Chemical Bonding Notes (revsd) PDF
Document Details
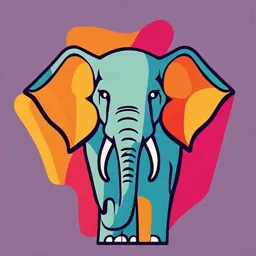
Uploaded by DignifiedBanshee
MKSSS's Cummins College of Engineering for Women
Tags
Summary
These notes cover chemical bonding, including ionic, covalent, and metallic bonding, and van der Waals forces. They define key concepts and provide examples.
Full Transcript
20BS02 Chemistry Unit I -Chemical Bonding Notes by Chemistry Staff, CCOEW Valency of an element Valence is the number of bonds formed by an atom in a molecule. Valence electrons: The electrons...
20BS02 Chemistry Unit I -Chemical Bonding Notes by Chemistry Staff, CCOEW Valency of an element Valence is the number of bonds formed by an atom in a molecule. Valence electrons: The electrons in the outer energy level (i.e. valence shell) of an atom are the ones that take part in chemical bonding, and are referred as valence electrons. e.g. Sodium has electronic configuration as 2,8,1. and it forms only one chemical bond in its compounds hence its valence is one. While Chlorine has electronic configuration as 2,8,7. and it forms only one chemical bond in its compounds hence its valence is also one. Atoms with 8 electrons (or 2 electrons if only one orbit is there) in their valence shell are stable. Other atoms which do not have 8 electrons (or 2 electrons if only one orbit is there) in their valence shell, they try to attain the octate (or duplate if only one orbit is there) either by transferring some electrons or by sharing them and form chemical bonds. QB Chemical Bond: A chemical bond is defined as a force that acts between two or more atoms to hold them together so as to get a stable molecule (lower energy system). Based on mode of formation, chemical bonds can be classified as: I. Ionic or Electrovalent Bond. II. Covalent Bond. III. Coordinate covalent Bond. IV. Metallic Bond. V. van der Waals forces. (forces of attractions on molecular level) I. Ionic or Electrovalent Bond. QB Definition: The bond formed by transfer of an electron from one atom to another atom is called as Ionic or Electrovalent bond.e.g. NaCl, MgCl2, CaO. Example: Sodium Chloride is formed from an atom of sodium and one atom of chlorine, Na (2,8,1) has one valence electron while Cl(2,8,7) has seven valence electrons, Na transfers its one valence electron to Cl and both achieve noble gas configuration i.e. stable electron octate. Thus Na gives Na+ and Cl gives Cl- ion. * Reference: Essentials of Physical Chemistry by Arun Bahl, B.S. Bahl and G.D. Tuli Page 1 QB Factors Governing formation of Ionic Bond: 1. Ionisation Energy : Ionisation energy of the metal atom, which loses electron should be low so that the formation of positively charged ion is easier. Lower the ionisation energy, greater is the tendency of a metal atom to change into cation and hence greater will be the ease of formation of ionic bond, thus alkali metals and alkaline earth metals forms ionic bonds easily. 2. Electron Affinity: The atom which accepts an electron and changes into an anion should have high electron affinity. Higher the electron affinity more is the energy released and stable will be the anion formed. Halogens and Gr VI A Elements tend to form Ionic bonds. ( less energy remains in the system, more stability) 3. Electronegativity difference of atoms involved: Electronegativity difference between atoms involved should be more than 2 only then they will form ionic bond. e.g Na has electronegativity 0.9 while Cl has 3.0, so the difference is 2.1 hence Na and Cl will form an Ionic compound. Characteristics of Ionic Compounds: 1. They are solids at room temperature. 2. They have high melting and boiling points. 3. They are hard and brittle 4. They are soluble in water. 5. They are conductors of electricity in molten and in solution state. 6. Reactions involving ionic compounds are fast. 7. Ionic bonds involve electrostatic lines of force between oppositely charged ions, it is not rigid and is non directional therefore ionic compounds are incapable of exhibiting isomerism. Page 2 II. Covalent Bond. Defination: The attractive force between atoms, created by sharing of an electron pair is called as a covalent bond and the compouds containing covalent bond are called covalent compounds. Electronegativity difference of atoms involved should be less than 2 to form a covalent bond. Based on Electronegativity difference covalent bond can be categorised into two types. 1. Non polar covalent Bond A. If the electronegativity difference is 0 i.e. two atoms of same element are involved in bond formation and bond thus formed is called Non polar covalent bond. B. In such case bonded electrons are equally shared between two similar atoms. e.g. H2, Cl2, N2, F2, O2. QB 2. Polar covalent Bond A. If the electronegativity difference is between 0.1 to 2.0 i.e. two atoms of different elements are involved in bond formation and bond thus formed is called Polar covalent bond. e.g. HCl, NH3, H2O. H : Cl B. In such case bonded electrons are unequally shared between two different atoms and the electron pair is pulled towards more electronegative atom. C. Since electron pair is unequally shared, more electronegative atom aquires partial negative charge and less electronegative atom aquires partial positive charge thus molecule is referred as a dipole. D. Such polar compounds possess partial ionic character which can be calculated using the following formula. % ionic character = 16(XA- XB) +3.5 (XA – XB)2 ### 2. **Water (HO)** ### 1. **Ammonia (NH)** - **Electronegativity**: Oxygen (O) has an electronegativity of about - **Electronegativity**: Nitrogen (N) has an electronegativity of 3.5, while hydrogen (H) is again about 2.1. The difference (1.4) about 3.0, while hydrogen (H) has an electronegativity of about 2.1. indicates a significant polarity in the O-H bonds. The difference (0.9) indicates that nitrogen is more electronegative - **Bond Formation**: In the O-H bonds, oxygen pulls the shared than hydrogen. electrons closer to itself, resulting in a partial negative charge (-) on - **Bond Formation**: In the N-H bonds, nitrogen attracts the the oxygen atom and partial positive charges (+) on the hydrogen shared electrons more strongly than hydrogen does. This creates a atoms. partial negative charge (-) on the nitrogen atom and a partial - **Molecular Geometry**: Water has a bent (or V-shaped) structure positive charge (+) on each hydrogen atom. due to the two lone pairs on the oxygen atom. The asymmetry of this - **Molecular Geometry**: Ammonia has a trigonal pyramidal shape shape ensures that the dipoles from the O-H bonds do not cancel, due to the presence of a lone pair on the nitrogen atom. This leading to a net dipole moment. This makes HO a polar molecule as geometry means that the dipoles from the N-H bonds do not cancel well. Page 3 out, resulting in a net dipole moment, making NH a polar molecule. E. Hydrogen Bonding: Hydrogen Bonding : When