Dang et al. (2021) PDF - Meaning in Life & Heart Rate Variability
Document Details
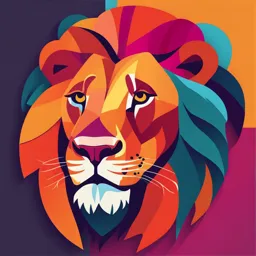
Uploaded by ModernHeliotrope
York University
2021
Kevin Dang, Megan A. Kirk, Georges Monette, Joel Katz, Paul Ritvo
Tags
Summary
This article investigates the relationship between meaning in life (MIL) and vagally-mediated heart rate variability (VmHRV). It explores how MIL might influence physiological responses to stress and mindfulness. The study examines resting, stress, and mindfulness conditions.
Full Transcript
International Journal of Psychophysiology 165 (2021) 101–111 Contents lists available at ScienceDirect International Journal of Psychophysiology journal homepage: www.elsevier.com/locate/ijpsycho Meaning in life and vagally-mediated heart rate variability: Evidence of a quadratic relationship at bas...
International Journal of Psychophysiology 165 (2021) 101–111 Contents lists available at ScienceDirect International Journal of Psychophysiology journal homepage: www.elsevier.com/locate/ijpsycho Meaning in life and vagally-mediated heart rate variability: Evidence of a quadratic relationship at baseline and vagal reactivity differences Kevin Dang a, Megan A. Kirk a, Georges Monette b, Joel Katz a, c, Paul Ritvo a, c, * a School of Kinesiology and Health Science, York University, Toronto, Ontario, Canada Department of Mathematics and Statistics, York University, Toronto, Ontario, Canada c Department of Psychology, York University, Toronto, Ontario, Canada b A R T I C L E I N F O A B S T R A C T Keywords: Meaning in life Eudaimonia Heart rate variability Cardiac vagal reactivity Cardiac vagal tone Stress Higher meaning in life (MIL) consistently predicts better health, but the physiological processes underlying this relationship are not well understood. This study examined the relationship between MIL and vagally-mediated heart rate variability (VmHRV) under resting (N = 77), stressor (n = 73), and mindfulness intervention (n = 72) conditions. Regression was used for MIL-VmHRV analyses at baseline, and longitudinal mixed models were used to examine phasic changes in VmHRV as a function of MIL. Regression revealed a quadratic MIL-VmHRV relationship, and mixed models linked higher MIL to greater stress-reactivity but not enhanced stressattenuation. MIL and mindfulness did not interact to influence VmHRV recovery after experimental stress. Findings suggest that cardiac vagal tone and cardiac vagal reactivity are linked to MIL, shedding light on the physiology underlying MIL and its health associations. 1. Introduction 1.1. Meaning in life and health Definitions of Meaning in Life (MIL) share three common character istics: coherence, purpose, and significance (King and Hicks, 2021). Coherence is observed when people comprehend and make sense of informative patterns. Purpose is associated with systematically-derived goals and directions, and significance is associated with perceiving ac tions as consequential and life as valuable (George and Park, 2016; Martela and Steger, 2016). Deficits in these characteristics (i.e., a life low in meaning) are hypothesized to result from inabilities to see value in life, resulting in boredom, indifferent decision-making, and low expen ditures of effort (Maddi, 1967). The emphasis on studying MIL (Steger et al., 2006) is reinforced by links between high levels of self-reported meaning, positive mental and physical health status (Czekierda et al., 2017; Heisel et al., 2016; Roepke et al., 2013; Steger et al., 2008), and healthier biomarker levels (Friedman et al., 2007; Zilioli et al., 2015). Despite research on MIL and health indicators, there is insufficient data on how MIL manifests physiologically, especially in terms of its predictive relationship with stress-reactivity and recovery. Thus our study examined MIL in relation to a key physiological variable, vagally-mediated heart rate variability (VmHRV), under resting, artificial stressor, and stress reduction conditions. 