Chemical Bonding PPT
Document Details
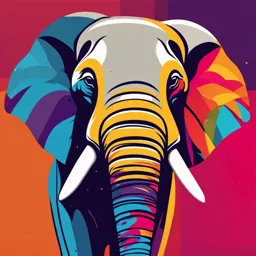
Uploaded by HumourousBarbizonSchool
Ramon Teves Pastor Memorial Dumaguete Science High School
Tags
Summary
This presentation provides an introduction to chemical bonding. It explains the different types of bonds, including ionic, covalent, and metallic, and discusses their properties. It also covers concepts such as ionization energy and electronegativity. The material is suitable for an undergraduate chemistry course.
Full Transcript
Introduction to Chemistry Chemical Bonding Chapter 4 Copyright © The McGraw-Hill Companies, Inc. Permission required for reproduction or display. Chemical Bonding W...
Introduction to Chemistry Chemical Bonding Chapter 4 Copyright © The McGraw-Hill Companies, Inc. Permission required for reproduction or display. Chemical Bonding Why do atoms form bonds? The most stable arrangement of electrons in an atom is to have either a filled valence shell or to have 8 valence electrons (octet rule). Atoms obtain this stable electron arrangement by sharing, gaining or losing electrons – which is what creates chemical bonds. Chemical Bonding Types of Chemical Bonds There are three major types of chemical bonds: ionic bonding covalent bonding metallic bonding The type of bond formed depends on the ionization energy and electronegativity of the atoms involved Chemical Bonding Ionization energy (IE) = energy required to remove one valence electron. metals have LOW ionization energies non-metals have HIGH ionization energies Electronegativity (EN) = measure of the tendency for an atom to “steal” an electron from another atom within a chemical bond. Follows the same trends as electron affinity: metals have LOW electronegativities non-metals have HIGH electronegativities The Electronegativities of Common Elements Ionic Bonds Ionic Bonding The Ionic Bond When an atom with a high EN is placed near an atom with a low IE, the first atom may “steal” an electron from the second atom. The atom that gives up the electron becomes a positively charged cation, and the atom that gains the electron becomes a negatively charged anion. An ionic bond is the “electrostatic” attraction formed between the oppositely charged cation and anion. Ionic Bonding This electron transfer can only occur if the first atom’s electronegativity is quite high, (that is, it readily steals electrons) and the second atom’s ionization energy is very low (that is, it readily gives up an electron)! Thus, this electron transfer typically occurs between a metal (low IE) and a non-metal (high EN) + metal (low IE) non-metal (high EN) oppositely charged cation and loses electron gains electron anion attract = ionic bond Ionic Bonding Ionic Bonding: Summary An ionic bond is the electrostatic attraction formed between (+) cations and (-) anions. The cations and anions are formed when a non- metal (high EN) steals an electron from a metal (low IE). This transfer of electrons gives each atom a filled valence shell (stable octet) of electrons. Ionic Bonding Arrangement of ions in ionic compounds Ionic compounds do not form individual “molecules,” composed of just two or three atoms. Instead, ionic compounds are composed of literally billions of cations and anions electrostatically attracted to each other. The empirical formula for an ionic compound is just the simplest RATIO of the cations to the anions in compound. Ionic Bonding The regular, repeating arrangement of ions in an ionic compound is called the crystal lattice. expanded view of the actual crystal lattice crystal lattice of NaCl structure of NaCl Ionic Bonding Factors that Determine the Structure of an Ionic Compound’s Crystal Lattice The charge of the ions: this determines the ratio of cations to anions The size of the ions: larger ions will not fit in the same arrangement as smaller ions will. Ions are arranged within the lattice in such a way as to maximize the separation of like charged ions, and minimize the separation of un-like charged ions. (Like charges repel and opposite charges attract.) Ionic Bonding Properties of Ionic Compounds Definite crystalline structure High melting points Brittle as solids Most dissolve in water Conduct electricity only when in the molten state or when dissolved in water. Ionic Bonding Definite Crystalline Structure: This is explained by the factors already discussed concerning the crystal lattice structure: charge of ions, size of ions, and attraction/repulsion of ions. Ionic Bonding High Melting Points: To melt a substance requires that the particles of the substance be separated, which requires energy to accomplish. In ionic compounds, each particle is attracted to multiple other particles of opposite charge in a three-dimensional lattice network. Melting ionic compounds requires breaking multiple ion-ion attractions, which requires more energy = higher melting pt. this cation is held in place by attractions to three anions, all three of which must be overcome in order to melt the compound. Ionic Bonding Brittle as Solids: If you “hammer” on an ionic crystal, it shatters. Any attempt to distort the crystal lattice causes the “layers” to shift, which causes like-charged ions to become aligned. The resulting repulsive forces shatters the crystal. force applied to crystal like charges crystal shatters due to become aligned repulsive forces between like-charged ions Ionic Bonding Dissolve in Water: As we shall see later in this chapter, water is a polar molecule. Polar molecules are molecules which have a small degree of charge