Unit 4 Chemical Bonding PDF
Document Details
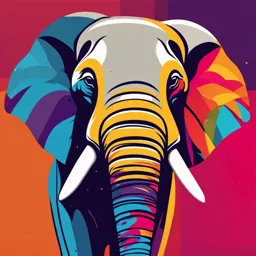
Uploaded by IntelligentOsmium585
Tags
Summary
This document is a chemistry study guide, specifically focused on chemical bonding. It includes detailed explanations and examples of how atoms form bonds in ionic and covalent compounds.
Full Transcript
Unit 4 Chemical Bonding Table of contents Ionic bonding Covalent bonding VSEPR Properties of Ionic Compounds/Covalent Molecules Naming Ionic Compounds and Covalent Molecules Polarity Intermolecular forces The friendship of Lithium and Chlor...
Unit 4 Chemical Bonding Table of contents Ionic bonding Covalent bonding VSEPR Properties of Ionic Compounds/Covalent Molecules Naming Ionic Compounds and Covalent Molecules Polarity Intermolecular forces The friendship of Lithium and Chlorine I love you! Uh huh Once upon a time there were two elements The first was lithium He had a dog e And one valence electron The other was chlorine She owned a e feral, possibly e diseased monkey e And had e seven *cough* e valence e electrons e One day, lithium met chlorine: e e e I like animals! I like my e monkey, even e through he throws poop. e e e But they were both sad: I have one valence e electron but I don’t e e want any. I want to be like my pal helium e e He has a motorcycle e e I have seven valence electrons, but I e want one more to be like my pal argon Lithium had an idea! If you want one e valence electron to be like argon and I Why won’t want to lose one you feed me? valence electron to be like neon, why don’t I just give you my electron and make us both happy? Chlorine thought about this a bit… e If I gain an electron, e I’ll have an overall -1 charge. e If you give me an e electron, you’ll have an overall +1 charge. e Our opposite charges e will cause us to be together forever! e Lithium had already figured that out e I know. That’s what I was getting at. You’ll have a negative charge, I’ll have a positive charge, and we’ll be together forever and ever and ever and ever and ever and ever and ever and ever… Though chlorine was kind of creeped out by how many times lithium said “and ever”, she decided to take an electron e from lithium. e e e e e e e This gave lithium a +1 charge and chlorine a -1 charge. As a result, they stuck together forever to make the ionic e compound LiCl. e + _ e e e e e e And they lived happily ever after Until the monkey started hallucinating. But that’s a story for another time. 9 9 PRACTICE SCHOOLOGY → Ionic bonding problem set https://tinyurl.com/pjfzpk v Learning Objectives Explain how nonmetals share electrons to complete their valence shell octet, resulting in the formation of a covalent bond. Identify single, double, and triple covalent bonds and draw electron dot diagrams for each. Describe how elements of different electronegativities can share electrons unequally, leading to the formation of a polar covalent bond. The Uneasy Friendship Between Fluorine And His Twin Fluorine was upset….. I have seven valence electrons, but I’d really like to. have eight so I can be like my.. nearest noble gas, neon. I should probably find something less electronegative to steal electrons from... Meanwhile, his other brother (also named. fluorine) was mad about the same thing…. Like my brother, I also want... to gain one more valence electron to be like my nearest noble gas, neon. I’ll find a good old fashioned metal and since it’s not.. electronegative, I can take its electron. There was only one problem: None of the metals wanted to hang out with either of the fluorine brothers. Fluorine said I look Fluorine says I’ve Fluorine said my eyes weird without a nose. got male pattern were freakishly far baldness apart Fortunately, this guy made an appearance… Hey fluorine and fluorine, I’ve got an idea!.. Fluorine #1, you’ve got seven valence electrons but nobody with low... electronegativity will hang out with you. Why not share one electron with your brother to make a covalent bond?.. And fluorine #2, if you share one of your electrons with.. your brother, then you’ll both have eight electrons next to you. You can pretend you’ve got eight valence electrons and.. that you’ve got the same number of valence electrons as neon!..... Well, I guess we can share.. our unpaired electrons...... I’ll try it out........ Let’s share those unpaired electrons!.................... So they moved together and shared their unpaired electrons.... I’ve got eight electrons next to me........ I do, too!...... And the two shared electrons made up a covalent bond!............ And they all lived happily ever after… Step 1: Count the total number of valence electrons. For a neutral molecule, add up the valence electrons for all of the atoms in the molecule. For a polyatomic ion, add up the valence electrons for all of the atoms and then add electrons (for negative charges) or subtract electrons (for positive charges), based on the overall charge of the ion. Step 2: Use single bonds to connect the atoms, forming a skeletal structure of the molecule or ion. A general rule is that the central atom is the one that tends to form the most bonds or the one that has the lowest electronegativity value. If one atom A is different than the rest of the atoms in the formula (e.g., a compound with a formula such as AX2, AX3, AX4, etc.), then atom A will be the central atom. Hydrogen (H) and fluorine (F) will never be the central atom. Each of these atoms only forms one bond. These atoms would be terminal atoms on the outer edge of the structure. Step 3: Add lone pairs of electrons around each terminal atom, in order to complete the octets for the terminal atoms. Skip this step for a hydrogen atom, because a hydrogen atom only needs a single bond to complete its valence shell. A hydrogen atom will never have lone pairs on it. If any valence electrons (from the total determined in Step 1) remain unused, place lone pairs of electrons on the central atom until the Lewis structure contains the total number of valence electrons from Step 1. Step 4: If the central atom has an octet, the structure is complete. If the central atom has less than an octet, move one or more of the lone pairs from the terminal atoms toward the center, forming additional bonds until each atom has a complete octet. https://bit.ly/3iGsL19 COMPLETE THE PROBLEM SET!!! Valence Shell Electron Pair Repulsion Theory (VSEPR) Believe it or not, this name actually describes this idea pretty well. Covalent bonds consist of pairs of electrons This probably isn’t news to anybody, but electrons all have negative charge: Micrograph of electron courtesy of Dr. T. Sammakia, University of Colorado, Boulder. In covalent molecules, there are a whole lot of electrons: See? Dots and lines everywhere! Because electrons all have the same charge, they repel each other Put another way, the lone pairs and covalent bonds in covalent compounds try to get as far away from each This means that even though other as possible. we’ve been drawing methane as a 2-D structure, it actually forms a 3-D structure where the electrons get as far away from each other as possible. Another example: Ammonia Likewise, the lone pairs and covalent bonds in ammonia repel each other, causing the real structure of ammonia to be three dimensional, as shown on the right. This is where the name for VSEPR comes from The outermost pairs of electrons in bonds and lone pairs repel each other. This explains the shapes of covalent compounds. And the shapes help to explain their reactivities. So, what do these shapes look like? Approx. Angle 180° 120° ~117° 109.5° ~107° ~104° Bonding domains = Things attached to central atom= lone pairs + atoms But what about double bonds and lone pairs? Double bonds, no. They don’t affect bond angles at all. Lone pairs, yes. All lone pairs tend to push a little harder on the other things bonded to an atom. As a result, the bond angles of the other atoms bonded to the central atom are less than A good thing expected. to know for a quiz. Let’s see what these examples actually look like: And that’s the abrupt end of the talk. Go do some practice stuff. Properties of Ionic and Covalent Compounds ionic crystal structure covalent molecular structure The structure of compounds determines their properties What a compound looks like on a microscopic level determines how it behaves on a macroscopic level. This is why it’s important that we know how ionic compounds and covalent Methane is a structures differ from one covalent compound another. The properties of ionic compounds reflects the way in which ions bond Keep in mind, there’s NEVER just one cation and one anion present. Instead, there are trillions of atoms giving electrons to each other, resulting in great big blocks of ions all held together by electrostatic interactions. 1) Ionic compounds are crystalline A crystal is when you have regular patterns of atoms or ions arranged to form a larger structure. As a result, ionic compounds, by definition, are crystalline solids. Covalent compounds, on the other hand, form molecules. Each molecule exists independently of other molecules. There is no covalent bonding between these molecules, so the molecules tend to move around all over the place under normal conditions. Ionic crystals act like LEGO blocks that are stuck rigidly to one another; covalent molecules behave more like Tylenol capsules that can easily move around one another. 