Hydrogen is covalently bonded to highly electronegative atom X, (O, F, N,), The shared electron pair is pulled so close to X, that a strong dipole results, Defination: The electrostatic attaraction between H atom covalently bonded to highly electronegative atom X, and a lone pair of electrons of X in another molecule is called hydrogen bonding. Only O, N and F which have very high electronegativity and small atomic size are capable of forming hydrogen bonds. Hydrogen bond is longer and much weaker than normal covalent bond. Hydrogen bond results in long chain or cluster of large number of associated molecules. Like a covalent bond hydrogen bond has a bonding direction. Types of Hydrogen Bonding: i. Intermolecular H Bonding: This type of bond is formed between two different molecule of same of diferent substance. e.g. Hydrogen bonding in HF, H2O, NH3. This type of H bond result in the formation of associated molecules, thus substances with intermolecular H bond have high melting points, boiling points, viscocity, surface tension etc. Ice (Solid HO): Hydrogen bonding between water molecules. HS (Hydrogen Sulfide): Covalent bonds within each water molecule (O-H bonds). Covalent bonds between H and S. Dipole-dipole interactions and London dispersion forces between molecules. HF (Hydrogen Fluoride): Covalent bonds between H and F. Ethyne (CH): Strong hydrogen bonding between HF molecules. Covalent bonds within the molecule (C-H and CC bonds). London dispersion forces between molecules. Naphthalene (CH): Covalent bonds within the molecule. HO (Hydronium Ion): London dispersion forces between molecules (since it is nonpolar). Covalent bonds between H and O in the ion. Hydrogen bonding with surrounding water molecules in aqueous solution. Diamond (Carbon): Covalent bonds in a tetrahedral network (giant covalent structure) HCl (Hydrogen Chloride):. Polar covalent bonds between H and Cl. HO (Water): Dipole-dipole interactions between HCl molecules. Covalent bonds within each water molecule. Hydrogen bonding between water molecules. Page 4 Bond Strength: becoz of Molecular Structure: also water has higher molecular weight than The H-I bond is much weaker than the H-F bond. This is HF 1. Water has a bent molecular structure, which because iodine is much larger than fluorine, so the allows for extensive hydrogen bonding between overlap between the hydrogen and iodine orbitals is less molecules. effective, leading to a weaker bond that is easier to break. 2. Each water molecule can form up to four In contrast, the H-F bond is very strong due to the small hydrogen bonds (two from the hydrogen atoms size of fluorine, making the bond harder to break. and two due to lone pairs on oxygen). Bond Dissociation: 3. In contrast, HF can form only two hydrogen bonds (one from each HF molecule to another). In acids, the strength is determined by how easily 4. This results in fewer intermolecular interactions the molecule can donate a proton (H). Since the H-I in HF compared to water. bond is weaker, HI dissociates more easily to 5. The cumulative effect of multiple hydrogen release a proton, making it a stronger acid. bonds in water leads to stronger overall The strong H-F bond makes HF less likely to intermolecular forces, resulting in a higher dissociate, so it is a weaker acid. boiling point and allowing it to remain in the liquid state at room temperature. 6. HF has comparatively less intermolecular force ii. Intramolecular H Bonding: Intramolecular H bonding: This type of bond is formed between H atom and electronegative atom present within the same molecule, it results in cyclisation of the molecule therefore molecules exist as descrete unit and not in the associated form. It doesn't affect physical properties of a substance. o-Nitrophenol forms intramolecular hydrogen e.g. Hydrogen bonding in 2-Nitrophenol, 2-Nitrobenzoic acid. bonding between the hydroxyl group (-OH) and the nitro group (-NO) on the same molecule. This intramolecular hydrogen bonding reduces the extent of intermolecular interactions, which leads to a lower boiling point. p-Nitrophenol, on the other hand, forms intermolecular hydrogen bonding between different molecules. This stronger intermolecular hydrogen bonding increases its boiling point compared to o-nitrophenol. Because o-nitrophenol has a lower boiling point due to intramolecular hydrogen bonding, it will distill out first in a distillation process. Characteristics of H Bonding: 1. Abnormally high melting and boiling points. In case of intermolecular H bonding association of molecules physical properties like melting and boiling points unusually increases. e.g. H2O has high melting and boiling point as compared to H2S even though it has lower molecular weight, this is because of intermolecular H bonding which exist in H2O and not in H2S. 2. High solubility of some covalent compounds. There is high solubilities of some compounds containing O, N and F such as NH3, CH3OH in certain H containing solvents are due to H bonding. Page 5 e.g. NH3 and CH3OH are highly soluble in water due to H bonding. 3. Ice is lighter than Water. Since H bonds are directional and are pretty strong to form three dimentional crystal lattice. e.g. in ice water molecules are held together in a tetrahedral network and have same crystal lattice as of diamond, this is because O atom of water has two covalent bonds and can form two H bonds, these are distributed in a space like four covalent bonds of C in diamond, thus tetrahedral structural units are linked to other units through H bonds. Since there is enough empty space in its open lattice stucture of ice which increases its volume and ultimately decreases its density, while in case of Water it donot have lattice structure and molecules are free and compactly arranged and there no any empty space between them which decreases volume ultimately increases its density, hence ice is lighter than water. Difference between Ionic bond nad Covalent bond: Ionic Bond Covalent Bond of electrons 1. Formed by tranfernace from a metal to a 1. Formed by sharing of electrons between non-metal. nonmetal atoms. 2. To form ionic bond, electronegativity 2. To form covalent bond, electronegativity differnce between bonding atoms should be differnce between bonding atoms should be two or more. less than two. 3. Consists of electrosatatic force betwwen 3. Consist of shared pair of electrons cation and anion. between atoms. 4. Non- rigid and non-directional; cannot 4. Rigid and directional; can cause isomerism. cause isomerism. Properties of Ionic compounds: Properties of Covalnt compounds: 1. Solids at room temprature. 1. Gases,liquids or soft solids. 2. High melting and boiling points. 2. Low melting and boiling points 3. Hard and Brittle. 3. Soft and much readily broken. 4. Soluble in water but insoluble in organic 4. Insoluble in water but soluble in organic solvents. solvents. 5.Conductors of eletricity. 