1.2. Heart rate variability and health Heart rate variability measures functional balance in the autonomic nervous system (ANS), and VmHRV reflects variability in inter-beat in tervals, providing a measure of parasympathetic activation (Appelhans and Luecken, 2006). Higher VmHRV is indicative of cardiac health, reflecting adaptive and efficient ANS responses to external stimuli (Acharya et al., 2006; Thayer et al., 2010). By contrast, low VmHRV signals a pattern of autonomic inflexibility and dysregulation linked to impaired stress reactivity (Brosschot et al., 2007; Weber et al., 2010), distress (Beauchaine and Thayer, 2015), and disease risk (Task Force, 1996). 1.3. Meaning and social health Polyvagal theory links higher VmHRV (cardiac vagal control) to positive social functioning, emphasizing vagus nerve activity in an in tegrated neural circuit consisting of somatomotor (striated muscles of * Corresponding author at: School of Kinesiology and Health Science, York University, 4700 Keele Street, Toronto, ON M3J 1P3, Canada. E-mail address: [email protected] (P. Ritvo). https://doi.org/10.1016/j.ijpsycho.2021.03.001 Received 17 November 2020; Received in revised form 3 March 2021; Accepted 4 March 2021 Available online 18 March 2021 0167-8760/© 2021 Published by Elsevier B.V. K. Dang et al. International Journal of Psychophysiology 165 (2021) 101–111 head and face) and autonomic components that support or impede social bonding (Porges, 2011, 2007, 2001). Threatening facial expressions, for example, trigger defensive states incompatible with social engagement, while vagally regulated autonomic states support calmer expressions and prosociability (Porges, 2018, 2003). Vagal regulation appears to contribute to adaptive social behaviors (Porges and Furman, 2011), a finding supported in multiple studies linking higher cardiac vagal tone with greater social competence (Beauchaine, 2001), empathy (Fabes et al., 1994; Stellar et al., 2015), and connectedness (Kok and Fre drickson, 2010). Therefore, a possible pathway between meaning and physical health likely involves social relationships, as close relationships are a central source of MIL and support all three MIL characteristics (Debats, 1999; King and Hicks, 2021). Moreover, MIL and social connectedness likely interact in cycles where close relationships elevate MIL and elevated MIL promotes social bonding (Stavrova and Luhmann, 2016). For example, higher MIL is linked to interpersonal appeal and relationship initiation (Stillman and Lambert, 2013), and is longitudi nally predictive of marriage, community engagement, and social connectedness (Stavrova and Luhmann, 2016). Individuals with higher MIL thus have greater access to the stress-buffering benefits of positive relationships (Cohen, 2004; Umberson and Karas Montez, 2010), sup porting VmHRV’s relevance as a potentially important MIL marker. perceptions (Kim et al., 2019), individual differences in MIL should manifest in cardiac vagal control and VmHRV measures. The present study examines whether higher vs. lower MIL uniquely predicts cardiac vagal control under resting, stress, and recovery con ditions while controlling for depression symptoms1 and positive affect, potential mood confounders known to correlate with both MIL (King et al., 2006; Steger et al., 2006) and VmHRV (Kemp et al., 2010; Oveis et al., 2009). Hypothesis 1. Greater MIL will be associated with greater levels of VmHRV during resting baseline. Hypothesis 2. Higher MIL will relate to (a) less stress reactivity (smaller VmHRV reductions) in response to an artificial stressor and (b) faster stress attenuation (more cardiac vagal input) as the artificial stressor progresses. Hypothesis 3. Stress-reducing influences of mindfulness meditation (MM; Azam et al., 2016, 2015; Burg et al., 2012; Ditto et al., 2006; Krygier et al., 2013; Wu and Lo, 2008) will accelerate VmHRV recovery at different rates depending on MIL level. Because MM requires the exercising of executive control (Gallant, 2016; Jankowski and Holas, 2014; Teper et al., 2013) and because MIL is positively linked to exec utive functioning (Lewis et al., 2017), an “active” MM condition will facilitate faster VmHRV recovery in individuals with higher MIL compared to a “control” MM condition devoid of active components. 