2) Ionic compounds have high melting and boiling points caused by the very strong attraction between all of the cations and anions in the crystal. In order to melt an ionic compound, you need to overcome all of the energy that holds all of these ions together. This is called the lattice energy and it is very strong. Melting points of ionic Which of these are most likely ionic? compounds are typically in the hundreds to thousands of degrees Celsius. Because these molecules don’t stick well to each other (weaker bonds), covalent compounds have the following properties: Covalent compounds have low melting and boiling points because they melt differently than ionic compounds Covalent compounds melt when the molecules can move away from each other. No bonds are broken, and simply moving them away from each other requires little energy. 3) Ionic compounds are hard and brittle Why are they hard? It takes a lot of energy to move the ions in relation to one another, so instead of moving and bending, they just stay in place. Why are they brittle? If you do succeed in moving the ions, they probably won’t be lined up in a stable configuration anymore. This causes the ions to repel each other and crack the crystal apart. Covalent Compounds Are Soft And Squishy OK… this might be a bit of an overstatement. After all, ice isn’t particularly soft or squishy. However, because covalent molecules don’t lock in place next to each other, they’re usually not as hard and brittle as ionic compounds because they can deform more easily. Consider rubber (covalent) vs. salt (ionic) 4) Ionic compounds tend to be soluble in water Ionic compounds frequently dissolve in water because water has partial positive and negative charges that can grab the ions and pull them apart. Covalent Compounds Are Usually Less Soluble In Water Than Ionic Compounds Water molecules can easily grab onto the cations and anions in ionic compounds and pull them apart. Covalent compounds don’t very often have areas with such strong electric charge that water molecules can grab onto. As a result, water molecules don’t do a good job of dissolving covalent compounds. 5) Ionic compounds are electrolytes if you dissolve them or melt them In order for something to conduct electricity, electric charges need to move around. This can be done in two ways: Metals conduct electricity by allowing the movement of electrons via electron sea theory. Melted or dissolved ionic compounds conduct electricity because the ions are no longer locked in place! Note: Ionic crystals don’t conduct electricity. They only conduct if melted or dissolved. Covalent Compounds Don’t Conduct Electricity In order to conduct electricity, either electrons need to move from one place or another (as in metals) or ions need to move from one place to another (as in melted/dissolved ionic compounds). Since covalent compounds don’t have ions or delocalized bonding, they don’t conduct. 6) Ionic compounds rarely burn For something to burn, it needs to contain both carbon and hydrogen. Because both elements are equally far away from the nearest noble gas, they don’t really want to gain or lose electrons to form ions at all. As a result, ionic compounds usually don’t burn because they just don’t have the right elements Firefighters like to goof off when people try to start fires with ionic to do so. compounds. Covalent Compounds Sometimes Catch Fire Unlike ionic compounds, which hardly ever contain carbon and hydrogen, many covalent compounds do. As a result, many covalent compounds are flammable. Summary of ionic compound properties Ionic compounds have high melting and boiling points because it takes a very large amount of energy (i.e. high temperature) to pull all of the cations and anions apart from each other. Ionic compounds are hard because the ions are tightly held in place. Ionic compounds are brittle because any movement of the ions will cause ions with similar charge to come into contact with each other. Ionic compounds conduct electricity when melted or dissolved because electricity can be conducted through moving ions (but not stationary ones). Ionic compounds don’t burn because carbon and hydrogen don’t generally form ionic compounds. In summary: Ionic compounds tend to be hard, brittle, and have high melting and boiling points because the ions are locked tightly in place. Covalent compounds tend to be less rigid and have lower melting and boiling points because the molecules are easy to separate – they don’t interact with each other much. This is an enzyme or something. But definitely covalent. Naming Ionic Compounds & Covalent Molecules This one’s named Bob. Writing formulas of ionic compounds MUST CONSIST OF A METAL AND A NONMETAL 1) Write the “base name” of the compound. 