5. Non- conductors of electricity. 6. Undergoes ionic reactions which are fast. 6. Undergo molecular reactionswhich are slow. III. Co-ordinate covalent Bond. Page 6 A covalent bond in which both electrons of the shared pair of come from one of the two atoms (or ions) is called as coordinate covalent bond. Componds containing coordinate bond are called as coordinate compounds. If an atom have an unsharred pair of valence electrons i.e. a lone pair of electrons and another atom is short of two electrons to complete its octate, then the atom with lone pair of electrons donates it to the atom which is short of electrons and a coordinate bond is formed. Coordinate bond is no way different than ordinary covalent bond. The atom which donates a lone pair is called as 'Donor' and the atom which accepts the lone pair is called as 'Acceptor'. Lewis bases acts Donor species while Lewis acids act as acceptors. The coordinate bond is indicated by an arrow pointing from donor to acceptor. e.g. Addition compound of NH3 and BCl3. Aluminium Chloride, Al2Cl6. octate of central atom must be attained halogen has one lone pair which they are donating Ammonium Ion NH4+. Other e.g. BF4-, H3O+, SO2, SO3, CH3NO2, SO42-, O3, CO. Page 7 IV. Metallic Bond. The bond which holds the atoms of metal together in a metal crystal is called the Metallic Bonding. In case of pure metal it is not possible to transfer electrons from one atom of metal to another atom, as all atoms are same, and have same electronegativity, hence ionic bond is not possible, also sharing of electrons doesn't completes the octate of any atom hence covalent bond is also not possible. Also metals have many properties such as high melting temperatures, good conductance of heat and electricity in solid state, ductility, malleability, high tensile strength, etc. These cannot be explained in terms of either ionic bond or covalent bond. Many theories are there to explain metallic bonding, but will study one of them as Electron Sea Model OR Electron gas model. According to this theory Solid containing metallic bonding consists of positive metal ions (called kernels) in a cloud of valency electrons (called as electron gas or electron sea), Kernels occupy fixed positions and valence electrons lie in between the kernels and these are mobile. these i.e. the electrons are mobile V. The van der Waals forces There are few more forces which holds molecules together when they are close to one another out of which one is 'van der Waals force'. Definition: The intermolecular attractive forces operative between all molecules, when they are close to one another, are called van der Waals forces. The temporary and instantaneous shifting of electrons in a molecule causes it to development of a temporary dipole in it, which when placed near to another molecule induces dipole in other molecule, such process goes on throughout the bulk of material and such fluctuating dipole inall molecules causes a force of attraction between them called as vander Waals forces. The van der Waals forces are weak in nature and are significant only when molecules are very Page 8 close together. Trends: 1. The magnitude of van der Waals attraction increases with increase in number of electrons per molecule and, therefore also with molar mass hence b.p. of I2 (mol mass = 253) > Br2 (mol mass = 159.8) > Cl2 (mol mass = 76.91) > F2 (mol mass = 28.0)> H2 (mol mass = 2.01) 2. The van der Waals forces are of decreasing effectiveness with increasing temperature, Thus it is van der Waals attraction that causes non-polar substances (absence of polar bonds decreases the H bonding and ultimately their condensing and freezing points) such as noble gases and the halogens to condense to liquids and freeze into solids when the temperature is lowered enough. van der waal's force 1 VSEPR Theory: This theory tries to explain the shape and geometry of molecules which contains three or more than three atoms, uptill now we have seen only different types of bonds and not the geometries of molecules. Shape of molecule is related to the number of valence shell electron pairs, around the central atom in a molecule. This theory states that electron pairs (both lone pairs and bond pairs) surrounding the central atom will arrange themselves as far apart as possible to minimize repulsion between them. VSEPR theory is an important tool while determining the shape and geometry of the molecule, and some points are to be followed as: 1. It treats multiple bonds as a single group of electrons. 2. Order of repulsion between lone pairs and bond pairs is as: Lone pair - Lone pair > Lone pair - Bond pair > Bond pair - Bond pair. 1. Linear Molecules: e.g. Beryllium dichloride (BeCl2) The central atom Be has two bonding electron pairs and no lone pairs of electrons, according to VSEPR theory the bonding pairs will occupy positions on opposite sides of Be, forming an angle of 180O. An angle of 180O gives a straight line. Therefore BeCl2 molecule is linear. In general all molecules of the type A-B-A which have only two bond pairs and no lone pairs are Page 9 linear. e.g. CO2, HCN, Acetylene etc. 2. Trigonal Planar Molecules: e.g. Boron trifluoride (BF3) The central atom B has three bonding electron pairs and no lone pairs of electrons, according to VSEPR theory the bonding pairs will occupy positions as far as possible, this will lead the bonding pairs to the three vertices of an equilateral triangle and B atom at the center of it, forming a trigonal planar molecule having a bond angle of 120O. All four atoms i.e. One B and three F atoms lie in the same plane. e.g. SO3, BCl3, BBr3, 3. Tetrahedral Molecules: e.g. Methane: (CH4) The central atom C has four bonding electron pairs and no lone pairs of electrons, according to VSEPR theory the bonding pairs will occupy positions apart as far as possible, this will lead the bonding pairs to the four vertices of regular tetrahedron and C atom at the center of the tetrahedron, forming a tetrahedral molecule having four faces which are equilateral triangles, In this structure bond angles are 109.5O. e.g. NH4+ ion, SO42- ion. 4. Pyramidal Molecules: e.g. Ammonia (NH3) The central atom N has three bonding electron pairs and one lone pair of electrons, according to VSEPR theory these electron pairs will occupy positions apart as far as possible, this leads all the electron pairs in the direction of four vertices of regular tetrahedron and N atom at the center of this tetrahedron, but the shape of molecule is determined by the arrangement of atoms and not the electron pairs, thus if we see only at the atoms, NH3 froms a pyramidal shape with N atom located at the apex and three H atoms at the three corners of the triangular base. Page 10 Here it can be seen that bond angle shown is 107.3O, according to VSEPR theory, a lone pair exerts greater repulsion on bond pairs than the bond pairs do it on each other. As a result the lone pair of N