intake and decrease energy expenditure. Positive energy balance. Inhibited by PYY, GLP, CCK. Ghrelin induces food intake, but all the other hormones decrease food intake. POMC: have the reversed effect of NPY, decreased food intake and increases energy expenditure. Negative energy balance. Leptin, from white adipocytes, activates POMC. 2. Oral phase: chewing on food, making food smaller and mixing saliva with the food. Saliva contains mucins (lubrication), amylase (starch), lipase (fats), lysozyme (antibacterial), IgA (Immune protection). Starches and amylase are mixed in the mouth, the amylase is protected in the middle of the starch, protecting the amylase from degradation by the low pH when in the stomach so at the end of the stomach half of the amylase is still present. Sphincters prevent passage in the oesophagus. Acetylcholine causes contraction, closing of the sphincters and NO and VIP cause relaxation, opening. Swallowing also causes more relaxation of the stomach. Upper oesophageal sphincter (UES): Normally closed to not swallow everything. Lower oesophageal sphincter (LES): is normally closed to inhibit backflow of gastric acids. Opens when food has to enter the stomach. 3. Gastric phase: the stomach has longitudinal and circular muscles, helping to mix and grind the food. Gastric distension triggers enhancement responses mediated by vago-vagal reflexes, releasing more stomach acid, gastrin and pepsinogen. The pH causes protein denaturation, making them accessible for enzymatic degradation. The lower the pH, the more optimal the activity of pepsin. The pH drops from 6 to 2 in about 1,5 hours. Suspends the bolus, some triglycerides are broken down to monoglycerides and free fatty acids. Some protein is broken down to peptides and amino acids, some starches become oligosaccharides. Water and acid are added, osmolality is increased. 4 Stomach parts LES and Cardia: prevention of reflux, entry of food, regulation of belching Fundus and body: reservoir, tonic force during emptying. Acid and enzyme secretion. Antrum and pylorus: mixing grinding and sieving. Contraction when food enters stomach Pyloric sphincter: the gatekeeper from the stomach to the duodenum. Gastric cells - Surface (Neck) cells: secrete mucus and bicarbonate, protect the wall of the stomach. - Parietal cells: Releases acid upon stimulation by acetylcholine, gastrin, or histamine. Plays a role in B12 digestion. - Chief cells: Releases pepsinogens and gastric lipase by activation by acetylcholine. Digestion. - G cells: release gastrin into the bloodstream by activation by GRP. - Endocrine cells: release hormones, gastrin, histamine and somatostatin. Regulate acid secretion. - ECL cells: produce histamine by acetylcholine and gastrin stimulation locally, only in the lamina propria. - D cells: Acids (low pH) stimulate D-cells to release somatostatin, inhibiting the G-cells paracrinally, so gastrin production goes down. This way acid release is inhibited and regulated. Pepsin: endopeptidase, cuts proteins at a specific locus, releasing oligopeptides. Oligopeptides then stimulate gastrin release, and acid and pepsinogen release. Triglyceride digestion: lipase digests triglycerides and free fatty acids stimulate CCK release. CCK causes gall bladder contraction, relaxation of the sphincter of Oddi, pancreatic enzymes are excreted in the small intestine. Vagotomy: cutting the nerves that activate stomach distension, to prevent stomach distension, so the pressure in the stomach builds up very quickly. Vitamin B12: comes into the stomach bound to salivary haptocorrin to protect degradation of vitamin B12. Haptocorrin is degraded into oligopeptides, the intrinsic factor then takes over protection of B12. Together they can bind to specific receptors in the ileum and B12 can be taken up by the body and is stored in the liver. When it is not protected by IF, it will be degraded and not taken up. Gastric motor responses: Gastric responses on a meal are receptive relaxation (enlargement of the volume), mixing and grinding (making the food bolus smaller and mix with secretions) and emptying (highly regulated). Digestive phase: Particles are sized down to 2 mm, the content is hyperosmotic and ca. 8.5 kJ/min releases from the stomach to the duodenum. The diet composition determines how fast it is emptied. Interdigestive phase: mixed motor complex, in between meals. Makes sure that the stomach is emptied fully. Indigestible particles stay in the stomach until it is emptied fully every 5 hours. Gastric emptying: larger particles can’t pass. The larger pieces at the antrum are grinded and flow back to the body of the stomach to be cut into smaller pieces before it can flow into the duodenum. Solid meals are digested much slower in the stomach. Hypertonic solutions are emptied slower than isotonic (low osmolarity) solutions. A higher concentration of glucose takes longer. Highly regulated. When nutrients reach the duodenum, it sends signals to the stomach to decrease tone in the proximal stomach, decrease contractions in the antrum and increase contractions in the pylorus. This makes sure that the duodenum has enough time to digest the nutrients that come in. 5 Lecture 3 (NP03): Intestinal Phases Duodenal cluster unit: combination of the duodenum, stomach, biliary system (gall bladder and the gall duct) and the exocrine pancreas that collaborate. The liver is not included, because the bile acids it synthesizes are stored in the gall bladder, which releases them into the duodenum. - Duodenum: secretion of hormones, intraluminal and surface digestion, absorption, motility throughout the whole GI. The duodenum gives feedback to the stomach that delay gastric emptying, providing enough time to digest the food properly. It is constantly mixing it contents by muscle contractions. The stomach content comes into the duodenum, regulated by the pylorus. The enterocytes lining the lumen secrete water and HCO3- into the duodenum. Secretion by the pancreas and the biliary tract is regulated by measuring nutrients, osmolality and the pH. Secretin regulates addition of HCO3- (by both the pancreas and the biliary secretion) to make the pH higher because of acidic contents from the stomach. pH: determined by the gastric acid that comes in, HCO3- from the pancreas, biliary duct and duodenal mucosa and H+ absorption. Bicarbonate increases pH because it catches acid and turns it into water and carbon dioxide. HCO3- + H+ H2CO3 H2O + CO2. CO2 is then exhaled and the pH is restored to normal. Buffers have an effect on the pH balance as well. S-cell: pH sensor cell for secretin secretion, which releases bicarbonate to restore pH. Osmolality: hypotonic and hypertonic meals become isotonic in the duodenum due to water uptake or intake. In the jejunum everything is isotonic. CCK influences the osmolality. From stomach antrum to duodenum: 2mm particles to macromolecules, emulsified triglycerides to micellar lipids, hyperosmotic to iso-osmotic, pH of 5-6 to pH of 7, protonized protein to solubilized protein, indigestible fibres are not changed. - Sphincter of Oddi: the pancreatic duct and the gall bladder duct fuse into one at the sphincter of Oddi, which is the gatekeeper for release of the fluids into the duodenum. It opens when there is food present. - Biliary Tract: releases bile and bicarbonate (increases pH, HCO3-) into the duodenal lumen. - Exocrine pancreas: excretes digestive enzymes, water and bicarbonate into the lumen of the duodenum, which is “outside” of the body. CCK, GRP and VIP hormones stimulate the release of digestive enzymes from the pancreas. Acetylcholine gives neuronal stimulation. Pro-enzymes (zymogens) are released into the lumen via granules that fuse with the membrane. Secretin stimulates water and bicarbonate release into the lumen. Acinus: storage of pro-enzymes. Ductule: enzymes are released into the ductule, via which it empties into the duodenum. Secretin is released. Monitor peptide and CCK-releasing peptide: Pancreas releases a monitor peptide to see if the digestion of peptides is ongoing or fully finished. If the peptide is present, it will activate cells that induce CCK release. CCK is also activated by free fatty acids and amino acids. Trypsin will degrade this monitor peptide into smaller fragments that don’t trigger CCK release anymore, this is a signal that protein digestion is complete. Then CCK-Releasing Peptide is released, which induces CCK release. When trypsin also digests that, CCK will go down. Constant monitoring of protein digestion. This needs to be completed before the next food bolus comes into the duodenum. CCK: CCK has different effects at different sites. It stimulates the DVC from the stomach and duodenum. Then, in both the stomach and the duodenum, this causes decrease in gastric acid secretion, decreased 6 gastric emptying and increased pancreatic digestive enzyme secretion (relaxation of sphincter of Oddi) to enhance digestion. CCK also leads to contraction of the gallbladder, releasing bile into the duodenum for lipid digestion. CCK acts both neuronally and as a hormone. When free fatty acids flow in, the gallbladder volume decreases (empties itself), the sphincter of Oddi pressure goes down, and plasma CCK increases. With a CCK antagonist, there is no hormonal activation and the gallbladder volume does not go down as much, because it then only acts via neuronal activation. This shows that the hormonal effect of CCK is much larger than the neuronal effect. They enhance each other. Bile acids: the major part of the solutes in bile. Needed for lipid digestion. They are synthesized in the liver, then stored in the gallbladder. When the gallbladder contracts, they are released into the duodenum. There they will help lipid digestion. In the ileum they are reabsorbed again into the liver via the capillaries. Only a small part is lost. Digestive (pro-)enzymes of the pancreas: Trypsinogen, procarboxypeptidase. Mostly pro-enzymes that are proteolytic, that need to be activated first. At the brush border, there are enzymes that perform the last steps of digestion and this is where absorption takes place and where proenzymes are activated. When trypsinogen (pro-enzyme) binds to enterokinase (endopeptidase), on the enterocyte brush border, it is cleaved into its active form, trypsin. Trypsin can then cut all the other proenzymes to activate them. They will then digest the dietary proteins. Trypsin can also cleave trypsinogen (as with pepsinogen and pepsin). Trypsin is highly specific, dependent on the amino acid sequence. Enzyme activity is influenced by: pH of incubation or environment, temperature, concentration of enzyme, concentration of substrate, inhibitors and activators. Different between organs. pH differences between arterial and venous blood: going to and from the organs. The arteries supply blood and then the veins take up H+ and bicarbonate (HCO3-) is released into the lumen. All organs that secrete bicarbonate make their veins slightly acidic, therefore the pancreas, gall bladder and the small intestine (to a much smaller extent) have slightly acidic venous blood. In the stomach it is the other way around, bicarbonate is taken into the veins, while H+ is released into the lumen. Small Intestine Duodenum: secretion of CCK, secretin, bicarbonate. Most of the digestion and absorption. Microvilli. Jejunum: no secretion. Digestion and absorption still take place. Microvilli are also present. Ileum: Secretion of PYY and bicarbonate. Digestion and absorption still take place. Microvilli are also present. Ileocecal valve: sphincter dividing the ileum and the colon. Nutritional paradox: balance between diet and use of nutrients. Food is not made for our nutritional requirements, but their natural function. Not all foods are nutritious. In diabetes, your body wants carbohydrates, but that doesn’t mean that it is good for you. It leads to metabolic disturbances, increases fat deposits and high blood sugar. 