2) Check to see if you need to add a Roman numeral. 3) If you do, figure out what the Roman numeral is and then write it. These steps are both handy and easy to do, but are almost certainly incomprehensible without more information. Let’s see how to do it… Step 1: Write the “base name” of the compound The base name consists of two words: The first word is the name of the cation. It’s just an element name. The second word is the name of the anion. This comes from one of two places: It’s the name of the second element with “-ide” at the end (chloride, oxide, etc) It’s the name of a polyatomic ion, which contains more than one element. These need to be memorized. Examples of how to write the base name: NaF: sodium fluoride Pb(OH)2: lead hydroxide CaS: calcium sulfide NH4NO3: ammonium nitrate Calcium sulfide undergoes ”stimulated photoluminescence”, whatever that is. Step 2: See if you need to add a Roman numeral Some cations have more than one possible positive charge. These include all of the elements except for the metalloids/nonmetals and the other elements outlined in purple below. Step 2: Using our examples NaF: sodium fluoride Na does not need a Roman numeral! Pb(OH)2: lead hydroxide Lead does need a Roman numeral CaS: calcium sulfide Ca does not need a Roman numeral NH4NO3: ammonium nitrate NH4 does not need a Roman numeral Step 2 (continued): Roman numerals If, after checking the Of our examples, NaF, Pb(OH)2, periodic table, you decide CaS, and NH4NO3, only Pb(OH)2 that you don’t need a needs a Roman numeral. For Roman numeral, STOP. IN the rest, you have your answer! THIS CASE YOU ARE DONE! IF YOU CONTINUE, THE ANSWER WILL BE WRONG! JUST WALK AWAY! Only if you determine that you need a Roman numeral should you go to step 3. Step 3: Determining the Roman numeral To figure out the Roman numeral needed, use this handy equation: (number of anions)(charge on anion) Roman numerals: Roman numeral = number of cations I, II, III, IV, V, VI, VII What does this mean? Vertical lines refer to the fact that the cation charge is positive (absolute value of calculation) The number of anions is usually clear. However, for polyatomic ions, this is only greater than one if you see parenthesis around it. The charge on the anion is figured out either by looking at the periodic table (if it’s a single atom) or looking it up on the back of the periodic table (if it’s a polyatomic ion). The number of cations is the number to the bottom right of the cation formula. Step 3: For our example, Pb(OH)2 (number of anions)(charge on anion) Roman numeral = number of cations (2)(-1) Roman numeral = 1 = 2, which is II in Roman numerals Fun fact: lead (II) Compound name = lead (II) hydroxide has no hydroxide common uses! Let’s do some more examples! MgO MnS Cr(SO4)2 AgNO3 K3N ZnS Colloidal silver is thought to be a good antibiotic, so some people drink solutions of it. This causes them to get argyria, a harmless, irreversible, and hilarious condition that makes them turn blue. Writing formulas of covalent molecules MUST CONSIST OF NONMETALS ONLY 1) Write the names of the elements in the order they’re shown. 2) Add prefixes to these names to indicate how many of each atom is present in the compound. Write the names of the elements in the order they’re shown. For example, if you see PF₃, write the words “phosphorus fluoride”. There will be no polyatomic ions or Roman numerals – just those two words. Add prefixes to these names to indicate how many of each atom is present in the compound. In the case of PF₃, you can see that there is one phosphorus and three fluorine atoms. As a result, we don’t need a prefix for phosphorus (we assume that if there’s no prefix there’s just one of them) Use the “tri-” prefix for fluoride to indicate that there are three atoms of it in the compound. Put it together, and you’ve got the name “phosphorus trifluoride”, which literally means “one phosphorus atom and three fluorine atoms.” Which is a pretty accurate description of PF₃. For those of you who don’t remember your prefixes, here they are 1 atom of an element = mono (only use for oxygen if it’s the second atom shown!) 