in NH3, will exert higher repulsion on bond pairs pushing them closer to each other, decreasing the bond angle from 109.5O to 107.3O. e.g. PCl3, 5. Bent or Angular Molecules: e.g. Water (H2O) The central atom O has two bonding electron pairs and two lone pair of electrons, according to VSEPR theory these electron pairs will occupy positions apart as far as possible, this will lead all the electron pairs to the four vertices of regular tetrahedron and O atom at the center of this tetrahedron, but the shape of molecule is determined by the arrangement of atoms and not the electron pairs, thus if we see only at the atoms, H2O froms an angular shape. Here it can be seen that bond angle shown is 105O, according to VSEPR theory, a lone pair exerts greater repulsion on bond pairs than the bond pairs do it on each other. As a result the two lone pairs of O in H2O will exert higher repulsion comparred to one lone pair of N in NH3, on the bond pairs pushing them much closer to each other, decreasing the bond angle from 109.5O to 105O. e.g. SO2, Page 11 Valence Bond Theory (VBT): Bond formation between two atoms to give chemical compounds can be well explained in terms of atomic orbitals overlapping and their interaction with each other. Ionic or electrovalent bond formation involves physical transfer of the electron and atomic orbital theory is not useful for its explanation. The atomic orbital theory involves the concept of overlapping of orbitals which explains the covalent bond formation very well. Heitler and London state that: QB i. To form a covalent bond , overlapping orbitals must have unpaired electrons with anti spins. ii. Two combining atoms must come closer to each other with proper alignment of axis of overlapping orbitals so that the orbital of one overlaps with that of other. iii. Due to overlap, new localised bond orbital is formed. iv. Formation of covalent bond is due to pairing of antispin electrons and electrostatic attraction between nuclei and the electrons in the newly formed localised bond orbital. v. Greater the extent of overlap, more will be the attraction giving out more bond energy hence more stable will be the bond formed. vi. Greater the overlapping , shorter is the bond length. During the process of overlapping of orbitals some forces are affecting the overall process : i. Attractive forces between nucleus of one atom and electrons of other atom. ii. Repulsive forces between nuclei of both atoms. iii. Repulsive forces between electrons of both atoms. With the active interaction of these forces, orbitals try to overlap and an equilibrium distance is attained where both the atoms are stabilised with the other, this equilibrium distance is nothing but bond length and the energy released during overlapping and stabilisation is called as Bond Energy. e.g Formation of H2 molecule showing s-s orbital overlap (Fig. below). Page 12 Overlapping of atomic orbitals forms covalent bonds and there are different type of overlaps hence different types of covalent bonds formed as: 1. Sigma Bond. ( σ ) 2. Pi Bond. ( π ) 1. Sigma Bond. ( σ ) When there is end to end overlapping of atomic orbitals along the internuclear axis (by convention which is 'Z' axis of cartaesian coordinate axis) then bond formed is called as Sigma Bond. It is also called as head-on overlapping or axial overlapping. Depending on type of orbitals taking part, Sigma bond can be formed in following ways as: a. s - s overlapping When two s orbitals of two different atoms overlaps along the internuclear axis they form a sigma covalent bond as: b. s - p overlapping When s orbital of one atom and p orbital of another atom overlaps along the internuclear axis they form a sigma covalent bond as: c. p - p overlapping When two p orbitals of two different atoms overlaps along the internuclear axis they form a sigma covalent bond as: 2. Pi Bond. ( π ) Page 13 When there is sidewise overlapping of atomic orbitals (specifically p orbitals) perpendicular to internuclear axis then bond formed is called as Pi Bond. The type overlapping is also called as lateral overlapping. Here the extent of overlap in case of Sigma bond is higher than that of Pi bond hence Sigma bond is stronger than Pi bond. 1. Formation of H2 molecule Each H atom has one unpairred electron in 1s orbital. Two such atoms join to form a H2 molecule, In this case s - s overlapping between two 1s - orbitals of Hydrogen takes place resulting in a sigma ( σ ) bond formation, and H2 molecule as: 2. Formation of O2 molecule Oxygen atom has electronic configuration as 1s2, 2s2, 2px2, 2py1, 2pz1. In the formation of O2 molecule the orbitals taking part are as: in 2py1, 2pz1 as they contain an unpaired electron each. Two such atoms join to form an O2 molecule. Here as per convention, z axis is to be considered the molecular axis, 2pz1 orbitals of both the atoms will overlap axially resulting in a sigma ( σ ) bond and 2py1 orbitals of both the atoms will overlap lateraly i.e. sidewise resulting in a pi ( π ) bond. Thus O2 molecule contains a double bond consisting of a sigma ( σ ) bond and a pi ( π ) bond. Page 14 QB Difference between Sigma Bond ( σ ) and Pi Bond ( π ). Sigma Bond ( σ ) Pi Bond ( π ) 1. It is formed by end to end overlapping of 1. It is formed by sidewise overlapping of half half filled atomic orbitals. filled p orbitals only. 2. Overlapping takes place along internuclear 2. Overlapping takes place perpendicular to axis. internuclear axis. 3. The extent of overlapping is large and bond 3. The extent of overlapping is small and bond formed is stronger. formed is weaker. 4. The molecular orbital formed is 4. The molecular orbital formed as a result of symmetrical about the internuclear axis. overlapping consists of two lobes above and below the internuclear axis. 5. There is free rotation about σ bond and no 5. There is no free rotation about π bond and geometrical isomers are possible. geometrical isomers are possible. 6. The bond can be present alone. 6. The bond is always formed in addition to sigma (σ) bond. 7. s and p orbitals can participate in the 7. Only p - orbitals participate in the formation formation of σ bond. of pi (π) bond. Page 15 Concept of Hybridization: Formation of simple molecules and their geometry could be explained easily by orbital overlapping theory, but in case of complex molecules it is difficult to explain their geometries as well as bond energies. To address this issue a new concept called Hybridization is introuduced. It is nothing but mixing QB of atomic orbitals of an atom, of nearly equal energies, giving rise to new hybrid orbitals equal in number to the mixing orbitals, these new orbitals have same energy and have identical shapes. For Hybridisation to occur below mentioned are the conditions: 1. Orbitals on a single atom only would undergo hybridization. 2. There should be very little difference of energy level between the orbitals mixing to form hybrid orbitals. 