7 Digestive enzymes - Proteases: cut proteins into oligopeptides and amino acids, then the brush border peptidases cut them into dipeptides, tripeptides and amino acids, which are absorbed by the enterocytes. - Amylase (maltase): digests starch and produces (iso) maltoses, dextrins and maltotrioses. Glucoamylase on the brush border produces glucose, which is absorbed. - Nucleases: degrade the DNA and RNA in the food and makes nucleotides, then alkaline phosphatase on the brush border makes nucleosides that can be absorbed into the enterocytes. Phosphate is cleaved off. - Lipolytic enzymes: lipase makes 2-monoglycerides and fatty acids, cholesterol esterase makes cholesterol and fatty acids and phospholipase makes lysophosphatidylcholine and fatty acids. No brush border enzymes are involved, they are absorbed differently because they are hydrophobic. Digestion of macronutrients Carbohydrates: hydrolysis of starch by amylase makes glucoses, isomaltose (branched) and maltose (linear) in the small intestine lumen. These are then digested into glucose by the brush border enzymes and are absorbed. Glucose and galactose are the end products. SGLT is a different solute carrier family compared to GLUT, proteins names are all SLC. The majority of glucose is absorbed in the jejunum. When pure glucose is given, 100% will be absorbed, the mean for carbohydrates is 98%. It is still not entirely clear which transporters are involved (see differences). Glucose and galactose: are co-transported with Na+ into the enterocytes. Na+ is pumped back into the lumen with the Na+ pump and Na+/K+ ATPase, which requires ATP. They can also be transported passively, dependent on the situation. Fructose: is not co-transported with sodium but is absorbed via passive diffusion. Proteins: Luminal, brush border and intracellular digestion. Proteins are cut into oligopeptides in the lumen by proteases and pepsins, then those are cut by brush border peptidases into amino acids and dipeptides and tripeptides, which are then absorbed into the enterocytes. Part of the di/tripeptides are further digested to amino acids by peptidases before entering the bloodstream, the other part is absorbed as small peptides into the bloodstream. Some necessary amino acids are taken up from the bloodstream when they are not present in dietary proteins. There are 5 different transporters because of the variety of amino acids. Amino acids are co- transported Na+ as well, di and tripeptides with hydrogen. Na/K ATPases are involved again. Mean absorption from food is around 93% and the majority is absorbed in the jejunum, hardly in the duodenum. Takes longer than carbohydrates. Protein digestion takes place in the gastric and the intestinal phase. 8 Fat: Fat droplets, containing triglycerides and phospholipids, are surrounded by bile salts. These are digested by pancreatic lipase, which cleaves of fatty acids from triglycerides forming monoglycerides and fatty acids. These can form micelles or are absorbed by the enterocytes. Free fatty acids are re- esterified into triglycerides because high free fatty acid concentration is toxic. They are then captured into chylomicrons, which travel via the lacteal to the lymphs. They don’t enter the bloodstream via the portal vein. The fatty acids and fat-soluble vitamins travel to the heart first, and reach the liver very late in the body because they are absorbed via the lymphatic system. 95% of fat is absorbed and the majority is absorbed in the jejunum. No brush border enzymes are involved. Cholesterol: is used as a precursor for synthesis of bile acids. Major lipid metabolism component. Bile salts: Hydrophobic cholesterol groups, hydrophilic parts. Helps with lipid metabolism. Lipids form micelles with hydrophilic groups facing outwards and the hydrophobic parts facing inwards. PYY: When free fatty acids are sensed at the ileum, PYY is released at the end of the ileum and the start of the colon. They give a feedback signal that slows down digestion, decreasing gastric acid secretion, gastric emptying, pancreatic secretion, transit rate and colonic motility. This is done to enhance digestion of lipids and absorption. 9 Colon: Ascending colon, transverse colon and descending colon, the rectum with sphincters. Relaxing the sphincters releases the faeces. Colons differ greatly between species; plant eaters have enormous colons. The function of the colon is the absorption of water and ions, bacterial fermentation of unabsorbed nutrients, storage and elimination of waste and indigestible materials. The colon is stimulated by rectal distention (neural -> storage to propulsive motor pattern and defecation), intravascular volume depletion (humoral -> absorption of ions and water) and free fatty acids (PYY - > inhibition of GI secretomotor functions). The contents are completely mixed throughout the colon, some 36h old parts are at the start and others at the end for instance. Mixing is done via mixing movements (grinding of the contents, contracting and relaxation), haustral migration and mass movements. The colon is already neurally stimulated when food enters the stomach. When air is swallowed into the stomach, nitrogen and oxygen can be burped out or diffused in the intestines where they are ultimately exhaled. CO2 produced into intestines (H+ + HCO3-) can be exhaled or enter the colon. Microbial fermentation: The microbiota makes CH4, H2 and CO2 24h per day, which can diffuse into the circulation or farted out (flatus). When substrate delivery is increased, gas production is increased. They use indigestible fibres (non- starch polysaccharides) to produce these gases. Voluntary defecation reflex: closure of the glottis, descent of the diaphragm, contraction of the abdominal wall. Increases abdominal pressure. Involuntary defecation reflex: uncontrollable. Propulsive motility of descending colon and rectum. Relaxation of internal anal sphincter and external anal sphincter. Arterial Splanchnic blood flow: arterial blood flow, oxygen rich blood from the heart. Goes to the liver, pancreas, small intestine and the colon. Making oxygen and substrates available for the organs. When you eat, the blood flow increases for the mesenteric artery and decreases for the brachio- cephalic artery to support the absorption. Venous Splanchnic blood flow: the portal vein carries all the veins from the colon, small intestine, stomach and pancreas to the liver. The toxic compounds from the food are detoxified in the liver. The hepatic vein then carries the detoxified blood to the rest of the body via the heart. The portal vein is the only vein that does not transport to the heart. All the veins transport to the heart, except for the portal vein. Water flow in the intestines: 8.5 litres are transported to the intestines daily, 1.5 from oral intake, the rest from salivary glands, the stomach, bile, pancreas and the intestine. Only 200ml leaves the body via the stool, most goes to the small intestines. Sphincters Oesophagus: Upper Esophageal Sphincter, Lower Esophageal Sphincter Stomach: pylorus, Lower Esophageal Sphincter Small intestine: pylorus, ileo-caecal valve Colon: ileo-caecal valve , anal sphincters 10 Lecture 4 (NP3a): Bariatric Surgery, Ghrelin, Exam Questions GI-tract Bariatric surgery: There is an overwhelming increase in obesity, globally. To combat obesity, you need to increase the energy expenditure or decrease the intake. People don’t adhere to this, so bariatric surgery is a solution. You can reduce the gastric volume or you can reduce the intestinal digestion/absorption. This is applied at BMI >35. - Gastric banding: band is inserted at the top of the stomach. This reduces the volume of food that can enter the stomach at the same time. The intake goes down. An inflatable gastric balloon can also be used. This can be adjusted in time. Decreases energy intake but the stomach and ghrelin production are still fully functional. - Roux-en-Y Gastric Bypass (RYGB): A part of the small intestine is added to the start of the stomach and then the end joins together with the small intestine that did start at the end of the stomach. That part does not get any pancreatic enzymes, leading to a malabsorptive component. Inhibits absorption in part of the small intestine. A side effect is that compounds enter the colon that don’t normally, causing gas production. Ghrelin levels go down. Pancreatic juices and gall are together for a much shorter time. - Sleeve Gastrectomy: cut off a large part of the stomach. It is still fully functional, but reduces energy intake. - Biliopancreatic diversion with duodenal switch (BPD with DS): Stomach size is reduced as in sleeve gastrectomy and the duodenum is switched. The duodenum is cut around the pylorus, and the jejunum is cut next to the ileocecal valve and attached to the duodenum. High malabsorption and vitamin decrease. A large part of the small intestine just transports pancreatic juice to the end of the small intestine. The gallbladder is completely removed. Non-surgical procedures Endobarrier therapy: hollow tube put in the small intestine and blown up. The food is now transported via the hollow tube, while the pancreatic juices are on the outside of the tube. It can be removed. Duodenal Mucosal Resurfacing: the whole surface