1.4. Neurovisceral integration model and stress-regulation According to the neurovisceral integration model, VmHRV is linked to self-regulation of the central autonomic network (CAN; Smith et al., 2017; Thayer and Lane, 2000, 2009) where prefrontal cortical (PFC) regions inhibit subcortical regions (e.g., amygdala), critical for adaptive cognitive-emotional stress responses (Heatherton and Wagner, 2011; Sakaki et al., 2016). Because CAN output reaches the heart via sympathetic-parasympathetic innervation, VmHRV reflects functional connectivity between PFC and subcortical regions (Smith et al., 2017; Thayer and Lane, 2000). Supporting these assumptions is a large evi dence base linking higher VmHRV to higher executive functioning (Thayer et al., 2009), better regulated emotive responding (Appelhans and Luecken, 2006), and neuroimaging indications of greater PFC ac tivity (Thayer et al., 2012). 2. Method 2.1. Participants Study participants were between the ages of 17–27 years. Partici pants were recruited through an undergraduate research pool that grants university course credit for participation. Exclusion criteria included diabetes, cardiovascular diseases (arrhythmias and hyperten sion), and psychiatric illnesses (e.g., anxiety, depressive, and eating disorders), as their association with VmHRV is well-documented. This study was approved by the Human Participants Review Committee at York University. 2.1.1. Sample size estimation Based on an a-priori power analysis (G*Power 3.1) and a randomized control trial design for testing MM’s effect on VmHRV, a sample of N = 52 was estimated to provide adequate power (1 − β =.80) for detecting a medium (Burg et al., 2012) to large (Krygier et al., 2013) effect (f2 = 0.20) based on α =.05. We further assumed a 20% drop-out rate, and estimated that N = 65 would be an appropriate sample size. Stoppage of data collection was informed by this sample estimation and determined by COVID-19 pandemic restrictions. 1.5. Meaning and stress-regulation Existing data suggest higher MIL buffers adverse life stress (Kim et al., 2019), leading to assertions that when people face uncontrollable stressors, coherence can improve self-regulation by enhancing the comprehension of stressors that would otherwise appear random (Hooker et al., 2018; Park and Baumeister, 2017). Additionally, enhanced experiences of life purpose appear to attenuate stress-reactivity by providing a framework where goals and associated cognitions are coherently organized, facilitating adaptive prioritizing of daily stressors (Halama, 2014; McKnight and Kashdan, 2009). Several studies support linkages between greater life purpose and quicker emotional recoveries (Schaefer et al., 2013), reduced activation of the amygdala (a region linked to fear and anxiety-related emotional responses), more ventral anterior cingulate cortical activity (linked to amygdala down-regulation; Van Reekum et al., 2007), and better ex ecutive functioning (critical for self-regulation; Hofmann et al., 2012; Lewis et al., 2017). Conversely, induced meaninglessness appears to increase stress perceptions during stress induction protocols (Park and Baumeister, 2017). If MIL indeed attenuates stress-reactivity and stress 2.2. Measures 2.2.1. Vagally mediated heart rate variability VmHRV was acquired using a 3-lead electrocardiogram (ECG) configuration attached to both wrists and the left ankle. ECG signals were digitized using a PowerLab 4/35 acquisition system (ADInstru ments, Colorado Springs, United States) at a sampling rate of 1000 Hz. Digitized signals were recorded and analyzed using LabChart 8 software. Labchart 8’s HRV 2.0 module was used for R wave detection and VmHRV analysis. Prior to analysis, all ECG recordings were visually inspected for artifacts, arrhythmias, and ectopic beats, and manually adjusted if beat markers were missing or misplaced. 1 We controlled for depression symptoms instead of negative affect because depression is known to be more strongly associated with MIL (Steger et al., 2009, p. 50; Steger and Kashdan, 2013, p. 107). 102 K. Dang et al. International Journal of Psychophysiology 165 (2021) 101–111 VmHRV was calculated from spectral analysis in the high frequency band (0.15–0.40 Hz [HF-HRV]) using the Lomb-Scargle Periodogram algorithm and from time domain analysis of the root mean square of successive heart beat differences (RMSSD). RMSSD and HF-HRV are strongly correlated (Laborde et al., 2017) and are preferred measures of VmHRV (Task Force, 1996). 