3. Number of hybrid orbitals generated is equal to the number of hybridizing orbitals. 4. The hybrid orbitals assume the direction of dominating orbitals. For e.g if s orbitals and p orbital are to hybridize, The s orbital don't have any directional character and does not contribute toward the direction and p orbitals determines the directional character of the hybrid orbitals. 5. It is the orbital that undergo hybridization and not the electrons. For e.g. four orbitals of oxygen atom (2s2, 2px2, 2py1,, 2pz1.) belonging to second level (i.e. 2s, 2px, 2py, 2pz.) can hybridize to give four hybrid orbitals, two of which have two electrons each (as before) and the other two have one electron each. 6. The electron waves in hybrid orbitals repel each other and thus tend to be farthest apart. Types of Hybridization: Due to hybridization valence orbitals take a new shape and energy level, it becomes important while determining shape and geometry of the molecules. Depending upon number and nature of the orbitals undergoing hybridization, there are different types of Hybrid orbitals. Here we are going to consider the hybridizations involving s, p, and d orbitals of simple atoms. Hybridisation involving s and p orbitals a. sp Hybridization: One s and one p orbitals mix together to form two sp hybridized orbitals and the process is called as sp hybridization. Each sp hybrid orbital has 50% s-character and 50% p-character. This type of hybridization is also called as Linear hybridization and the orbitals are called as Linear Page 16 Hybrids. There is repulsion between these two new orbitals, to minimize this repulsion they arrange themselves far apart from each other and attain a linear alignment with nucleus at the center, here bond angle is found to be 180O. The shape of each newly formed sp hybrid orbitals is dominated by p orbital and have two unequal lobes, of which the bigger lobe involves itself in overlapping and bond formation while the smaller lobe is neglected during the process. acetylene Examples of sp hybridized central atom compounds are BeCl2, BeF2 , C2H2. Let us consider an example of BeF2 Beryllium atom have electronic comfiguration as 1s2, 2s2, 2px0, 2py0, 2pz0. Here although both s orbitals seems to be fully filled up, it has a vacant 2p orbital of nearly equal energy as 2s orbitals, and they can get mixed up to form hybrid orbitals. Here Be atom gets excited and one electron from 2s orbital is promoted to next vacant 2px orbital, and excited state configuration becomes as 1s2, 2s1, 2px1. Now the orbitals containing an unpaired electrons mixes up with each other and form two new equivalent sp hybridized orbitals. These new orbitals now repel each other and gain a linear shape with the nucleus at center. These two sp hybridized orbitals now overlap and axially with p orbitals of two Fluorine atoms and form two sigma bonds with them.The two sp hybridized orbitals have linear geometry gives linear shape to BeF2 molecule, with Be atom at the center and two F atoms at opposite ends of linear molecule, giving the bond angle of 180O. Page 17 b. sp2 Hybridization: One s and two p orbitals mix together to form three sp2 hybridized orbitals and the process is called as sp2 hybridization. Each sp2 hybrid orbital has 33.33% s-character and 66.67% p-character. This type of hybridization is also called as Trigonal hybridization and the orbitals are called as Trigonal Hybrids. There is repulsion between these three new orbitals, to minimize this repulsion they arrange themselves far apart from each other and attain a planar triangular shape with nucleus at the center, here bond angle is found to be 120O. Similar to sp hybridized orbitals shape of each newly formed sp2 hybrid orbitals is dominated by p orbital and have two unequal lobes, of which the bigger lobe involves itself in overlapping and bond formation while the smaller lobe is neglected during the process. Examples of sp2 hybridized central atom compounds are BF3, NO3-, C2H4. ethylene Let us consider an example of BF3 Boron atom have electronic comfiguration as 1s2, 2s2, 2px1, 2py0, 2pz0.Here Boron is expected to form only one bond as 2s orbital seems to be fully filled up, but it known that it forms compounds as BF3, BCl3, BH3 indicating that it have capacity to form three bonds. So actually it promotes one of the two electrons from 2s2 orbital to the nearest vacant 2py0 orbital and the excited state electronic configuration becomes as 1s2, 2s1, 2px1, 2py1, 2pz0. Now the orbitals containing an unpaired electrons i.e. 2s1, 2px1, 2py1. mixes up with each other and form three new equivalent sp2 hybridized orbitals. These new orbitals now repel each other and gain a Triangular planer shape with nucleus at center. These three sp2 hybridized orbitals now overlap and axially with pz orbitals of three Fluorine atoms and form three sigma bonds with them. The three sp2 hybridized orbitals have Triangular planar geometry gives Triangular planer shape to BF3 molecule, with B atom at the center and three F atoms at three vertices of the triangle giving the bond angle of 120O. Page 18 c. sp3 Hybridization: One s and three p orbitals mix together to form four sp3 hybridized orbitals and the process is called as sp3 hybridization. Each sp3 hybrid orbital has 25% s-character and 75% p-character. This type of hybridization is also called as Tetrahedral hybridization and the orbitals are called as Tetrahedral Hybrids. There is repulsion between these four new orbitals, to minimize this repulsion they arrange themselves far apart from each other and attain a tetrahedral shape with nucleus at the center, here bond angle is found to be 109.5O. Similar to sp hybridized orbitals shape of each newly formed sp2 hybrid orbitals is dominated by p orbital and have two unequal lobes, of which the bigger lobe involves itself in overlapping and bond formation while the smaller lobe is neglected during the process. Examples of sp3 hybridized central atom compounds are SO42-, ClO4-, CH4, H2O, NH3. Let us consider an example of CH4, Page 19 Carbon atom have electronic comfiguration as 1s2, 2s2, 2px1, 2py1, 2pz0.Here Carbon is expected to form only two bond as 2s orbital seems to be fully filled up, but it known that it forms compounds as CH4, CCl4 indicating that it has capacity to form four bonds. So actually it promotes one of the two electrons from 2s2 orbital to the nearest vacant 2pz0 orbital and the excited state electronic configuration becomes as 1s2, 2s1, 2px1, 2py1, 2pz1. Now the orbitals containing an unpaired electrons i.e. 2s1, 2px1, 2py1, 2pz1. mixes up with each other and form four new equivalent sp3 hybridized orbitals. These new orbitals now repel each other and gain a Tetrahedral shape with nucleus at center. These four sp3 hybridized orbitals now overlap with s orbitals of four Hydrogen atoms and form four sigma bonds with them. The four sp3 hybridized orbitals have