area of the small intestine is destroyed; the body then renews the surface. They flush in very hot water with an endoscope, destroying the surface area. This has major benefit for pancreas signalling. Ghrelin: stomach releases it upon food entering the stomach. A very small protein of 28 aa with a fatty acid group attached. Endogenous activator via receptor cascade leading to growth hormone release. Is regulated by a whole cascade. Prepro-ghrelin needs to be cleaved, the signal peptide 5’ is cleaved off, the 3’ end is cleaved off. Then the active hormone remains. Upregulation: Fasting, low BMI, Leptin, thyroid hormones, testosterone. When the stomach is empty. Downregulation: high BMI, food intake, glucose, insulin, somatostatin, growth hormones. When the stomach is stretched. Effects: increases growth hormone release, cortisol, prolactin, appetite, immunity, sleep, lower metabolic rate/thermoregulation. Increases food intake in rats. Influences many things. When ghrelin is injected in the intracerebroventricular region of the brain, it has an even stronger effect. Has a long time effect and increases weight gain while food intake is not increased in mice. Independent of growth hormones (GH- rats), weight gain is increased. It’s more about the total energy in the stomach, not about the stretching. Ghrelin levels go up before meals and after losing weight, causing an increase of energy intake. Gastric bypass: the ghrelin levels go down significantly to almost the detection level. Even at the same BMI. 11 Exam Questions Homeostasis is the ability of the body to counteract physiological conditions that could disturb vital functions of the body. The digestion of carbohydrates to absorbable sugars is associated with the translocation and transformation of carbohydrates in the digestive tract. The vagus affects gastro-intestinal responses during the cephalic phase, these responses include the motor and the secretory behaviour of the gastrointestinal tract After the oral phase gastric motor and secretory behaviour can be maintained by the presence of oligopeptides in the stomach, not the pH or proteolytic enzymes. The amount of energy released from the stomach to the duodenum in kJ/min is larger than the normal energy expenditure of the body. Normal energy expenditure is (2000 kcal * 4.18)/(24*60)=5.81kJ/min while energy released from the stomach is 8.5 kJ/min. The colon can be stimulated to secrete the regulatory peptide PYY. This secretion is a response to the passage of undigested/unabsorbed: a. sugars b. amino acids c. fatty acids d. nucleic acids Which monomeric component is not absorbed by co-transport of Na+? a. Glucose b. Fructose c. Galactose d. Lactose -> Not a monomeric component! Mean absorption in a human diet Protein: 93% Lipids: 95% Carbohydrates: 98% Chylomicrons have a larger diameter compared to micelles. Functions of HCl secreted by the stomach wall: to optimize the pH for pepsin-activity in the lumen of the stomach, the conversion of pepsinogen into pepsin and killing bacteria in the food. The muscles in the stomach differ greatly between the fundus and the antrum. Different functions. Amylase occurs in both saliva and the juice of the exocrine pancreas. Assuming normal nutritional conditions, what is true with respect to anabolic and catabolic processes during the post-absorptive phase of a meal? 12 Lecture 5 (NP04): Nutritional Energy and role of ATP Nutrients: raw materials that keep you alive. They still need to be processed by the metabolism. Metabolism: series of chemical reactions that change the raw materials into products or energy that can be used by the body to keep you alive. Immaterial output: maintenance of body, heat, energy. Input to waste -> CO2 and urea Material output: growing/producing body. Storage of TG. Input to product -> muscles or fat. Energy sources in blood: glucose, ketone bodies, fatty acids, lactic acid and amino acids. The brain is the top priority and uses the most glucose. It is highly dependent on glucose, and ketone bodies only supply energy to the brain when glucose is lacking. Fatty acids and lactate can’t supply energy to the brain. Muscles are not dependent on lactate. The liver and heart mainly uses fatty acids. Gross Energy (GE): How much energy a food product contains. kJ/gram. Can be shown by the heat released during combustion (oxidation). This is done by a bomb calorimeter, the temperature increase from burning food is measured to determine the energy in the food. In a bio calorimeter an animal is surrounded by ice, then the amount of melted ice is tracked after eating. Specific to the food, not to the animal digesting it. Glycogen = Cellulose = Amylose = Amylopectin > Saccharose = Maltose > Glucose. Cellulose and amylose have lots of glucoses, they therefore have higher gross energy. Digestible Energy (DE): how much of the raw material can be turned into energy, gross energy minus what is lost via faeces. Dependent on the digestibility of a food, which is normally considered constant. Carbohydrates: 98% is absorbed in humans. So 98% of gross energy will be DE. Fats: 95% is absorbed in humans Protein: 93% is absorbed in humans Metabolizable energy (ME): Digestible energy minus the urine and gas. This is the energy that is deposited in tissues and used as energy source. Part of the metabolizable energy is lost as heat and part is turned into ATP for maintenance, production and physical activity. The kcal on a pack is the metabolizable energy. The metabolizable energy is different per animal. Amylose > maltose > glucose > cellulose Carbohydrates: no urinary loss. 4 kcal/gram Fats: no urinary loss. 9 kcal/gram Protein: 5.2 kJ/gram of protein is lost in the urine. 4 kcal/gram 1 kcal: 4.186 kJ, the energy needed to heat 1 litre of water by 1 degree. ATP: energy units put into mechanical, electrical and chemical work. Base (Adenine), 5-carbon sugar. ATP has three phosphates. GTP (Guanine) and UTP (Uracil) have the same energy. ATP formation requires the input of energy. ADP + Pi ATP (33kJ/mol). In ATP there are three phosphate groups. In AMP there is only one, and it contains no energy that can be released. ATP contains 2 energy units and this can be reduced to ADP (1 unit released) or AMP (2 units released) Emergency route: 2 ADP -> ATP + AMP Direct production: substrate phosphorylation, anaerobic. Kinases transfer phosphate groups from substrates to ADP, making it ATP. Indirect production: oxidative phosphorylation, electron transport chain, aerobic. Without a sponsor. Redox reactions. Kinase: transfers phosphates from a substrate to ATP. 13 Exothermic reaction: energy is released. ATP is gained. We invest less than what we gain, favourable reactions. More energy than the activation energy is released when the new bond is formed. Endothermic reaction: less energy than the activation energy is released when the new bond is formed and endothermic reaction requires an input of energy to proceed. We invest more than what we gain. Unfavourable reactions. ATP +H2O (H+ + OH-) to ADP + Pi. Hydrolysis. Activation energy: energy needed to break a chemical bond. ATP functions Sodium pump: Generation of a proton gradient. H+ from inside the cell and OH- from outside of the cell are used for endothermic ATP->ADP reactions. Sodium will then be pumped out of the cell, which can act as a cotransporter to the inside of the cell or for exporting waste from inside to outside of the cell. ABC-transporter: can transport cholesterol out of the cell. ATP causes phosphorylation of the transporter, opening it. ATP becomes ADP. Transport of the substrate causes dephosphorylation of the transporter, closing it again. ATP can change the configuration of the transporters. Metabolic trapping: Glucose is phosphorylated to transform in to glucose 6-phosphate, so it can’t go out of the cell anymore and can be further metabolized. Glutamate + ATP (intermediate step: -> glutamyl phosphate) -> glutamine + ADP. Protein phosphorylation: phosphate tag to regulate activity of proteins or enzymes. PDHC (Pyruvate dehydrogenase complex): Pyruvate + NAD+ +CoA -> CO2 + acetyl-CoA + NADH. When it doesn’t have the phosphate group it is active, with the phosphate it is inactive. PDHC is the enzyme that catalyzes the reaction above. Metabolically irreversible! Creatine Phosphate: Creatine phosphate is helpful in short duration sports. Can easily turn ADP into ATP. This is stored in muscles and give a better sport performance in short duration. Pyruvic acid = pyruvate (we don’t use the acid form in this course) Energetic efficiency of trapping glucose into ATP 1 glucose = 30 mol ATP (33 kJ per mol) C6H12O6 + 6 O2 -> 6 CO2 + 6H2O + 2816 heat (energy gained) 30 * 33 = 990 trapped in ATP. 2816-990 = 1826 released as heat Efficiency=990/1826=35% 14 Lecture 6 (NP05): Conversion of Energy ATP -> ADP: 1 ATP unit of energy is released ATP -> AMP: 2 ATP units of energy are released 2 ADP -> 1 ATP + 1 AMP: emergency energy Body (70kg): 3 mmol ATP/kg. MWATP=507.18 g/mol ATP stored: 70 * 3 = 210mmol ATP. 210 * 507.18 = 106507.8mg= 106.5078gram How much glucose needed to cover a maintenance requirement of 8500kJ/day? (Glucose = 15.6 kJ/gram, MWGLU=180g/mol): 8500/15.6=544.87gram. 544.87/180=3.027mol The same for ATP (30ATP per glucose)? 30 ATP * 3 mol = 90 mol ATP * 507.18 = 45 kg ATP What problem shows up and how is it solved? We need a lot of ATP, so we need a very efficient method to do that Oxidation - Reaction with oxygen: sugar + 6 O2 -> 6 CO2 + 6H2O. Oxygen is the electron or H acceptor. Anaerobic bacteria may also use S or C as the H- acceptor, H2S or CH4) - Withdrawal of electrons: Fe2+ -> Fe3+ + e- - Dehydrogenation (Substrate oxidation): oxidation of alcohol to aldehyde by dehydrogenases. Hydrogen + electron is lost from substrates (XH2 +NAD+ -> X + NADH, H+). Substrate is oxidized. H-carrier is reduced, NAD and FAD coenzymes are temporary H-acceptors and become NADH and FADH. When NADH (2.5 ATP) and FADH (1.5 ATP) are regenerated to NAD and FAD, ATP is released. NAD(P)H coenzyme: 2.5 ATP. NAD is a 5-carbon sugar with a base and two phosphates (ADP), with a nicotine ring attached. NAD+ is positively charged. The cytoplasmic and mitochondrial NADH are not equivalent! Cytoplasmic NADH: cannot go into the mitochondria directly but has to be transported. The transporter turns the NADH into FADH, losing 1 ATP. It will only generate 1.5 ATP per cytoplasmic NADH. FAD(P)H coenzyme: 1.5 ATP. ADP with a riboflavin attached. In the oxidized form it is not charged, it can be reduced once or twice, then one or two H-groups are added. Mitochondrial electron transport chain: substrates are oxidized, NAD and FAD become NADH and FADH. Then that energy is put into turning ADP into ATP via the different compartments of the electron transport chain. The energy will flow through the cytochromes and O2 is put in, while ATP is gained. When NADH is used, more ATP is gained then when you start with FADH. Energy is used to pump the H+ protons from the matrix to the crista space. Then this proton gradient is built up and used to drive ATP synthase, which pumps the protons back to the mitochondrial matrix. 