5-min measurements of RMSSD and HFHRV were used for cross-sectional analyses (Hypothesis 1), and because RMSSD yields valid estimates even from ultra-brief recordings (e.g., 1 min; Esco and Flatt, 2014; Melo et al., 2018; Nussinovitch et al., 2011; Shaffer et al., 2016), RMSSD was used to assess longitudinal change (slopes and trajectories) on a minute-to-minute basis (Hypoth eses 2–3). reliability, and excellent concurrent validity (Radloff, 1977). 2.3. Pattern recognition task (PRT) A computer-administered pattern recognition task (PRT) designed to elicit a stress response (Azam et al., 2015) was used during the stress phase. The PRT consisted of 22 trials, and in each trial, 4 alphanumeric characters were presented for approximately 11 s during which partic ipants were expected to try to discern a pattern. Character sets were designed to plausibly resemble simple or complex patterns when in fact there were none. After approximately 11 s, a 5th character was pre sented and participants were expected to quickly but accurately deter mine if it was pattern-consistent with the first 4 characters. Participants pressed the left arrow key for “True” if they believed the character was pattern-consistent and the right arrow key for “False” if they believed it was not. Immediately after responding, feedback with the text “Right Answer” or “Wrong Answer” was displayed on the screen along with a running total of “Correct” and “Incorrect” responses. The PRT was programmed to display “Right Answer” and “Wrong Answer” 50% of the time in a pre-defined and random sequence that was identical across participants. Before beginning the PRT, each participant was told that task performance reflected cognitive processing capacity and that the average score was approximately 80% with an average response time of 2 s for each of the 22 trials. 2.2.2. Respiratory frequency There is ongoing debate about whether VmHRV should be adjusted for respiratory parameters. Some researchers argue that adjustment is needed to accurately assess VmHRV (Grossman and Taylor, 2007), whereas others argue that respiration and VmHRV share a common genetic-neural origin and that removal of respiratory-related variance inappropriately removes some of the influence from this common origin on heartbeat, in turn, eliminating important individual differences (for a detailed review see Thayer et al., 2011). Therefore, respiratory fre quency was included as a time-varying covariate to supplement primary findings. Respiration data were acquired using a TN1132/ST respiratory belt (ADInstruments, Colorado Springs, United States) positioned over the diaphragm at the area of maximum respiratory expansion. 2.4. Mindfulness meditation task 2.2.3. Meaning in life questionnaire (MLQ) The presence of meaning subscale (MLQ-P; Steger et al., 2006) was used to measure the degree of overall MIL perceived by participants at baseline. The MLQ avoids presupposing any one definition of meaning and instead, allows respondents to define “meaning” for themselves (Steger et al., 2006). Participants rated the MLQ-P’s five items using a 7point scale ranging from “strongly disagree” to “strongly agree”. The MLQ-P has demonstrated good internal consistency (Cronbach’s alpha =.82), test-retest reliability, and convergent and divergent validity (Steger et al., 2006). We used two treatment conditions: mindfulness meditation instruc tion (MMI) and mindfulness meditation description (MMD). MMI involved a 10 min recorded guided meditation emphasizing breath awareness and non-judgemental redirection of attention to breathing sensations after noticing a distracting thought or emotion. MMD, by contrast, involved a 10 min audio recording describing mindfulness concepts and related evidence without any instructional references. 