2 atoms of an element = di 3 atoms of an element = tri 4 atoms of an element = tetra 5 atoms of an element = penta 6 atoms of an element = hexa 7 atoms of an element = hepta 8 atoms of an element = octa 9 atoms of an element = nona 10 atoms of an element = deca Excepetions That all seems pretty straightforward, except for maybe the part with the “mono” in it. My little note above means that the only time you ever use the “mono” prefix is if oxygen is the second atom in the compound and there is only one atom of it. As a result, we name these compounds: CO = carbon monoxide OF₂ = oxygen difluoride This is the only exception that you’re going to run into for covalent compounds, so don’t worry about it too much. Other exceptions (Common names) Compounds to memorize: water is H₂O, ammonia is NH₃, methane is CH₄ Diatomic elements: There are seven elements on the periodic table that are diatomic, which means that if they’re in their pure elemental form they have a “2” subscript written after them. These include hydrogen (H₂), nitrogen (N₂), oxygen (O₂), fluorine (F₂), chlorine (Cl₂), bromine (Br₂), and iodine (I₂). Phosphorus is P₄ and sulfur is S₈. And now let’s do some more examples! This poor woman’s doctor prescribed her colloidal silver nose drops when she was a child. Needless to say, she’s extremely angry about this. Polarity in Covalent Compounds When covalent compounds have partial charges What if covalent molecules have attractive and repulsive forces? For example: Oxygen has an electronegativity of 3.44. Hydrogen has an electronegativity of 2.20. The difference in electronegativity is not enough to cause electrons to transfer, but it does cause the electrons in the covalent bonds to spend more time around oxygen than hydrogen. This leads to this… O-H Let me explain… O-H O-H O-H The arrow points toward the more electronegative atom as a reminder that the electrons spend more time there. An aside: The arrow shown here is referred to as a “dipole.” This term will come in handy later. O-H Putting it all together, this is just another way of saying: “Because oxygen has higher O-H electronegativity than hydrogen, the electrons in the O-H bond spend more time around oxygen than hydrogen.” When any two atoms with different electronegativities bond with one another, the electrons will spend more time around the more electronegative one and less time around the less electronegative one. Definitions SOMETHING IS POLAR WHEN THERE IS AN UNEVEN DISTRIBUTION OF ELECTRONS SIMPLE TERMS: THERE IS A LARGE ELECTRONEGATIVITY DIFFERENCE BETWEEN THE ATOMS. The larger the difference, the more polar SOMETHING IS NONPOLAR WHERE THERE IS AN EVEN DISTRIBUTION OF ELECTRONS SIMPLE TERMS: THERE IS A SMALL ELECTRONEGATIVITY DIFFERENCE BETWEEN THE ATOMS Use your periodic trends to determine electronegativity of both atoms Subtract the smaller electronegativity from the larger one to find the difference. For example, if we're looking at the molecule HF, we would subtract the electronegativity of hydrogen (2.20) from fluorine (3.98). 3.98 - 2.20 = 1.78. It would be considered a polar covalent bond Can entire covalent molecules be polar? How can covalent compounds be polar? We saw that polar covalent bonds are formed when the electrons spend more time around one atom in the bond than another. Polar covalent molecules are formed when the electrons spend more time in one part of the molecule than another. How can you tell if a molecule is polar? Draw the molecule and check to see if all of the things connected to the central atom are the same. If they are, then the molecule is nonpolar (i.e. it has an equal sharing of electrons). If not, then it’s polar (unequal sharing). As a reminder, “things” refer to atoms and lone pairs. The number of bonds is NOT what matters. Two examples: H2O and CO2 Water has two different “things” next to each other Since hydrogen atoms are different from lone pairs of electrons, we can conclude that water is a polar covalent molecule The oxygen atom is more This diagram shows two polar O-H bonds and that the electronegative than the electrons in the molecule hydrogen atoms, the dipole spends time around oxygen arrow points toward the oxygen more than hydrogen. atom and the little δ signs are located on the side of the molecule with + or - charge. Carbon dioxide has only one type of thing stuck to the central atom: In this structure, we can see that carbon has two oxygen atoms stuck to it. Because these atoms are the same, the molecule is nonpolar and there’s no need to draw any of those δ things or an arrow. Why does this work? Symmetry If molecules are symmetrical, any dipoles caused by polar covalent bonds will cancel each other out. Some more examples NH3 BF3 CH4 Methane in one of its more energetic moments. Some more