1 NADH pumps out 10 H+ in total, per ATP you need to put 4 H+ through the ATP synthase, so 2.5 ATP per NADH is gained. FADH (succinate) will use complex II, therefore skipping complex I and pumps only 6 H+, generating 1.5 ATP. Can be 15 measured with instruments such as Seahorse. Sometimes electrons escape and will react with oxygens, becoming ROS, superoxides. Complex IV turns oxygen into water to make electrons, the ultimate electron acceptor. We breathe to give the oxygen needed, and we eat to give the FADH and NADH for the electrons. Superoxides: can attack DNA or fatty acids in the cell membrane. Cell death. Uncoupling proteins (UCP): H+ flows back into the matrix, not generating ATP but heat. This is also used to increase weight loss, but that is very dangerous. Mitochondria: powerhouse of the cell. Sites for energy production that are in all cells except mature red blood cells (they only use glycolysis -> lactate). Has cristae inside of the membrane, where oxidative phosphorylation (electron transport chain) happens. In the matrix the TCA cycle happens. Formed as a spaghetti noodle. Each mitochondrion has its own DNA. Outer mitochondrial membrane: is much more permeable than the inner mitochondrial membrane. RQ ratio: to completely oxidize 1 glucose, all carbons have to go to 6 CO2, so 6 water will be formed and 6 O2 is also needed. RQ ratio is then 1, because 6 O2/6 CO2 = 1. 1 Glucose will give 24 electrons. Each oxygen (O-) can only accept 2 electrons. So, in total 6 oxygen (O2) atoms are needed. TCA cycle: happens in the mitochondrial matrix. Always starts from Acetyl-CoA, no matter what the product is. Cyclic catabolic pathway. Oxaloacetate is the vehicle, while acetyl CoA is the passenger. Oxaloacetate is turned back into citrate and the cycle restarts. Don’t remember the substrates and enzymes, only what happens. Dehydrogenases catalyse oxidation reactions. In the decarboxylation steps, CO2 is a by-product (no oxygen involved). 3 NADH (7.5 ATP), 1 FADH (1.5 ATP) and 1 GTP are won from this cycle, this yields 10 ATP in total (and 2 CO2). Free fatty acids always go through Acetyl- CoA, but carbohydrates can start via pyruvate (made in glycolysis), making Acetyl-CoA (start of TCA cycle) or directly oxaloacetate (end of TCA cycle, more vehicle), depending on the need. Citrate synthase: the rate limiting enzyme of the TCA. When it is inhibited, the whole citric acid cycle will be blocked. Citrate synthase can be inhibited by high ATP/ADP, Acetyl- CoA/CoA, NADH/NAD+ ratio (500:1) or high substrate concentration (citrate and succinyl- CoA). C incorporation: the carbons that leave the vehicle are from oxaloacetate and not from Acetyl-CoA. The building blocks are not identical but interchange in every cycle because the vehicle carbons leave via CO2. Nett reaction: AcCoA + OAA -> OAA 2CO2 + 3 NADH + 1 FADH + GTP. AcCoA + OAA - > OAA 2CO2 + 10 ATP. AcCoA -> 2 CO2 + 10 ATP. Three ways to write it. 16 Lecture 7 (NP06): Carbohydrate metabolism I: Glycolysis Carbohydrates Simple: monosaccharides and disaccharides. Contain Cn(H2O)n. In the nucleotides ribose (RNA) and deoxyribose (DNA) are very important. These all have a high GI, glycaemic carbohydrates. - Monosaccharides: Glucose, fructose and galactose are all monosaccharides. 6 carbon sugars. They are differently arranged, but they all have a ring structure. The difference is that the groups of OH groups are cis or trans between glucose and galactose and 2 of the carbons are in side groups in fructose so the ring is 1 carbon smaller. - Disaccharides: sucrose (saccharose), trehalose, lactose, maltose (2 glucose in alpha 1-4 bond) and isomaltose (2 glucoses in alpha 1-6 bond). 2 monosaccharides connected via an alpha bond. The difference between the disaccharides are the composition of monosaccharides or the location of the bonds. When two monosaccharides form a bond, H2O is released. When they are split, H2O is needed for hydrolyzation. - Sugar alcohols: are derivatives of simple sugars - Intrinsic sugars: sugars within the cell wall or milk. Oligosaccharides: 3-10 sugar. The GI depends on the structure and bounds. Polysaccharides: >10 sugars. Sugar chains that are connected on different parts of the chains with other chains. Polysaccharides are cut into fragments. Glycogen and starch have a similar gross energy. The GI depends on the structure and the bounds. Linear starches have a low GI, branched starches have a high GI. Non-starch polysaccharides: are not digestible by humans, because they have no enzymes to digest them. They have beta bonds instead of alpha bonds, which can’t be cut by our digestive enzymes. Pectin, chitin and cellulose. Glycaemic Index: a measure of how fast a carbohydrate increases the blood glucose compared to the equivalent amount of glucose. The lower the value, the slower it is digested. When you compare a high GI diet with a low GI diet, the mice with the high GI diet will have higher adipose tissue, higher free fatty acids and triglycerides in the blood. They become less healthy. High glycaemic index: branched starched have a higher GI. Low glycaemic index: not all digested in the small intestine. Low digestible starches have a low GI and produce more gas. He said that they were high GI but that’s not correct…. Glycolysis: glucose-6-phosphate to pyruvate. Happens in the cytoplasm. When you are fed, you will have a lot of glucose in the blood, this will be absorbed by the liver. The energy from glucose will be released in steps of energy. Glucose + (2ATP) + 2 ADP + Pi + 2 NAD -> 2 pyruvic acid (pyruvate) + (2ATP) + 2 ATP + 2 NADH. At the beginning 2 ATP has to be invested. 1. Glucose-6-phosphate: Phosphorylation with 1 ATP by hexokinase (or glucokinase) will transform glucose into glucose-6-phosphate. With this tag, it can’t go out of the cell anymore. In the fasted state, glycogen is used to restore glucose, reversible and this does not require ATP. 2. Fructose-6-phosphate: phosphohexose isomerase turns the glucose into a fructose. Reversible. 3. Fructose-1,6-biphosphate: phosphofructokinase uses 1 ATP to add another phosphate. This is another tag, to commit this fructose to be metabolized and not to be converted to other sugars. Reversible. The phosphofructokinase enzyme is stimulated by AMP and inhibited by ATP and citrate. 4. Dihydroxyacetone phosphate + Glyceraldehyde-3-phosphate: Fructose-1,6-diphosphate splits into 2 3C sugars by aldolase. First the dihydroxyacetone phosphate has to be turned into glyceraldehyde-3-phosphate by triose phosphate isomerase. After that there are 2 of those. 17 5. 2x Biphosphoglycerate: glyceraldehyde-3-phosphate dehydrogenase oxidize the glyceraldehyde-3-phosphate, gaining 1 NADH per molecule, 2 NADH per glycolysis. Cytoplasmic NADH, so it will only give you 1.5 ATP! NADH has to be transported to the mitochondrion by the glycerophosphate shuttle and that the proton is then transported to the FADH, making only 1.5 ATP. Reversible, but it costs NADH. 6. 2x 3-phosphoglycerate: Phosphoglycerate kinase converts biphosphoglycerate to 3- phosphoglycerate. 1 ATP is gained per molecule. 2 ATP per glycolysis. Reversible, but costs ATP. 7. 2x 2-phosphoglycerate: reversible, done by phosphoglyceromutase. 8. 2x Phosphoenolpyruvate (PEP): enolase converts the sugar into phosphoenolpyruvate, gaining H2O. 9. Pyruvate: pyruvate kinase turns PEP into pyruvate and makes 1 ATP in that reaction. 2 ATP per glycolysis. 1-way only, because that reaction is very favourable. Another route is needed to make go back. Glucokinase: isoform of hexokinase, usually found in liver. Only substrate is glucose. When you have a lot of glucose in the liver, it will trap it and store them as glycogen. It has a very large Km, so it will be switched on only at high concentrations of glucose. High activity. Hexokinase: switches on at very low concentrations of glucose. Low Km. Acts at its maximal rate under nearly all conditions to cover the normal demands of the liver metabolism. Glycogenolysis: lysis of glycogen to form new glucose-6-phosphate. Happens in a fasted state. Glycogen is turned into glucose-1-phosphate by glycogen phosphorylase and this becomes glucose-6- phosphate. Not all organs can turn glucose-6-phosphate into glucose, it is only possible in the liver (and the intestines) because glucose-6-phosphatase is only present in the liver. The sugars can’t be transported to other organs but have to be burned in the liver. Found by gene expression Gluconeogenesis: re-synthesis of glucose from glucose-6-phosphate, done by glucose-6- phosphatase. The opposite of glycolysis. Link between glycolysis and TCA cycle: pyruvate is imported into the mitochondria via a transporter to start the TCA cycle. Pyruvate dehydrogenase will then oxidize pyruvate to acetyl CoA (2 carbons) + CO2 + NADH (2x per glucose). The enzyme is regulated by phosphorylation, the phosphorylated form is inactive. The Acetyl CoA will enter the TCA cycle. 10 ATP per TCA cycle + 2.5 ATP from the NADH so 12.5 ATP per pyruvate. Per glucose 30 ATP is generated because of 2x pyruvate (25) + 2 ATP and 2 cytoplasmic NADH (-> 2 mitochondrial FADH -> 3 ATP instead of 5) from glycolysis. 4 ATP in glycolysis and 2 GTP in the TCA are formed by substrate-level phosphorylation. 