2.5. Procedure Testing was conducted in a University laboratory. Each participant was assessed in a single session. Participants were instructed to avoid food (for 2 h), caffeine (for 3 h), and vigorous exercise (for 24 h) before their appointments. Upon arrival, participants provided written informed consent and completed electronically administered questionnaires. The experiment adopted a repeated measures design consisting of three consecutive phases: resting baseline, stress exposure, and treat ment. As the experiment was deemed useful for comparisons with another psychophysiological measure, pupil diameter, the experiment took place in a dimly lit room and incorporated use of a wearable eye tracker (Tobii Pro Glasses 2), a chin rest to optimize pupil measurement, and a fixation cross displayed on a computer screen to minimize eye movement. We were unable to analyze pupillometric data due to COVID-19 pandemic restrictions. The present report thus omits the an alytic details related to pupillometric data; analyses are planned for a future date. The baseline phase required participants to sit for 5 min in a relaxed and upright position while resting their chin in a chinrest and their gaze on a fixation cross. Participants were instructed to think freely while remaining silent and avoiding sudden movements. The stress phase employed the PRT. Immediately after the stress phase, participants were asked to complete a manipulation check measuring perceived stress, performance, and task difficulty. 2.2.4. Positive and negative affect schedule (PANAS) The positive affect subscale of the PANAS (PANAS-P; Watson et al., 1988) was used to assess positive affect at baseline and incorporated as a covariate in all primary analyses. The PANAS-P consists of 10 adjectives that describe positive affect (e.g., interested, enthusiastic, and strong). Participants rated the extent to which they felt each adjective during the past week using a 5-point Likert scale ranging from 1 (“very slightly or not at all”) to 5 (“extremely”). The PANAS has demonstrated internal consistency (Cronbach’s alphas =.86–.90), convergent validity, and discriminant validity (Watson et al., 1988). 2.2.5. Center for Epidemiologic Studies Depression Scale (CES-D) The CES-D (Radloff, 1977) was used to determine baseline depres sion symptoms and incorporated as a covariate in all primary analyses. The CES-D is a self-report questionnaire designed to study depressive symptoms in the general population. The questionnaire includes 20 questions and focuses on the affective components of depression (Radloff, 1977). Participants rated how often they felt each symptom during the past week. There are 4 response options scored on a 4-point scale: 0 = rarely or none of the time (less than 1 day), 1 = some or a little of the time (1–2 days), 3 = occasionally or a moderate amount of time (3–4 days), 4 = all the time (5–7 days). The CES-D has demonstrated internal consistency (Cronbach’s alpha =.90), acceptable test-retest 103 K. Dang et al. International Journal of Psychophysiology 165 (2021) 101–111 Participants were then randomly assigned to either the MMI condi tion or the MMD condition. In both conditions, participants sat upright while resting their chin in a chinrest and their gaze on a fixation cross. Upon experiment completion, participants were debriefed and informed about the nature of the PRT and the purpose of deception. Table 1. 3.1. Hypothesis 1: MLQ-P is positively correlated with RMSSD and HFHRV A hierarchical multiple regression was conducted to examine the relationship between MLQ-P and RMSSD. MLQ-P was median-centered to improve interpretability of parameter estimates. RMSSD was derived from the full 5-min duration of the baseline phase. Results are summarized in Table 2. As per model 1, there was no evidence of a linear relationship be tween MLQ-P and RMSSD, F(1, 75) = 0.27, p =.607; however, visual inspection of the data suggested a potential U-shaped relationship, which was supported by model 2, R2 =.10, ∆R2 =.09, F(2, 74) = 3.92, p =.024. The quadratic relationship remained significant after controlling for positive affect, depressive symptoms, and respiration (model 3), R2 =.23, ∆R2 =.13, F(5, 71) = 4.24, p =.002. This relationship is presented in Fig. 2. An effect-size sensitivity analysis (G*Power 3.1) for an increase in R2 from MLQ-P, given control variables, revealed a minimum detectable effect of f2MLQ-P|Covariates = 0.13 when n = 77, α =.05, and (1 − β) =.80. The corresponding observed effect size was f2MLQ-P|Covariates = 0.12. MLQ-P was linearly related to positive affect, r(77) =.44, p <.001; and depressive symptoms r(77) = −.48, p <.001. Results were similar when 5-min HF-HRV was regressed on the same variables. Table 3 presents the full model, R2 =.295, F(5, 71) = 5.95, p <.001. The observed effect size of MLQ-P given control variables was f2MLQ-P|Covariates = 0.08. The regression analysis therefore suggests that VmHRV parameters (RMSSD and HF-HRV) were relatively low at moderate levels of MLQ-P but tended to increase as MLQ-P increased or decreased even after controlling for important confounders. 2.6. Statistical analyses Prior to analyses, raw RMSSD and HF-HRV data were natural log transformed to adjust non-normality indicated by the Shapiro-Wilk test, ps <.001, and then rescaled by a multiple of 100; i.e., ln(RMSSD) * 100 and ln(HF-HRV) * 100.2 2.6.1. Hypothesis 1 Regression models were used to examine relationships between MLQ-P and 5-min VmHRV parameters at rest. 2.6.2. Hypothesis 2 Before testing Hypotheses 2 (and 3) using longitudinal mixed models, it was necessary to first model the expected minute-by-minute response trajectory of ln(RMSSD) * 100 across phases using a linear spline (a piecewise function comprised of linear pieces joined at “knot” points [Smith, 1979]). Knots were placed at each phase transition (5.0 and 10.0 min) to allow for possible discontinuous change (slope and level change) and at the end of the first half of treatment (15.0 min) to allow possible continuous change (slope change only). All spline pa rameters were allowed to vary randomly across individuals to capture differences in individual trajectories. Parameters were calculated using restricted maximum likelihood (REML) estimation. A second order autoregressive covariance structure [AR(2)] was fit to capture potential autocorrelation inherent in longitudinal data. To assess (a) stress-reactivity and (b) stress-attenuation as a function of MLQ-P, we tested the moderating effect of MLQ-P on ln(RMSSD) * 100 trajectories from (a) min 5.0–6.0 (final baseline minute to stress onset) and from (b) min 6.0–10.0 (stress task progression). 3.2. Hypothesis 2: MLQ-P is differentially associated with stress responding as measured by RMSSD 3.2.1. Step 1: modelling the expected RMSSD trajectory across 20 min To examine potential interactions between MIL and stressresponding across protocol phases, it was necessary to first model the expected response trajectory across the entire 20 min protocol (base model without moderating variables; e.g., MLQ-P). The expected tra jectory is plotted in Fig. 3 and its corresponding parameter coefficients, parameter variability, and significance levels are presented in Table 4. The intercept parameter denotes the mean RMSSD during the first baseline minute. Three types of change parameters related to RMSSD are described: (1) slope parameters describe the intra-phase rate of change, (2) “Jump” parameters describe abrupt level change related to phase transition (e.g., from baseline to stress onset), and (3) relative slope change parameters describe the change in slope compared to the slope in the period just before. Altogether, the expected response trajectory is characterized by 2.6.3. Hypothesis 3 To examine the effect of MLQ-P and mindfulness on VmHRV re covery, we tested whether the interaction between MLQ-P and treat ment condition (MMI vs. MMD) moderated ln(RMSSD) * 100 trajectories during min 10.0–20.0 (treatment phase). Wald tests were used for joint significance testing involving multiple regression parameters. Analyses were carried out using R version 4.0.0 with nlme (Pinheiro et al., 2020) to perform mixed-effects modeling and spida2 (Monette et al., 2019) for spline modeling and Wald testing. The significance threshold across analyses was defined as p <.05. 