Tetrahedral geometry gives Tetrahedral shape to CH4 molecule, with C atom at the center and four H atoms at four vertices of the Tetrahedron giving the bond angle of 109.5O. Hybridisation in other carbon compounds: As mentioned above in CH4 molecule C atom have got sp3 hybridization. Below mentioned are few examples which contain C atom with other than sp3 hybridization. e.g. 1. C2H4 Ethylene: In case of C2H4 molecule, Both C atoms are sp2 hybridized, and during hybridization only one s and two p orbitals take part in hybridisation as: Carbon atom have electronic comfiguration as 1s2, 2s2, 2px1, 2py1, 2pz0. Page 20 In excited state it promotes one electron from 2s2 to 2pz0 orbital, and configuration becomes as 1s2, 2s1, 2px1, 2py1, 2pz1, and during hybridization only 2s1, 2px1 and 2py1 orbitals gets mixed up together giving sp2 hybridization and three new sp2 hybridized orbitals, allined in triangular planer geometry, remaining 2pz1 orbital arranges itself perpendicular to above triangular planer strucuture of sp2 hybridized orbitals. Then three sp2 hybridized orbitals forms three sigma bonds as: Two sigma bonds with two Hydrogen atoms and one sigma bond with other carbon atom. and remaining 2pz1 orbital of one carbon overlaps sidewise with 2pz1 orbital of other carbon atom forming a pi bond. Hence in ethene molecule one carbon is attached to other carbon atom by two bonds as one sigma and one pi bond. 2. C2H2 Ethyne In case of C2H2 molecule, Both C atoms are sp hybridized, and during hybridization only one s and one p orbitals take part in hybridisation as: Carbon atom have electronic configuration as 1s2, 2s2, 2px1, 2py1, 2pz0. In excited state it promotes one electron from 2s2 to 2pz0 orbital, and configuration becomes as 1s2, 2s1, 2px1, 2py1, 2pz1, and during hybridization only 2s1 and 2px1 orbitals gets mixed up together giving sp hybridization and two new sp2 hybridized orbitals, allined in linear geometry, remaining two orbitals viz. 2py1 2pz1 arranges themselves perpendicular to above linear strucuture of sp hybridized orbitals. Then two sp hybridized orbitals forms two sigma bonds as: One sigma bonds with one Hydrogen atom and one sigma bond with other carbon atom. and remaining 2py1 and 2pz1 orbital of one carbon overlaps sidewise with 2py1 and 2pz1 orbital of other carbon atom forming two pi bonds. Hence in Ethyene (Acetylene) molecule one carbon is attached to other carbon atom by three bonds as one sigma and two pi bonds. Page 21 Hybridization involving s, p and d orbitals There are several types of hybridizations involving d orbitals, Since d orbitals have relatively complex shapes so we will consider some common types which are sp3d, sp3d2 and dsp2. 1. sp3d Hybridization: It involves mixing of one s orbital three p orbitals and one d orbital of same energy level, like 3s, 3p and 3d, after mixing and hybridization five new sp3d hybridized orbitals are formed, of which three spread in a planer shape attaining triangular shape and remaining two arrange themselves perpendicular to the triangle above and attain a geometry of Trigonal bipyramid. e.g. PCl5 molecule. In case of PCl5 molecule central atom P has the electronic configuration as 1s2, 2s2, 2p6, 3s2, 3px1, 3py1, 3pz1, 3d0 in ground state. It have only three unpaired electrons in its valence shell. One of the two electrons in 3s2 orbital is promoted to nearest vacant 3d0 orbital and excited state configuration becomes as 1s2, 2s2, 2p6, 3s1, 3p3, 3d1 and then one 3s1, three 3p3, and one 3d1 orbitals get mixed up together and are sp3d hybridized forming five new orbitals. Page 22 These five sp3d hybridized orbitals now overlap with five p orbitals of five different Chlorine atoms to form PCl5 molecule as below: Here Bond angles can be seen as, Three bonds in plane makes bond angle of 120O with each other and two bonds makes a bond angle of 90O to the plane of triangle. 2. sp3d2 Hybridization: It involves mixing of one s orbital three p orbitals and two d orbitals of same energy level, like 3s, 3p and 3d, After mixing and hybridization six new sp3d2 Hybridized orbitals are formed, of which four spread in a planer shape attaining square shape and remainig two arrange themselves perpendicular to the square above and attain a geometry of Square bipyramid which is known as Octahedral. e.g. SF6 molecule. In case of SF6 molecule central atom S has the electronic configuration as 1s2, 2s2, 2p6, 3s2, 3px2, 3py1, 3pz1, 3d0 , in ground state. It have only two unpaired electrons in its valence shell. One of the two electrons in 3s2 orbital and one of the two electrons in 3px2 orbital is promoted to nearest vacant 3d0 orbitals and excited state configuration becomes as 1s2, 2s2, 2p6, 3s1, 3p3, 3d2 and then one 3s1, three 3p3, and two 3d2 orbitals get mixed up together and are sp3d2 hybridized forming six new orbitals. Page 23 These six sp3d2 hybridized orbitals now overlap with six p orbitals of six different Fluorine atoms to form SF6 molecule as below: Here Bond angles can be seen as, Four bonds in plane makes bond angle of 90O with each other and two bonds makes a bond angle of 90O to the plane of square. So all bond angles in SF6 molecule are 90O each. Limitations of Valence Bond Theory: Valence bond theory explains about covalent bond formation, bond energies, geometries of various molecules, but it fails to explain: QB 1. The presence of other nuclei affecting the electronic arrangement of all the atoms in the molecule. 2. Sometimes a single electronic structure does not explain all known properties of that molecule or ion and we have the many electronic structures called resonating structures. 3. Valence bond theory fails to explain bonding in electron deficient compounds. 4. It fails to explain the paramagnetic and diamagnetic characters of various compounds. Molecular Orbital Theory To overcome limitations of valence bond theory Hunds and Mulliken proposed a theory as Molecular Orbital Theory. According to this theory, when two atoms approach each other to form a molecule, atomic orbitals of all the atoms get disturbed, they get mixed with each other and form equal number of new orbitals that belongs to the molecule and not to any specific atom. These newly generated orbitals are called as Molecular Orbitals. Hence every molecule is supposed to have its own molecular orbitals similar to an atom to have its own atomic orbitals. A molecular orbital can be defined as 'It is a polycentric region in space, defined by its size and shape, with two or more atoms in a molecule and each has a capacity to occupy two electrons Page 24 with opposite spins.' Main features of the theory are as follows: 1. A molecule is quite different from its constituent atoms and all electrons in the molecule belongs to all the atoms and are considered to be moving under the influence of all nuclei. 