2 FADH are made in glycolysis and 2 FADH in TCA, so that is 6 ATP. 18 Lecture 8 (NP07): Carbohydrate metabolism II ATP YIELD (full oxidation) Acetyl-CoA: 10 ATP Pyruvate: 10 from TCA + 2.5 from NADH (Mitochondrial) = 12.5 ATP PEP: 12.5 + 1 ATP from PEP to pyruvate= 13.5 ATP GA3P: 13.5 + 1 ATP + 1.5 from NADH (Cytosolic!) = 16 ATP Glucose: 30 ATP Citrate: 3 NADH + 1 FADH2 + GTP = 10 ATP Ketoglutarate: 2 NADH + 1 FADH + GTP = 7.5 ATP Succinyl-CoA: 1 NADH + 1 FADH + GTP = 5 ATP Fumarate/Malate: 1 NADH = 4 ATP Oxaloacetate: 0 ATP Full oxidation of citrate Citric acid -> Malate: 7.5 ATP Malate -> OAA (cytosolic): 1.5 ATP OAA -> PEP: -1 ATP PEP: 13.5 ATP. 10 + NADH (mitochondrial) + 1 ATP -> Total: 21.5 ATP from the complete oxidation of citrate. Because full oxidation always has to go through acetyl-CoA. You go through the TCA twice (-1 step), so 6 CO2 is released (2 per TCA and 2 CO2 in OOA -> PPA and 1 CO2 in pyruvate -> Acetyl-CoA. It doesn’t yield 30 ATP as glucose does, while both are C6, because citrate has losses. Citrate also produces only 1 Acetyl-CoA, while Glucose yields 2. Full oxidation of OOA OAA -> Malate: -2.5 ATP Malate -> OAA (Cytosolic): 1.5 ATP OOA -> PEP: 1 ATP PEP -> TCA cycle: 13.5 ATP (10 + 1 mitochondrial NADH + 1 ATP) Pyruvate -> Glucose (Gluconeogenesis): the glycolysis can’t be reversed, but there is another route. Pyruvate can be converted to oxaloacetate by pyruvate carboxylase and that is then converted to PEP. Pyruvate carboxylase is only found in the mitochondria. Oxaloacetate can’t be transported out of the mitochondria directly. It is first converted into malate, which can be transported out of the mitochondria. Then, outside of the mitochondria it can be converted back into oxaloacetate. That is then converted into PEP and then into glucose. 1 NADH (2.5 ATP) is invested in the mitochondria. 1 NADH is gained in the cytosol (1.5 ATP). 1 ATP is lost. Full oxidation: getting the full potential of energy out of the reactions. For this, oxaloacetate has to be converted to Acetyl-CoA, in order to gain more ATP. So OAA -> Malate -membrane-> OAA -> PEP -> pyruvate -> Acetyl-CoA. Complete oxidation always means that you have to go through Acetyl-CoA. Complete oxidation of fatty acids. Propionic acid will be converted into succinyl-CoA. In these reactions, ATP is converted into AMP and another ATP is converted into ADP, which costs 3 ATP in total. 19 Glycogenesis Human blood glucose: between 3-5mM, about 1 gram/L in 2.5 litres of plasma, so only 2.5 gram circulates. The glucose in our blood can only maintain us for 5-6 min. Therefore, we need to constantly supply glucose. When you are fed, glycogen is stored in the liver and in the muscle. When you are fasted, the body releases glycogen from the liver to the circulation, not the muscle because glycogen phosphatase is not present in the muscle (only in liver or kidney). Substrate cycling: glucose -> glycogen -> glucose. Glucose concentration does not tell you everything, you can have the same concentration, but the speed of the enzymes is very different. The enzyme speed is more important. Post prandial phase: digestion and absorption, input > needs, anabolic or catabolic disposal. Storage, interconversion and oxidation. Post absorptive phase: utilization. Input < needs, mobilization of energy. Turnover, interconversion and oxidation Glycogen synthesis: glucose -hexokinase +ATP-> glucose-6-phosphate -isomerase-> glucose-1- phosphate -ATP + uridyl transferase-> UDP-glucose. The UDP-glucose is then added to glycogen with an alpha-1-4-bound. 2 ATP are invested. Glycogenolysis: glycogen phosphorylase breaks down glycogen to glucose-1-phosphate without any ATP. Glucose-6-phosphate, the starting point for glycolysis, can be made without any use of ATP. Direct glycolysis: glucose -> G-6-P. costs 1 ATP. Yields 30 ATP. Indirect glycolysis: Glucose -> G-6-P -> Glycogen -> G-6-P. Glycogen -> G6P is free. Costs 2 ATP. So that yields 29 ATP (Direct glycolysis -1ATP). Indirect oxidation in muscles can’t be transported to other muscles! Via liver it will only yield 28 ATP, because the capture during transport to the muscle costs 1 ATP extra. 1 glucose-glycogen cycle costs 1 ATP, because glucose-6-phosphate is the starting point, so after 5 cycles you lose 5 ATP and the gain is only 25 ATP. Phosphofructokinase (PFK): once glucose-6-phosphate is digested by PFK, the glucose can only be burned and not used for any other purposes anymore. One-way reaction. It is regulated by ATP and acetyl-CoA. Inhibition by ATP, stimulation by AMP. Inhibition by citrate (enough energy), stimulation by low O2. The rate-limiting step of glycolysis. Pasteur effect: switch to fast glucose use due to hypoxia. Aerobic glycolysis to anaerobic glycolysis. Glucose + 2 ADP + Pi + 2NAD+ -> 2 pyruvic acid + 2 ATP + 2 NADH. The oxygen is the electron acceptor, which go to NADH. When NADH has to be turned back into NAD+, oxygen is needed. When there is no oxygen, in hypoxia, pyruvate will be converted into lactate (electron acceptor) to regain the NAD+, which allows the glycolysis to continue. When there is no oxygen, only 2 ATP and 2 lactate will be gained per glucose, compared to 30 ATP in aerobic glycolysis. The Pasteur effect is a 15x increase in glucose utilization. ATP: 33 kJ/mol GEglucose: 2816 kJ/mol GELactate: 1364 kJ/mol Aerobic: 30 ATP. (30*33)/2816=35% is lost Anaerobic: Glucose -> 2 ATP + 2 Lactate. 2*1364=2728 kJ. 2816-2728 kJ= 88kJ is lost. In 2 ATP there is 33*2= 66kJ. So 66/88=75% energy efficiency. The rest is lost as heat (22kJ). 20 Lecture 9 (NP08): Pentose Phosphate Pathway Pentose phosphate pathway (PPP): NADPH production in the cytoplasm. The only source of NADPH for red blood cells. The pathway for synthesis of 5-carbon sugars. Source of 50% of NADPH needed in fatty acid synthesis. Can only happen in the liver, the mammary gland and adipose tissue. Glucose-6- phosphate is the substrate. It can be turned into fructose-6-phosphate by dehydrogenases (NADPH is created as a by-product). The next product is ribulose-5-phosphate, creating NADPH and CO2. The ribulose-5-phosphate is also used to create nucleotides. The substrate is 3 x glucose (C18). The end products are 2 fructose-6-phosphate (2 x C6) and 1 glyceraldehyde-3-phosphate (C3). In total 15 carbons are gained and 3 CO2 is lost. 3 moles of glucose-6-phosphate will result in 6 mole NADPH+ from NADP+. 3-G-6-P -> 2 F-6-P + GA-3-P + 6 NADPH + 3 CO2. Glycolysis vs PPP: If 3 G6P goes into glycolysis, and 2 G6P + 1 GA3P has to be made extra, 15 ATP will be gained from the 1 GA3P that is lost in PPP. In the PPP only 6 NADPH will be gained from making 2 F6P + 1 GA3P from 3 G6P. So both processes lose 1 GA3P to make either 15 ATP or 6 NADPH, the remaining 2 G6P and 1 GA3P can still proceed in the glycolysis after both. Energetics of reverse reactions Substrate cycles: The forward reaction costs energy and the backward reaction doesn’t deliver energy. In forward, ATP is invested and the backwards reaction does not cost any ATP. A lot of heat will be generated. - Hexokinase: only ATP invested in forward reaction. - Phosphofructokinase: only ATP invested in forward reaction. - Pyruvate kinase: only ATP invested in forward reaction. Neutral energetics: energy is invested for the forward reaction, the energy is gained back in the backwards reaction, so no energy is gained or lost. - G6P dehydrogenase: NADH is gained in the forward and invested in the backward reaction. Gluconeogenesis: 1 Glucose to 2 Pyruvate and back to 1 glucose loses 6 ATP. The PEP -> pyruvate reaction is one way only, but when pyruvate is turned into oxaloacetate, then malate and transported out of the mitochondria and then turned back into PEP it can still be made. This costs 2 ATP and 1 mitochondrial NADH is invested (2.5 ATP) and 1 cytosolic NADH (1.5 ATP) is gained. Trace the steps in the map. Note that there are 2 G6Ps per glucose and which ones are substrate cycles or neutral. Indirect oxidation: goes through gluconeogenesis and then glycolysis. Feedback signaling High Acetyl-CoA: signal to convert to oxaloacetate, to spend the energy. High AMP – Low ATP: stimulation of the citric acid cycle, condensation of oxaloacetate and acetyl-CoA production. Need for energy. Low AMP – High ATP: stimulation of gluconeogenesis, oxaloacetate is converted to PEP and glycolysis is inhibited. 21 Lactic Acid Pathway: when no oxygen is available as a terminal electron acceptor, pyruvate is used as the terminal electron acceptor and turned into lactate by lactate dehydrogenase in the muscle. This process regenerates 1 NADH to 1 NAD+ per pyruvate. Overall 2 ATP is gained per glucose in the lactate pathway, while from aerobic glycolysis 30 ATP is gained. Lactate is transported from the muscle to the liver. In the muscle pyruvate is turned into lactate, transported to the liver and turned back into pyruvate there. Some tissues adapt better to anaerobic conditions. Red blood cells do not contain mitochondria and only use the lactic acid pathway. Happens during high intensity exercise. Myocardial ischemia: It does not harm the skeletal muscle, but can hurt the cardiac muscle, because that normally respires aerobically. Can cause myocardial ischemia under aerobic conditions. Muscle energetic efficiency: aerobic 35%, anaerobic 75%. Only 25% of energy is lost as heat in the anaerobic phosphorylation Hypoxia acclimatization: adaptation of the body to hypoxia condition, used by top athletes to improve endurance. Oxygen levels of 12% causes stress on the metabolism. In a low-fat diet, the fed respiratory exchange rate is higher and at low oxygen levels, the fasted RER is also higher. Animals on a low-fat diet depend more on oxygen consumption and mice on a high-fat diet depend more on fat oxidation. Gene expression was different between diets. Cori Cycle: The conversion of lactate back to glucose requires 8 ATP for 2x pyruvate -> glucose and 2x transport of OOA out of the mitochondria (6 + 2). In the liver lactate is turned back into pyruvate first by the same lactate dehydrogenase as in the muscle, ----- 2 ATP but it performs the opposite reaction. Glucose is then returned to the muscle. Complete oxidation of Propionate: Propionic acid is first converted to succinyl-CoA, 3 ATP is invested in two steps. Propionate enters the TCA cycle as Succinyl-CoA. In total 13.5 ATP is gained for the direct oxidation of propionate. You always have to go through Acetyl-CoA and a complete TCA for a complete oxidation. Then malate is shuttled out of the mitochondria, and via pyruvate the TCA can fully start. 3 ATP is lost from propionate -> succinyl CoA. And from succinyl CoA -> Acetyl- CoA, 2 ATP, 1 mitFADH, 1 cytNADH and 1mitNADH and are gained, and 1 ATP is lost. This equals -2 ATP + 1.5 +1.5 + 2.5 = 3.5 ATP. Then 1 cycle of the TCA generates 10 ATP. In total the complete oxidation of propionate yields 13.5 ATP. 22 Lecture 10 (NP09): Amino Acid Catabolism Protein turnover: Nitrogen, derived from the amino acids, is released via the urine (70g) and the faeces (10g). The intake is 80g, so proteins are circulated and lost via the body. Constant degradation and synthesis of proteins. Catabolism of amino acids gives NADH and oxidative phosphorylation gives CO2, H2O. PKU: phenylketonuria, genetic disease. They lack one enzyme that degrades phenylalanine. Causes severe retardance. Patients receive a special diet lacking phenylalanine. Amino acid: backbone, nitrogen group and an acid group (=O, OH). Aromatic amino acids have ring structures. Methionine and cysteine have sulphur groups. Hydrophobic and hydrophilic amino acids. Large diversity between amino acids. On average amino acids have a 17% nitrogen content. 