3. Results Fig. 1 summarizes the flow of participants through experimental phases. Ninety participants (65 female; 25 male) were screened from October 2019 to February 2020. Thirteen participants were ineligible due to physical or mental health conditions (e.g., diabetes, cardiovas cular disease, anxiety disorder), resulting in 77 healthy participants at baseline. Four participants recognized deception in the stress task and were excluded from post-baseline analyses. One participant experienced a headache during the treatment phase and was excluded from poststress analyses. Four participants provided partial respiratory data due to respiratory belt malfunction. Sample characteristics are presented in ▪ a gradual RMSSD decrease during baseline, ▪ a marked RMSSD decrease at stress onset, ▪ positive slope change from baseline to stress, but no evidence of a significant slope during stress, ▪ a marked RMSSD increase at treatment onset, ▪ a gradual RMSSD decrease during the first half of treatment, and ▪ positive slope change from the first half of treatment to the second half, but no evidence of a significant slope during the second half of treatment. 2 Note that log differences of a variable reflect the approximate proportional change in that variable. Thus, in a regression where ln(RMSSD) * 100 is, for example, the dependent variable, coefficients of linear predictors are directly interpretable as the approximate percentage change in RMSSD associated with a unit change in the linear predictor. 3.2.2. Step 2: incorporating MLQ-P as a moderator, holding mood variables constant MLQ-P was then included in the base model as a moderating variable to assess its potential relationship with the expected trajectory – that is, 104 K. Dang et al. International Journal of Psychophysiology 165 (2021) 101–111 Screened for eligibility (n = 90) Physical or mental health condion (n = 13) Baseline (n = 77) Recognized decepon (n = 4) Stress (n = 73) Experienced headache (n = 1) Mindfulness Instrucon (n = 34) Mindfulness Descripon (n = 38) Fig. 1. Participant flow through experimental phases. whether trajectories varied as a function of MLQ-P. Given evidence of a quadratic relationship between MLQ-P and VmHRV, the quadratic polynomial of MLQ-P was used in the model. PANAS-P and CES-D were included as covariates. Instead, slopes appeared parallel and sustained across MIL levels as evidenced by slopes indistinguishable from zero with small SEs (see Appendix). This interaction remained non-significant after adjusting for respi ration, F(2, 1370) = 0.61, p =.941. 3.2.3. Hypothesis 2a: higher MLQ-P is associated with less stress-reactivity (smaller RMSSD reductions) at stress onset A Wald test was used to assess whether MLQ-P moderated the effect of stress on RMSSD with mood variables held constant. There was evi dence of a significant interaction between MLQ-P and stress onset (from min 5.0–6.0), F(2, 1383) = 5.35, p =.005. Stated differently, there was evidence of variability in RMSSD level change at stress onset as a function of MLQ-P. Fig. 4 illustrates the response variability expected from the final baseline minute (T5) to the first stress minute (T6) and Fig. 5 plots the difference between the two response curves (T6 - T5). Table 5 provides corresponding difference estimates for low, medium, and high values (10th, 25th, 50th, 75th, and 90th percentiles) of MLQ-P. Per Table 5, high MLQ-P levels were associated with greater level changes in RMSSD compared to moderate MLQ-P levels. By contrast, there was no evidence of level change in RMSSD at low MLQ-P levels. The evidence therefore suggests that at stress onset, higher MLQ-P was associated with larger reductions in RMSSD. This interaction remained significant after adjusting for respiration, F(2, 1370) = 4.07, p =.017, but in contrast to the results presented in Table 5, only the level change at the 90th percentile of MLQ-P was significant (estimate = − 12.39, SE = 5.87), t(1370) = − 2.11, p =.035. 3.3. Hypothesis 3: MMI (active treatment) facilitates faster recovery (more positive RMSSD trajectories) as a function of higher MLQ-P compared to MMD (control treatment) 3.3.1. Step 1: testing the moderating effect of treatment condition (MMI vs. MMD) First, we examined MMI’s effect on response trajectories (relative to MMD’s effect) by testing differences in level and slope changes. There was no evidence of significant differences related to treatment, F(3, 1384) = 0.64, p =.591. MMI therefore appeared neither more nor less effective in facilitating post-stress parasympathetic recovery compared to MMD. Results remained non-significant after entering respiration as a co variate, but p-values were substantially changed, F(3, 1371) = 1.84, p =.139; a detailed comparison is provided in Table 6. 