2. Atomic orbitals of individual atoms combine to form molecular orbitals and these MOs are filled up in the same way as atomic orbitals, following the Aufbau's principle, Pauling's exclusion principle and Hund's rule of maximum multiplicity. 3. Molecular orbitals have definite energy levels. 4. The shape of MOs formed depend upon the shape of combining atomic orbitals. Linear Combination of Atomic Orbitals (LCAO method) Acoording to wave mechanics atomic orbitals are shown by a symbol Ψs, similarly molecular orbitals can be shown by the symbol ΨMO. The Linear Combination of Atomic Orbitals to from MO can take place either by addition (constructive) or by substraction (destructive) of wave functions of atomic orbitals as A. MO obtained by addition of wave function of AO's involved. ΨMO = ΨA + ΨB (constructive interaction) MO fromed by addition of wave function of AO's is called as Bonding Molecular Orbital. (B.M.O.). Characterstics: a. The energy of BMOs is less than that of constituent overlapping atomic orbitals and the difference between them is called as stabilisation energy thus BMO stabilizes the molecule. b. It possesses high electron density in the region between the two nuclei. c. Every electron in it makes contribution to the attraction of two combining atoms. d. It is only formed when the lobes of combining atomic orbitals possess the same sign. B. MO obtained by substraction of wave function of AO's involved. Ψ*MO = ΨA - ΨB (destructive interaction) MO fromed by substraction of wave function of AO's is called as Antibonding Molecular Orbital. (A.B.M.O.) Characterstics: a. The energy of ABMOs is higher than that of constituent overlapping atomic orbitals and the difference between them is called as destabilisation energy thus ABMO destabilizes the molecule. b. It possesses low electron density in the region between the two nuclei. c. Every electron in it makes contribution to the replusion of two combining atoms. Page 25 d. It is only formed when the lobes of combining atomic orbitals possess the opposite sign. To differentiate between bonding molecular orbital and antibonding molecular orbital, to ABMO an asterisk is attached. e.g when two 1s AOs of any two H atoms combines they form σ BMO amd σ * ABMO as shown below: The probability of formation of molecular orbital is given by square of Ψ2 i.e. for BMO Ψ2 = ( ΨA + ΨB )2 Ψ2 = ΨA2 + 2ΨAΨB + ΨB2 Ψ2 = (ΨA2 + ΨB2) + 2ΨAΨB................... (i) for ABMO Ψ*2 = ( ΨA - ΨB )2 Ψ*2 = ΨA2 - 2ΨAΨB + ΨB2 ΨA2 + ΨB2 = Ψ*2 + 2ΨAΨB................... (ii) Substituting equation (ii) in (i) Ψ2 = Ψ*2 + 2ΨAΨB + 2ΨAΨB Ψ2 = Ψ*2 + 4ΨAΨB Here we can say that Ψ2 > Ψ*2 Probability of bonding molecular orbitals (Ψ2) formation is greater than that of antibonding molecular orbital (Ψ*2) formation. s orbital + s orbital Type of M.O.: This combination will result in a sigma () bonding orbital and a sigma-star () antibonding orbital*. Reason: The overlap of two spherical s orbitals is symmetrical along the internuclear axis, forming a orbital. s orbital + pz orbital Type of M.O.: This can form a sigma () bonding orbital and a sigma-star () antibonding orbital*. Reason: The pz orbital is oriented along the internuclear axis, so when it overlaps with an s orbital (which is also symmetrical), the overlap is along the same axis, leading to a orbital pz orbital + pz orbital Type of M.O.: This combination will result in a sigma () bonding orbital and a sigma-star () antibonding orbital*. Reason: The pz orbitals overlap end-to-end along the internuclear axis, leading to the formation of and * orbitals. px orbital + px orbital Type of M.O.: This will form a pi () bonding orbital and a pi-star () antibonding orbital*. Reason: The px orbitals are oriented perpendicular to the internuclear axis and overlap side-by-side, which forms a orbital. py orbital + py orbital Type of M.O.: This will form a pi () bonding orbital and a pi-star () antibonding orbital*. Reason: Like px orbitals, py orbitals are also oriented perpendicular to the internuclear axis and overlap side-by-side, resulting in a Page 26 C. The inner orbital MOs which are not taking part in bond formation are called as non-bonding MO's. If a molecule contains one or more unpaired electrons in its MO's then it is paramagnetic in nature otherwise it is diamagnetic in nature. Higher the number of unpaired electrons higher is the paramagnetic character. Difference Between BMO and ABMO Bonding MO Antibonding MO 1. It is formed by addition overlap of atomic 1. It is formed by subtraction overlap of atomic orbitals. orbitals. 2. The wave function of an antibonding MO is 2. The wave function of an antibonding MO is given by ΨMO = ΨA + ΨB.. given by Ψ*MO = ΨA - ΨB. 3. Its formation takes place when the lobes of 3.Its formation takes place when the lobes of atomic orbitals have same sign. atomic orbitals have different signs. 4. The energy of bonding MO is lower than 4. The energy of bonding MO is higher than that of the atomic orbitals from which it is that of the atomic orbitals from which it is formed. formed. 5. The electron density is high in the region 5. The electron density is low in the region between the nuclei of bonded atoms. between the nuclei of bonded atoms. 6. Every electron in bonding MO contributes 6. Every electron in bonding MO contributes towards the attractive forces. towards the repulsive forces. Page 27 Conditions to form Molecular Orbitals: There are some conditions to be fulfilled by Atomic orbitals to mix up together and form Molecular Orbitals as: 1. The combining AOs should have comparable energies, and orbitals from two different energy level cannot combine, that means 1s orbital of one atom cannot combine with 2s orbital of other atom, it will combine only with 1s orbital of other atom and form MO. 2. Combination of AO's and formation of MO's is only possible if overlapping takes place to a considerable extent, so greater is the overlapping greater is the density of bonding electrons between the nuclei. 3. The combining atomic orbitals should possess the same symmetry about the molecular axis, Thus s orbital can combine with either of pX or pY or pZ orbital as s orbital is spherical in shape and can overlap with p orbitals directed along the perticular axis, e.g. If s orbital overlaps with pZ orbital then it cannot overlap with pX or pY orbitals of same energy level. By convention Z axis is taken as sigma bonding axis hence the allowed combinations can be summarised as below: Allowed combinations of Atomic Orbitals 1st Orbital 2nd Orbital Type of MO formed s - orbital s - orbital σ (Sigma) s - orbital pZ - orbital σ (Sigma) pZ- orbital pZ- orbital σ (Sigma) py- orbital py- orbital π (pi) px- orbital px- orbital π (pi) Shapes of Molecular Orbitals Two atomic orbitals combine to form molecular orbitals, depending upon the type of orbital combining and geomtery along the internuclear axis, different types of MO's are formed as below: A. 1s with 1s: When two 1s orbitals or two 2s orbitals overlap upon internuclear axis with each other they form 2 MO's as a BMO ( σ ) and ABMO (σ * ) as below: Page 28 B. 2s with 2pZ: When 2s and 2pZ orbitals overlap upon internuclear axis with each other they form 2 MO's as a BMO ( σ ) and ABMO (σ * ) as below: C. 2pZ with 2pZ: When two 2pZ orbitals overlap upon internuclear axis with each other they form 2 MO's as a BMO ( σ ) and ABMO (σ * ) as below: C. 2pX with 2pX or 2pY with 2pY : When two 2px or 2py orbitals overlap laterally or sidewise perpendicular to internuclear axis with each other they form 2 MO's as a BMO ( σ ) and ABMO (σ * ) as below: Page 29 Difference Between Sigma (σ) MO and Pi (π) MO Sigma (σ) MO Pi (π) MO 1. It is formed by head to head overlap of 1. It is formed by sidewise overlap of atomic atomic orbitals. orbitals. 