300 mM 1-4 mmol/L in the blood, very low levels because high levels are toxic. Proteins are immediately degraded and turned into amino acids, released in the circulation and taken up into tissues for storage or new proteins, for balance, nitrogen is also lost in faeces and urine. Amino acids have a molecular weight between 75-203, but the average is 137. - Essential amino acids: 9 amino acids. Can’t be synthesized in the body. You need those in your diet. Are not always limiting, only when the supply is not good enough. When the levels are not sufficient due to incomplete dietary intake, they become limiting. - Semi-essential amino acids: cysteine and tyrosine can be synthesized from essential amino acids methionine and phenylalanine, respectively. If there are enough essential. - Non-essential amino acids: alanine, aspartic acid and glutamic acid can be synthesized from pyruvate, 3-phosphoglycerate, alpha ketoglutarate, oxaloacetate and phenylalanine. As long as there is adequate nitrogen. - Conditional essential: become essential in certain conditions. For instance, during growth. glycine is conditional essential for chicken, because they convert nitrogen into uric acid, if the dietary intake of glycine goes below 25%, nitrogen can’t be excreted enough. - Limiting amino acids: are always essential, because non-essential amino acids are never limiting, as they can be synthesized. - Non-protein amino acids: citrulline and ornithine from the urea cycle are not used for protein synthesis. Not all amino acids are used for protein synthesis. Entry of amino acids into the citric acid cycle - Glucogenic amino acids: can be converted to glucose. Those with a c-backbone of glucogenic amino acids, they are degraded to intermediates of glycolysis or the citric acid cycle. If any component is used twice in the cycle, then the last one is used to calculate energy (asparagine -> aspartate -> oxaloacetate). If there are different options, it will depend on your needs. - Ketogenic amino acids: can’t be converted to glucose, they are degraded to Acetyl-CoA. Leucine and Lysine are strictly ketogenic and can never be used to synthesize glucose. 23 Amino acid Catabolism Carbon-backbone - Deamination and transamination: the c-backbone is de-aminated by either the oxidative deamination or the transamination. They dissociate the nitrogen group from the c- backbone. Full oxidation means oxidative deamination because you need to get rid of the nitrogen. Oxidative deamination: dissociate the amino group from the amino acid and release it as ammonium (NH4+). Dehydrogenases catalyse the reaction in the mitochondria and create NADH (2.5 ATP). Alanine, aspartate and glutamate can be converted to pyruvate, oxaloacetate and alpha-Ketoglutarate. Which can provide energy. Nitrogen is released. Then it can be released via the urea cycle. 2 ATP is invested per Nitrogen in the urea cycle. + 2.5 – 2 = 0.5 gained per nitrogen. TCA cycle gives 12.5 ATP, so 13 ATP in total for a 1 nitrogen amino acid. Transamination: exchange of nitrogen, it attaches to another molecule. A keto-acid accepts the NH3+ group and becomes a keto acid. Alpha-keto- glutarate (keto-acid) + Alanine -> Glutamate (amino acid) + Pyruvate. Aspartic acid to oxaloacetate, glutamic acid to alpha-ketoglutarate, glycine to glyoxalate. Nitrogen balance stays the same. Nitrogen-group - Urea cycle: Only in the liver. Nitrogen (ammonium) goes into the urea cycle. The cycle is partly in the mitochondria and partly in the cytoplasm. Happens only in the liver, because it is the only organ that has the enzymes. Excretion goes via the kidneys and the urine. Small aquatic organisms can excrete ammonium into a large volume of water outside. Terrestial animals have to convert it to less toxic compounds, 2N in 1 urea. If water balance is a problem (in eggs), uric acid is produced, therefore birds produce uric acid. 25% of their waste comes from glycine. People also have uric acid in their bodies, but very low concentrations. High levels of uric acid develop ‘Gout’ from eating excessive amounts of meat, then you get extremely sensitive toes. Derived from purine catabolism. Urea is the correction from DE to ME, energy is lost in urea synthesis. Pathway: a cyclic biosynthetic pathway. A cycle with add-on steps to an ornithine carrier. In the end, Urea is cleaved off and excreted. Costs 4 ATP to make urea, which contains two nitrogen, so a cost of 2ATP/N. Energy is lost during break down of amino acids (DE to ME). 2 ATP is used to trap it in the mitochondria. Ornithine-Citrulline antiporter shuttles the citrulline out and the ornithine into the mitochondria, with a 1 in and 1 out principle and keeps the cycle going. Oxaloacetate can also be converted to PEP -> glucogenic, but this is not part of the urea cycle and the ATP is not considered the total. Fumarate to aspartate can be reused in the urea cycle. Complete oxidation of amino acids: Alanine (1N) is deaminated to pyruvate, pyruvate full oxidation yields 12.5 ATP. 1 NADH is gained and 2 ATP is lost per Nitrogen (+0.5 ATP/N) in deamination, so in total 13 ATP is gained from full oxidation of alanine. Check how many N’s are in the structure. Gram glucose from 100 gram protein (MW=137): 100/137 = 0.73 mol PEP. 2 PEP = 1 glucose. 0.73/2 = 0.36 mol glucose. 0.36 *180 (MW glucose) = 66 gram glucose. Difference in ATP yield in full oxidation of amino acids: If they are converted into TCA intermediates, both have to go through Acetyl CoA by shuttling malate out. Trace TCA differences. Check Nitrogen content of both amino acids. 24 Lecture 11 (NP10): Fat and fatty acid metabolism Fats: no requirement for fats, except for small amounts of two polyunsaturated fatty acids, omega 3 (Linolenic acid, EPA and DHA) and omega 6 (Linoleic acid). Fat provides 39 kJ/gram (over 17 in protein and 16 in carbs), which is much higher, therefore it is easy to overeat. Lubricates food in the mouth and adds flavour. Vitamins A, D, E and K are present in dietary fat and require fat for absorption. Fat is stored without water, while protein (1 gram of protein with 4g of water) and carbohydrates (1 gram of glycogen for 2 grams of water) need a lot of water. So 10 kg of body fat corresponds to 60 kg of stored glycogen or 80 kg of stored protein. Nomenclature lipids - Triacylglycerol (triglycerides, TG): simple glycerol backbone that is esterified with three fatty acids. All fatty acids from animals and vegetables are even numbered, while micro- organisms have odd numbered fatty acids. Milk and vegetable triglycerides have very different structures. Milk has a saturated fatty acid on position 2 and unsaturated fatty acids on 1 and 3, while vegetable fats have the opposite. Very different absorption. Saturated fatty acids: don’t have any double bonds (C18:0), those are solid at room temperature because they are very straight and packed. Unsaturated fatty acids: have double bonds (mono or poly, C18:2) and are liquid at RT because of flexibility and a lower melting point. Double bonds are numbered from the methyl group. C18:3 means that there are three double bonds. Omega-3 means that the first double bond is at the 3rd carbon from the methyl-end of the fatty acid, it doesn’t say anything about the other double bonds. Omega 3 always has more than 1 double bond. Cis: carbons face the same side. Normal configuration in foods. Trans: carbons face different sides. Trans-fats have a major effect on health. They result from fermentation or hydrogenation (acidic environment) of oils to make mono unsaturated fatty acids from polyunsaturated fatty acids. Difference in structure. - Phospholipids: glycerol with two fatty acids, a phosphate group and a rest group. The phosphate group is negatively charged, making the head hydrophilic, while the tails are hydrophobic. They are used in membranes and are in between layers of oil and water for instance. Also used as emulsifiers. Bilayer of phospholipids in the cell membrane with the tails towards each other. Proteins can be in between the phospholipids. ROS can damage the unsaturated fatty acids, destroying the membrane structure and causing cell death. - Steroid hormones: cholesterol is a precursor for steroid hormones, cortisol, testosterone, progesterone and oestradiol. Substrate conversions: The conversion of pyruvate into acetyl-CoA is irreversible. The conversion of ketogenic amino acids into acetyl CoA is irreversible, while the conversion of glucogenic aa and pyruvate is reversible. Fatty acids can be converted into acetyl-CoA, but because that can’t be converted into glucose or pyruvate, fatty acids can’t be converted into glucose. 25 Lipid metabolism: Hormone-sensitive lipase (HSL) and adipose triglyceride lipase (ATGL) perform the first steps of lipid metabolism. Triglycerides are broken down into 3 free fatty acids and 1 glycerol and then glycerol can be used to make new glucose or pyruvate, while the fatty acids can be used to make Acetyl-CoA. Triacylglycerols are present in chylomicrons after the meal or the liver releases very low-density lipoproteins containing triglycerides. Lipoprotein lipase can cleave off fatty acids from triacylglycerols. Single fatty acids are taken up into the cells. In the fasted state, the lipids come from adipose tissue and get degraded by adipose triglyceride lipase and hormone sensitive lipase. Free fatty acids from adipose tissue travel through the blood bound to albumin and are inserted as free fatty acids into the cells. Fatty acids are esterified into fatty acyl CoA, which costs 2 ATP (ATP->AMP) and proceed in the beta oxidation. Carnitine Acyl Transferase: carnitine facilitates fatty acid transport from the cytosol into the mitochondrion. Fatty acyl CoA is linked to Carnitine Acyl Transferase (CAT/CPT) 1, which replaces the CoA group with carnitine. Fatty acyl carnitine is then transported into the matrix through the 2 membranes via 2 transporters. There, CAT2 swaps the carnitine for CoA again and fatty acyl CoA is in the matrix. Carnitine is then recycled and brought back into the cytosol by the same transporters. CPT 1 + CPT2: carnitine transferases. Beta oxidation: In the mitochondria and peroxisomes. Fatty acids are oxidized into acetyl-CoA. In every one-way cycle, FADH and NADH is released from metabolizing fatty acyl CoA. 2 carbons are removed in every cycle and oxidation starts at the