3.3.2. Step 2: testing the moderating effect of MLQ-P x treatment condition Next, we examined whether an interaction between MLQ-P and treatment condition (MMI vs. MMD) moderated treatment response trajectories while controlling for mood variables (PANAS-P and CES-D). There was no evidence that trajectories varied as a function of MLQ-P and treatment condition, F(6, 1372) = 0.57, p =.755. Therefore, there was no evidence that MMI was more or less effective in facilitating poststress parasympathetic recovery at higher levels of MLQ-P compared to MMD. Results remained non-significant after entering respiration as a covariate, F(6, 1359) = 0.49, p =.817. 3.2.4. Hypothesis 2b: higher MLQ-P predicts faster stress attenuation (more positive RMSSD trajectories) during stress induction There was no evidence of variability in slope change (baseline vs. stress) as a function of MLQ-P, F(2, 1383) = 0.60, p =.551. Stated differently, different levels of MLQ-P were not differentially associated with parasympathetic control for the remainder of the stress task. 105 K. Dang et al. International Journal of Psychophysiology 165 (2021) 101–111 Table 1 Demographic, psychological, and physiological characteristics at baseline (N = 77). 450 n (%) / M (SD) Female Male Age Education level High school College University Race/ethnic origin African Caribbean Latin American West Asian South Asian Southeast Asian East Asian European/White Russian Multi-ethnic Psychological variables MLQ-P PANAS-P CES-D VmHRV (5-min) Raw HF-HRV (ms2) Raw RMSSD (ms) ln(HF-HRV) * 100 ln(RMSSD) * 100 Respiratory frequency (5-min; bpm) 53 24 19.95 (68.8%) (31.2%) (2.29) 75 1 1 (97.4%) (1.3%) (1.3%) 7 4 3 12 19 8 3 12 1 8 (9.1%) (5.2%) (3.9%) (15.6%) (24.7%) (10.4%) (3.9%) (15.6%) (1.3%) (10.4%) 23.18 32.66 18.35 Fitted Value + Residual Variable 10 (1769.01) (25.26) (112.10) (49.56) (3.93) Model 1 MLQ-P (linear) Model 2 MLQ-P MLQ-P (quadratic) Model 3 MLQ-P MLQ-P (quadratic) PANAS-P CES-D Respiratory frequency SE p 0.467 0.905.607 1.430 0.300 0.936 0.109.131.008 − 0.054 0.283 1.877 − 0.654 − 2.947 1.032 0.104 0.870 0.654 1.327.958.008.034.321.030 R2 ∆R2.00.00.10.09.23.13 20 25 30 35 Fig. 2. Visualization of relationship between ln(RMSSD) * 100 and meaning in life, adjusted for positive affect, depressive symptoms, and respiration (N = 77). Note. Component-plus-residual plot for MLQ-P in the regression of ln(RMSSD) * 100 on MLQ-P, adjusting for covariates CES-D, PANAS-P, and respiratory fre quency. Fitted values are predicted values of ln(RMSSD) * 100 when covariates are held constant at their mean value; residuals are from the regression. (For a review of component-plus-residual plots, see Cook, 1993; Wood, 1973.) RMSSD = root mean square of successive differences between normal heartbeats; MLQP = Meaning in Life Questionnaire - Presence of meaning subscale; PANAS-P = Positive and Negative Affect Schedule – Positive affect; CES-D = Center for Epidemiologic Studies Depression Scale. Table 3 Multiple regression analysis predicting HF-HRVa from meaning in life, positive affect, depressive symptoms, and respiration (N = 77). Variable Table 2 Hierarchical regression analysis predicting RMSSDa from meaning in life, posi tive affect, depressive symptoms, and respiration (N = 77). b 15 MLQ−P Note. Categorical variables reported as frequencies and percentages; numeric variables reported as Ms and SDs. MLQ-P = Meaning in Life Questionnaire Presence of meaning subscale; PANAS-P = Positive and Negative Affect Schedule – Positive affect; CES-D = Center for Epidemiologic Studies Depression Scale; VmHRV = vagally-mediated heart rate variability; HF-HRV = high frequency heart rate variability; RMSSD = root mean square of successive differences be tween normal heartbeats. Variable 350 300 (6.31) (6.71) (9.22) 1230.00 44.57 647.84 366.74 16.64 400 MLQ-P MLQ-P (quadratic) PANAS-P CES-D Respiratory frequency b SE p 0.864 0.537 3.164 − 1.058 − 11.471 2.234 0.225 1.882 1.416 2.871.700.020.097.458