examples SO2 H2 S NaCl Comparing the polarity SO2 CO2 The larger the electronegativity difference, the more polar the molecule is In conclusion Bonds are polar if one atom is more electronegative than another. Molecules are polar if they’re asymmetrical Intermolecular Forces Vocab Intramolecular forces: “Intra” means “within”, so these forces hold the things within molecules together. ○ Example: ionic and covalent bonds Vocab Intermolecular forces: These are forces between two different covalent molecules ○ Intermolecular forces have varying strengths, weaker than real bonds ○ cannot be thought of as locking things in place, but rather keeping things sort of loosely attached. Inter Vs Intra Molecular Forces intrA-molecular = within a molecule Intermolecular forces- interactions between molecules Inter-molecular = intermission There are three big types of intermolecular forces: London dispersion forces Dipole-dipole Hydrogen bonding London Dispersion Forces These are forces that take place when a partial charge on one nonpolar molecule is attracted to the partial charge on another nonpolar molecule. As our story starts, two nonpolar molecules are just chilling. They aren’t paying any attention to each other at all. London Dispersion Forces And then for no particular reason at all, random movements of electrons within the molecule on the left cause the electrons to shift, making the side with more electrons partially-negative and the side without them partially-positive. London Dispersion Forces This imbalance in electrons causes the electrons in the second molecule to compensate by shifting its electrons, too. As a result, the electrons nearest the partial negative side of the first molecule move away (they have the same charges as the electrons on the other molecule), making the second molecule polar, too. This causes the molecules to attract in the same method as molecules experience dipole-dipole forces. London Dispersion Forces This force doesn’t last for long. Remember, it was the random movement of electrons that caused this charge imbalance in the first place, so it’s the random movement of electrons that eventually undoes it and changes everything back to the initial state. London dispersion forces are very weak. The more electrons, the stronger the LDF Dipole-Dipole Dipole-dipole interactions occur between molecules that have a permanent dipole due to them being polar molecules. Dipole-dipole interactions are similar to LDFs, except that the dipoles are permanent and so the attractions are generally greater. The partially positive end of one molecule is attracted to the partially negative end of a different molecule, and so on throughout the system. The more polar the molecules, the greater the attraction. Dipole-Dipole Forces One side of polar molecules has partially-positive charge while the other side has partially-negative charge. As a result, if you put two polar molecules near each other, the partial positive side of one will be attracted to the partial negative side of the other: ○ Because + and – attract, this force helps to keep the HCl molecules stuck near each other. The partial positive charge on the Cl on the HCl on the left is attracted to the partial positive charge of the H on the HCl on the right. Hydrogen Bonding - not a bond Hydrogen bonding is an IMF that occurs in molecules where Hydrogen is covalently bonded to F, O, or N. (Hydrogen bonding is FON!) This difference in electronegativity creates a large molecular dipole, and this dipole causes a strong attracti to the dipole on the molecules surrounding it. 1. NO 2. YES 3. NO 4. YES 5. NO Rubbing alcohol vs water Rubbing alcohol vs water LDF, DIPOLE-DIPOLE, LDF, DIPOLE-DIPOLE HYDROGEN BONDING Why do we care? This causes differences in the properties of polar molecules and nonpolar molecules. How polar covalent compounds differ from nonpolar covalent compounds: Polar molecules have higher melting and boiling points than nonpolar molecules because of the dipole-dipole attraction holding them together. Nonpolar molecules have lower melting and boiling points because of the weak london dispersion forces holding them together. Important note: When polar covalent compounds melt, there is still no breaking of covalent bonds! Example: Chloromethane Methane Polar covalent molecule Nonpolar covalent molecule Melting point: -97o Celsius Melting point: -182o Celsius Boiling point: -24o Celsius Boiling point: -162o Celsius Polar molecules are more soluble in water than nonpolar molecules Because polar molecules have partial charges, water molecules can grab them and pull them apart.