beta- carbon of the fatty acid (2nd position from COOH end). There’s a difference between start and end point and there is no carrier. Every cycle, 2C are cleaved as Acetyl-CoA (10ATP), 1 FADH (1.5ATP) + 1 NADH (2.5 ATP) and (n-2) Fatty acyl CoA are the left. The rest of the fatty acyl CoA re-enters the cycle. So, per cycle, 14 ATP is gained. Depending on the carbon length of the fatty acid, there are different enzymes. Acetyl CoA can then be oxidized in the TCA cycle or can be used to produce ketone bodies. In mitochondria energy is released during the last steps to make acetyl CoA, while in the peroxisomes, no energy is gained so >18C gains as much energy as =18C (max length for mitochondrial beta oxidation). This energy contributes to ATP synthesis. The maximal chain length for importation into the mitochondria is not known exactly, possibly 22C. Unclear what the max length for mitochondria is. Fatty acyl CoA >16C: Can enter the peroxisomes first. Does not generate ATP, but does generate Acetyl-CoA. Fatty acids >C16 can enter peroxisomes and are digested to C16/C18 and then go to the mitochondria where they are digested in steps of 2Cs to acetyl CoA. Not all FA >16C go into peroxisomes first. Fatty acyl CoA Acyl carrier protein 3. Acyll carrier protein + Fatty acyl ACP -> Keto-acyl ACP 4. Keto-acyl ACP + NADPH-> hydroxy-acyl ACP: NADPH is invested, NADP remains. Fatty acid synthase 5. Hydroxy-acyl ACP -> enoyl ACP + H2O: water is released. Fatty acid synthase 6. Enoyl ACP + NADPH -> fatty acyl ACP: saturated. This fatty acyl is then used in step 3 to add to the new acetyl CoA’s that come in. Fatty acid synthase 7. After the fatty acid reaches C16, it is released into the cytosol and can be trapped into triglycerides. Triglyceride formation: glucose has an 82% efficacy of making body fat. 1. Fatty acids are labelled and turned into fatty acyl CoA (2 ATP). 2. Glycerol phosphate + Fatty acyl CoA – monocylglycerol phosphate: glycerol phosphate is synthesized from DHAP, a glycolysis product. 3. 2 Fatty acid CoA’s are added to form triacylglycerol 27 Lecture 12 (NP10a): Carb and amino acid metabolism, ketogenesis + exam questions Fructose: is a 6-carbon sugar, but has only 4 carbons in the ring, instead of five as in glucose. In a low dose, the small intestine clears most fructose, this is released in the circulation as glucose. In a high dose, there is a spill over and not all of the fructose can be taken up in the small intestine, therefore the microbiota get the chance to convert them into short chain fatty acids and some goes into the circulation as fructose to the liver (in mice, not yet checked in humans). Metabolism: KHK uses 1 ATP to phosphorylate fructose to fructose-1-P. This is then split into glyceraldehyde and DHAP. Glyceraldehyde is labelled with a phosphate using ATP. These two fructose products are intermediates of glycolysis. They then enter glycolysis. This in total uses 2 ATP. The glucose metabolism uses exactly the same energy to produce those 2 products. In fructose metabolism, you skip the rate limiting step of PFK, so they escape the regulation of the flux towards pyruvate. Breakdown of fructose will not stop when the body is fed, because PFK in glycolysis is inhibited, but the enzymes of fructose are all still active. This is why people think high fructose intake is linked to higher fatty acid synthesis. Galactose: the only difference between glucose and galactose is that the OH faces up on position 4 in galactose. Galactose is phosphorylated using 1 ATP. UDP-glucose is used and swaps the UDP with the phosphate. Glucose-1-phosphate proceeds in glycolysis and UDP galactose can be isomerized back into UDP-glucose. UDP is normally used in glycogen synthesis. The same energy is used for galactose as for glucose full oxidation. Glucose, fructose and galactose: all use 2 ATP and have DHAP and GA3P as end products. Degradation of glycerol: glycerol is the triglyceride backbone. When fats are turned into fatty acids, glycerol is burned. Glycerol is phosphorylated, using ATP. Then cytosolic NADH is gained when that is converted into dihydroxy-acetone-phosphate (DHAP). This process yields (1.5 -1 =) 0.5 ATP. DHAP is a glycolysis intermediate that can also be used for gluconeogenesis with use of triose phosphate isomerase (2 DHAP -> fructose 1,5-bisphosphate). Close link between glucose metabolism and fat metabolism. DHAP is converted into GA3P for complete oxidation, which gains another 16 ATP (2 ATP, 1 cytosolic NADH and 1 mitochondrial NADH + TCA: 10 ATP), so in total complete oxidation of glycerol gains 16.5 ATP. Glycerophosphate shuttle: NADH trapping in mitochondrial membrane. DHAP is used to trap NADH into glycerol-3-phosphate, which donates energy onto the complexes of oxidative phosphorylation, leading to 1.5 ATP per 1 mol of NADH synthesis. Mitochondrial FADH2 is made from cytosolic NADH. 28 Liver glycogen or glucose in circulation to muscle energy: you lose 1 ATP when you trap G1P to G6P, so both from liver glycogen and from glucose in the circulation. If glucose from the food is first stored as glycogen and then broken down to glucose again for the muscles, that loses -2 ATP more. Muscle glycogen to muscle energy: no ATP is lost. Muscles can’t turn glycogen into glucose, so they can’t release muscle glucose into the circulation, but they can only use it in the muscles. Muscle energy to other organ energy: Lactate can be released into the circulation to be reused via the Cori cycle. It can also be done by lactate that enters the colon and is then converted by the microbiota to propionate, which can be used in the TCA. Glucogenic amino acids: are converted into intermediates of the TCA cycle or glycolysis Ketogenic amino acids: can’t be converted into intermediates of the TCA cycle or glycolysis as glucogenic amino acids can. Leucine and lysine are the only completely ketogenic amino acids, they only lead to the production of Acetyl-CoA, which can’t be used for glucose synthesis because it is fully oxidized in the TCA. Linked to ketone bodies. Ketogenic + glucogenic: Some amino acids can both be ketogenic or glucogenic, phenylalanine, tryptophan, isoleucine and tyrosine. These can make acetyl-CoA or acetoacetate, which is a ketone body. Acetoacetate will not be considered in the exam when amino acid full oxidation is questioned. Only use the acetyl- CoA route! Urea cycle (oxidative deamination): splitting off the amino group, release of NADH Hepatic Ketogenesis: synthesis of ketone bodies, starting from 2 Acetyl-CoA. Only in the liver the acetyl-CoA can also serve as a substrate for ketone bodies in a low glucose state. Primarily derived from fatty acid beta oxidation. The brain relies on glucose. When fasting for long periods or adhering to a ketogenic diet, you switch to use of ketone bodies. When glucose drops, ketones are needed for energy for other organs as glucose is spared for the brain. Fats are broken down to fatty acids, that becomes fatty acyl coA, becomes acetyl-CoA and that goes into ketogenesis. 2 Acetyl CoA become acetoacetyl CoA, converted into HMG CoA. Three Acetyl-CoAs are combined and then one is released to make acetoacetate. In the release, one proton is also released, causing acidification of the blood. The end product is acetoacetate, which can become acetone or beta-hydroxybutyrate. Ketogenesis is very energetically favorable. The liver will not use it itself, but only release it in the circulation. Acetone: releases 1 CO2, non-enzymatic conversion, this is exhaled or excreted via urine, a loss of energy. The alternative is using NADH to trap the ketone body as beta- hydroxybutyrate. Some of the protons are trapped in the conversion to acetone. Beta-hydroxybutyrate: Trapped by 1 NADH, good energy source for organs. Breakdown of ketone bodies for energy: not in the map. Only in extra-hepatic tissues. They are released into the circulation. Especially muscle uses these ketone bodies, to survive in long term fasting situations. The liver has a huge capacity for beta oxidation (10x more than the muscle), providing a lot of Acetyl-CoA. Only if the glucose levels really get extremely low, the brain can also switch to ketone bodies. Energy generation from ketone bodies is done by reversed ketogenesis, leading to Acetyl-CoA production. Acetoacetate succinyl-CoA transferase is lacking in liver cells, therefore the liver itself can’t use ketone bodies for energy, but other organs can. The breakdown of beta-hydroxybutyrate regains the 1 NADH (liver invests, muscle gains), so that is the most energy rich ketone body. While acetoacetate is converted into acetoacetyl CoA, simultaneously succinyl CoA is converted into succinate (TCA intermediate, normally that generates GTP, but now that energy is put into the reaction). Acetoacetyl CoA is converted to 2 Acetyl-CoA -> 2x 20ATP 29 Amino acid catabolism Difference in ATP yield after complete oxidation: every intermediate always has to go through acetyl CoA for complete oxidation. You don’t want to make the end product oxaloacetate, because then you just have more carrier and not more energy. Instead, you shuttle malate out of the mitochondria, make oxaloacetate -> PEP -> pyruvate -> Acetyl-CoA -> 1 TCA cycle. Deamination gains 1 NADH, which is equal for all amino acids. Glutamate versus Aspartate: aspartate is converted to oxaloacetate, which needs to be converted back into malate first, costing 1 NADH (-2.5ATP). From glutamate (-> ketoglutarate) to malate you gain 1 NADH, 1 GTP and 1 FADH (5ATP). The difference is 7.5 ATP. Then both go through the cycle via shutteling malate out of the mitochondria and making acetyl-CoA. Aspartate can also be converted to fumarate, but we assume that it becomes oxaloacetate in this course. Glutamate - Alanine: alanine is converted into pyruvate, which only gains 1 NADH to make acetyl- CoA and then goes through 1 TCA cycle -> 12.5 ATP in total. Glutamate becomes ketoglutarate, which is an intermediate of the TCA cycle. It gains 2 mitochondrial NADH, 1 cytoplasmic NADH, 1 FADH and (1-1+1=) 1 ATP and then goes through the TCA cycle. So that is 19 ATP in total. The difference is 6.5 ATP. Actually, you should include de-amination (+2.5ATP) and the urea cycle (-2 ATP/N), so it is actually 19.5 and 13 ATP. When you look at the pathway from ketoglutarate to pyruvate, you also see that 6.5 ATP is gained. Dietary energy Is all dietary energy convertible to body fat: no, there are losses in all steps, such as heat. The energetic efficiency depends on the macronutrient that you are using. Lipids are very efficient in being turned into body fat (96%), glucose is 82% efficient and only 66% of proteins is stored as body fat, the rest is released at heat. Mitochondrial NADH: provides 2.5 ATP by oxidative phosphorylation in the mitochondria. Cytoplasmic NADH: provides 1.5 ATP by oxidative phosphorylation in the cytoplasm because it is converted into FADH2 -> 1.5ATP. Substrate level phosphorylation: convert a substrate into a product with a direct release of ATP. Substrate cycle: A -> B -> A. Always needs ATP investment. When you go from glucose to fructose 1,6-bisphosphate and back, 2 ATP are lost in glycolysis, and no ATP are gained back from gluconeogenesis. 