2. Theoverlap is along the internuclear axis. 2. The overlap is perpendicular to the internuclear axis. 3. As a result of this strong bond is formed. 3. As a result of this weak bond is formed. 4.This orbital is symmetrical to rotation 4. This orbital is not symmetrical to rotation about the line joiningthe two nuclei. about the line joiningthe two nuclei. 5. The region of overlap is greater. 5. The region of overlap is lesser. We can enlist the bonding molecular orbitals as: σ(1s) σ(2s) σ(2pZ) π(2px) π(2py) and antibonding molecular orbitals as: σ*(1s) σ*(2s) σ*(2pZ) π*(2px) π*(2py) and with respect to the energies related to these orbitals the increasing order of energies will be as: σ(1s) < σ*(1s)< σ(2s) < σ*(2s) < σ(2pZ) < [π(2px) = π(2py)] < [π*(2px) = π*(2py)] < σ*(2pZ). For this order the energy diagram will be as: Page 30 Bond Order: Bond order is measure of strength of bond between two atoms in a molecule. Using molecular orbital theory it can be calculated by given formula. (No. electrons in _ (No. of electrons in Bond Order = bonding orbitals) antibonding orbitals) 2 In general practice MOs formed from valence orbitals are considered for determining bond order.Bond orders calculated are found to be in confirmation with other methods of determining it. Hence MO theory helps to determine whether the bond formation and ultimately the molecule formation is possible or not. We can say, (a) A molecule is stable if Nb > Na (b) A molecule is unstable if Nb < Na Information given by Bond Order: 1. Stability of Molecule A molecule / ion is stable if Nb > Na 2. Bond Dissociation Energy Greater the bond order, greater is the bond dissociation energy. 3. Bond length Bond order is inversely proportional bond length, Higher the bond order smaller the bond length. Magnetic Properties The presence of unpaired electron in molecular orbitals of species makes it paramagnetic in nature. Greater the number of unpaired electrons higher will be its paramagnetic character. On the other hand, if no unpaired electrons are there in MO's then the species will be diamagnetic in nature. As we have studied about formation of various molecular orbitals, energy level diagram, elctronic configuration of MO's, Bond order and properties related to it, we can now apply this theory to form homonuclear diatomic and heteronuclear diatomic molecules. Page 31 Homonuclear Diatomic Molecules: When two atoms of same element are bonded together, the molecule is called as homonuclear diatomic molecule. e.g. 1. Hydrogen H2 Hydrogen molecule is formed by combining two 1s1 orbitals of two hydrogen atoms. It gives rise to two molecular orbitals as σ(1s) and σ*(1s), The molecule has two electrons to be accomodeted in these orbitals, and are filled by following Aufbau's order of filling, Both electrons go to lower energy molecular orbital first i.e. bonding molecular orbital and then to higher energy molecular orbital i.e. antibonding molecular orbital. So the electronic configuration can be written as : σ(1s2) σ*(1s0) and MO diagram will be as: Here bond order can be calculated as 1 Bond Order = 2 𝑥(2 − 0) = 1 We can say that H2 molecule have a single bond between two hydrogen atoms. 2. Helium H2 In case of Helium, It have electronic configuration as : 1s2, so after combining two atoms the molecule willl have total four electrons to be accomodated in two molecular orbitals as σ(1s) and σ*(1s), so two electrons will go to σ(1s) and remaining two will go to σ*(1s), and electronic configuration of the molecule will be as : σ(1s2) σ*(1s2) Page 32 Here bond order can be calculated as: 1 Bond Order = 2 ∗ (2 − 2) 1 =2 ∗ (0) = 0 Here, Bond order comes out to be zero that means no bond exists between two He atoms and they exists as independant atoms. 3. Nitrogen N2 Nitrogen atom has electronic configuration as 1s2 2s2 2pX1 2pY1 2pZ1 here we are going to neglect 1s2 orbital and consider only valence shell i.e. 2s2 2pX1 2pY1 2pZ1, it have 4 atomic orbitals and will contribute 5 electrons, so two nitrogen atoms will form 8 molecular orbitals and will contribute total 10 electrons. Nitrogen molecule thus formed will have molecular orbitals with 10 electrons accomodated in them as KK* σ(2s2) σ*(2s2) π(2px2) π(2py2) σ(2pZ2) π*(2px0) π*(2py0) σ*(2pZ0). Molecular Orbital diagram will be as: Page 33 As it seen from the diagram that there are two electrons which are unpaired i.e. π*(2px1) π*(2py1) molecular orbitals, it imparts the molecule paramagnetic character. Bond order for N2 can be calculated as 1 Bond Order = 2 ∗ (8 − 2) 1 =2 ∗ (6) =3 We can say that N2 molecule have a triple bond between two nitrogen atoms. 4. Oxygen O2 Oxygen atom has electronic configuration as 1s2 2s2 2pX2 2pY1 2pZ1 here we are going to neglect 1s2 orbital and consider only valence shell i.e. 2s2 2pX2 2pY1 2pZ1, it have 4 atomic orbitals and will contribute 6 electrons, so two oxygen atoms will form 8 molecular orbitals and will contribute total 12 electrons. Oxygen molecule thus formed will have molecular orbitals with 12 electrons accomodated in them as KK* σ(2s2) σ*(2s2) σ(2pZ2) π(2px2) π(2py2) π*(2px1) π*(2py1) σ*(2pZ0). Molecular Orbital diagram will be as: Page 34 As it seen from the diagram that there are two electrons which are unpaired i.e. π*(2px1) π*(2py1) molecular orbitals, it imparts that the molecule have paramagnetic character. Bond order for O2 can be calculated as 1 Bond Order = 2 ∗ (8 − 4) 1 =2 ∗ (4) =2 We can say that O2 molecule have a double bond between two oxygen atoms. Similar to above examples, the electronic configurations, bond orders, and magnetic character of other species like O2+, O2-, O22- are summarised below. Species No. of Electronic Configuration Bond Order Magnetic valence character remove electrons electron from one of O2+ 11 KK σ(2s2) σ*(2s2) σ(2pZ2) π(2px2) π(2py2) (8 − 3) Paramagnetic the two O π*(2px1) π*(2py0) σ*(2pZ0). 2 atoms only = 2.5 O2- 13 KK σ(2s2) σ*(2s2) σ(2pZ2) π(2px2) π(2py2) (8 − 5) Paramagnetic π*(2px2) π*(2py1) σ*(2pZ0). 2 = 1.5 O22- 14 KK σ(2s2) σ*(2s2) σ(2pZ2) π(2px2) π(2py2) (8 − 6) = 1 Diamagnetic π*(2px2) π*(2py2) σ*(2pZ0). 2 -------------------------------------------- Page 35