30 Lecture 13 (NP11): Efficiency of fat synthesis What is needed to synthesize 1 mole of TG stearate (C18:0)? Calculate the input as mole glucose. Use this amount for estimation of efficiency. Try to calculate the amount of glucose ONLY as input to end up with 1 mole of TG stearate (fat). GE stearate: 11.347 kJ/mol GE glycerol: 1661 kJ/mol - Fatty acids: Citrate from the TCA cycle is shuttled out of the mitochondria and used for fatty acid synthesis. 1 glucose -> 2 Acetyl-CoA. 9 acetyl CoA in the 18:0 FA, so 4.5 mole glucose to make one 18:0 fatty acid. To make glycerol you need 3 fatty acids and 1 glycerol. To make a fat from 3x 18:0 fatty acids, you need 13.5 mole of glucose. - Glycerol: For the glycerol you need 1 DHAP (0.5 mol glucose). So in total you need 14 mole of glucose. Then you need to obtain the NADPH. - NADPH (PPP): 2 NADPH is needed per 2C addition, which is 8 times if you have an 18 FA at the end, 1 NADPH is gained every time you shuttle Acetyl-CoA into the cytoplasm (50%), so per 2C addition, you’ll need to get only 1 NADPH (per cycle). The first acetyl-CoA, where all other 2C’s are added to, does not go through the cycle (doesn’t use NADPH) but does gain one when it is shuttled out, therefore you need 8-1=7 NADPH in total per 18:0 FA (7x3=21). NADPH is gained from the pentose phosphate pathway. Per 3 mole glucose-6-phosphate, you obtain 6 NADPH, 2 fructose-6-phosphate and 1 glyceraldehyde 3-phosphate (glycolysis intermediates). So when you go through the PPP, you gain 6 NADPH and 5 Acetyl-CoA instead of 6 per 3 glucose. In total you need 27 acetyl-CoA, so if you do this you need 12 moles of glucose to make 24 NADPH and generate 20 Acetyl-CoA. Then for the remaining 7 acetyl-CoA you can just skip the PPP and then you need 3.5 mole of glucose, so in total you’ll need 15.5 moles of glucose + 0.5 moles for glycerol = 16 moles of glucose. - ATP: enough ATP is produced as byproduct, but keep in mind the -2ATP per esterification on fatty acids. So -6 ATP in total. GE of 16 mol of glucose: 2,816 x 16 GE of 1 mol fat: 3 x 11,347 (fatty acids) + 1.661 (glycerol) = 35,702 Efficiency = GE fat/GE of glucose = 79%. 31 Digestion of fat: Lipases break down triacylglycerols into diacylglycerol -> monoacylglycerol -> glycerol + 3 fatty acids. This does not involve any energy. This happens in the lumen of the intestine or intracellular, in the adipocytes for instance. These lipases are adipose triglycerides lipases or hormone sensitive lipases. These two are inhibited by insulin. When glucose is ingested, insulin levels rise and this is when lipolysis has to be stopped, because you are in the fed state and don’t need to break down your fat. When diabetes patients inject insulin on the same spot, they develop fat there. Hypoglycin: compound in Jamaican fruit and Chinese mushroom that blocks beta-oxidation, so you are unable to use fatty acid beta oxidation to provide energy. It is much more difficult to switch to glucose and amino acid break down. It also blocks gluconeogenesis in the liver. Causes heart failure, because the heart is fueled mainly by beta-oxidation. Why is anaerobic metabolism only possible for glucose?: In the electron transport chain you need oxygen to generate ATP. To make the other macronutrients you need oxygen because you need ATP. Glucose can be turned into lactate without oxygen, fatty acids and amino acids can’t. Ethanol: 29 kJ/gram. Two dehydrogenases release NADH, then acetate remains, a 2-carbon fatty acid. When you invest 2 ATP, this can be used in the TCA cycle as Acetyl-CoA. East-asians lack aldehyde dehydrogenase activity. When you take aspirin or paracetamol, this is also blocked. Fructose activates the enzyme. Lactate + O2 acetate + CO2 + H2O. Much more complicated. Interaction with reactive oxygen species. Not a direct interaction with O2. Energetic efficiency of trapping in ATP after complete oxidation of tri-stearate GE stearic acid: 11347 kJ (120ATP) GE Glycerol: 1661 kJ (16.5ATP) GE ATP: 33 kJ 3 x 11347 + 16661 = 35702 kJ in Fat 3 x (120 * 33) + 16.5*33 = 12424.5 trapped in ATP ATP/Fat = 35 % trapped in ATP, 65% is released as heat, used for body temperature. What is the difference in ATP-yield between palmitic (C16:0) and linoleic (C18:2) acid? 16C -> 8 Acetyl CoA, 18C -> 9 Acetyl CoA: 1 acetyl CoA more, so that is 1 extra cycle of beta-oxidation. In one beta-oxidation spiral 1 FADH and 1 NADH are released, giving 4 ATP. Also Acetyl-CoA is released, which generates 10 ATP in the TCA cycle so that is a difference of 14 ATP. The double bonds (18:2) generate 2 less FADH, 1 FADH per double bond because they skip the first step of beta oxidation where 1 FADH is gained, two double bonds equal -3ATP. So, in total the difference is 11 ATP. Short Chain Fatty Acids: generated by fermentation, a product of microbiota. Votile fatty acids. Acetate (C2 -> 1 acetyl-CoA), propionate (C3) and butyrate (C4 -> 2 acetyl-CoA). Used for energy. In the conversion of these fatty acids, ATP is converted into AMP (2ATP equivalent). Propionate is an odd numbered fatty acid, this is converted to a TCA cycle intermediate succinyl-CoA, so it is digested very differently. Propionate is important in herkauwers (ruminants) Energy content: C16:0 Work / Heat. Homeostasis: at a drop in body temperature, you start shivering. When you get a high body temperature you start sweating to release heat. Some heat is lost to the environment. Heat balance: Tb = constant. Heat gain = Heat loss. Can be at different levels of heat flow. ATP: Metabolism uses ADP + P to make ATP, this can be used for work, splitting ATP into ADP and P again, in that step heat is always lost. With a variable Eefficiency, energy is put into ATP, the Eefficiency of turning ATP into work is constant for all substrates, glucose, protein and fat in aerobic metabolism, 25%. (75% released as heat). It will constantly release heat, which balances body temperature. General reaction: substrate + O2 -> ATP + CO2 + H2O + By-product (lactate/NH3/Urea) + Metabolic Heat. These reagents can be limiting when absent. Mitochondrial work. Metabolic heat: heat produced during the metabolic steps of intermediates in metabolism. Glucose: C6H12O6 + 6 O2 -> 6 CO2 + 6 H2O + 2816 kJ/mol. 1 glucose gives 30 mol ATP (33kJ/mol), so that is 33*30 = 990 kJ trapped in ATP. Then the other 1826kJ is lost as heat (65%). 61 kJ of heat is released per ATP. O2/CO2 (RQ)=1. No by-product. Palmitic acid: C16:0. C16H32O2 + 23 O2 -> 16 CO2 + 16 H2O + 10033kJ/mol. 106 mol ATP -> 3498 kJ in ATP, the other 6535 kJ is released as heat (65%). So again 61 kJ of heat per ATP released, constant between different substrates. RQ=0.7. No by-product. Energy storage: The majority of the energy storage is in the fat mass, because fat is very high in calories. There is no water associated with triglycerides, therefore the energy per mass is higher. Aerobic metabolism: is highly regulated. You inhale oxygen via the lungs, that is then transported via the red blood cells with haemoglobin to for instance the muscles. Within the muscles, oxygen is used in the mitochondria in the oxidative phosphorylation, producing ATP, CO2, heat and H2O. Aerobic metabolism can be expressed as ATP/MJ, ATP/O2, CO2/O2 (RER, RQ), H2O/ATP or Heat/ATP. The ATP/L O2 is also similar between substrates. Energetic efficiency is similar between sugars, proteins and fats (35%). In aerobic metabolism, 30 ATP is gained. 25% energetic efficiency. 28 ATP, 93% of ATP is mitochondrial. More energy is gained, but the process is slower than anaerobic metabolism. Anaerobic metabolism: When you are sprinting, not enough oxygen is delivered in the muscle. Only glucose, not fatty acids or amino acids, can be oxidized anaerobically, via lactate, gaining 2 ATP per glucose. Lactate is secreted into the circulation. C6H12O6 -> 2 lactate (B) + 88 kJ/mol. 2 mole of ATP = 66 kJ trapped in ATP. 66/88kJ is 75% energetic efficiency, only 25% is released as heat. Very fast. Super-efficient and fast in producing energy in exercise, the lactate is then taken care of later. The mitochondria are not used because no oxygen is present. Less energy, but faster. Glycogen: This efficiency is even increased when glycogen stores in the muscle are used, because 1 ATP is used to trap glucose. Glycogen + H3PO4 -> G-1-P -> G-6-P -> 3 ATP. Glycogen -> 2 lactate + 111kJ/mol. Then, the energetic efficiency is 99 kJ/111kJ=89% efficiency. Only 4 kJ of heat/ATP is released. 33 Short-term effects: within minutes the lactate levels shoot up, and they gradually decline in an hour. The glucose levels stay high for a long time after exercise. Takes over an hour to recover. The body immediately switches to lactate when it is needed. Lactate that is built up, is used later for the cori cycle, where it is turned back into glucose. Veilonella: identified gut bacteria that was related to elite athlete performance. This bacterium increased during training for the sport and enhances performance. When they put it into mice and let them run on the treadmill, they had longer endurance. When they labelled the lactate, they saw that it went from the circulation into the lumen and the other way around. It functions via the lactate metabolism. Microbiota can convert lactate into acetyl-CoA or propionate (higher ratio, short chain fatty acid). Infusing propionate into the colon increases endurance as well. Propionate increases performance and is a TCA intermediate. Mountain climbing: hypobaric condition, low oxygen availability. The body shifts to anaerobic metabolism, increasing lactate production. Then the cori cycle is used for gluconeogenesis. More ATP is needed to convert lactate to glucose. The energy expenditure goes up, therefore oxygen consumption should go up. This is a severe problem, you can’t continue in that condition for a long time. Lactate has to be cleared immediately when produced. Lactate itself decreases the activity of the enzyme that converts pyruvate into lactate, therefore lactate has to go out of the cell into