separation, so that one end of the molecule is slightly negatively charged, and the other end is slightly positively charged. red = partial negative blue = partial positive charge H charge O H water molecule Ionic Bonding Dissolve in Water: continued The partial positive charge on the hydrogen atoms in water are attracted to the anions in an ionic compound; the partial negative charge on the oxygen atom in water are attracted to the cations in an ionic compound. The water molecule is able to literally “pluck apart” the ionic compound, and carry the ions off into the solution. + = water = anion ( ) = cation (+) + Ionic Bonding Conduct Electricity when Molten or Dissolved in Water An electric current is made up of electrons in motion. In order for a current to flow through a substance, there must be an electric field, produced when charged particles are separated. (The electric field is what “pushes” the electrons along.) When an ionic compound is in the solid phase, there is no separation of charges – and no current can flow. When the compound is dissolved in water or in the molten (liquid) phase, the ions are separated, which creates the electric field and allows the current to flow. Covalent Bonds N N - - - - O C O O C O O C O O O O - - Covalent Bonding The Covalent Bond If two atoms both have relatively high, but similar electronegativities, they can obtain a stable octet of electrons by sharing electrons between them, rather than transferring electrons from one atom to another. This sharing of electrons by overlapping orbitals between two atoms is called a covalent bond. Covalent bonds typically form between two non- metals, or between a metalloid and a non-metal. We can now officially define what a molecule is… A molecule is the name given to two or more atoms which are bonded together by covalent or polar covalent bonds. The atoms may be different elements, or they can all be the same element. Polar Covalent Bonding If an ionic bond is a complete transfer of electrons, and a pure covalent bond is an equal sharing of electrons, then we can say a polar covalent bond is an un-equal sharing of electrons. If the two atoms have nearly identical EN, then the bond is pure covalent. If there is a large difference in EN between the two bonded atoms, then an ionic bond forms. When there is a moderate difference in EN between the two bonded atoms, a polar covalent bond forms Covalent Bonding When the atoms are bonded by polar covalent bonds, the shared electrons are shifted towards the atom with the greater electronegativity. This electron shift creates charge separation within the molecule. This charge separation can create a polar molecule in which one end of the molecule has a partial positive charge (+) and the other end has a partial negative charge (-). + A — B If B is more EN than A, the electrons are shifted away from A and towards B. We represent this shift with an arrow, pointing towards the partial negative side of the molecule, with a cross-bar at the partial positive end. Covalent Bonding Not all molecules with polar covalent bonds are polar molecules. In some cases, the electrons are pulled in opposing directions, which “cancels out” the shift, so that no charge separation occurs: polar molecule: arrows do not cancel each other out + nonpolar: arrows cancel each other out Covalent Bonds Because molecules can exist as individual, isolated units, many of the properties of the compound often depend on the nature of the attractive forces that exist between two or more molecules. Note carefully that these attractive forces are NOT covalent bonds. We will investigate the nature of these so-called “intermolecular” attractive forces in more detail later on… Covalent Bonds Properties of Molecular Compounds Molecular compounds have lower melting points than ionic compounds. Molecular compounds are poor conductors of electricity in any phase. Molecular compounds have a wide range of solubility in water – some dissolve easily in water, some do not dissolve at all. Covalent Bonds Low Melting Points The bonding within a molecule is a covalent bond between two atoms – however, there are only very weak attractions between separate molecules. As a result, it does not take a lot of energy to separate two molecules (melting). weak or no attractions Molecules are easily between molecules… separated = low melt pt. Covalent Bonds Poor Conductors of Electricity Recall that, in order to conduct a current of electricity, you must have charged particles that are some distance apart to create an electric field. Molecules are made up of neutral atoms. By sharing the electrons, neither atom has gained or lost electrons to become ions, even when the molecules are separated. Thus, there are no charged particles with which to create an electric field, and so they are non- conductors. Covalent Bonds Solubility in Water Recall that water is a polar molecule, in which there is a charge separation within the molecule so that one end is partially positive and other end is partially negative. Compounds that are also polar molecules can form attractions to water molecules, and they dissolve, much like ionic compounds. Non-polar molecules cannot form attractions to water, and as a result, they do not dissolve in water. we will have lots more to say on this topic later on… 9.4 F N F F Lewis Structures - - - O C O - O C O O C O O O O - - Lewis Dot Symbols for the Representative Elements and Noble Gases 9.1 RULES FOR DETERMINING THE LEWIS STRUCTURE OF MOLECULES Determine the TOTAL number of valence electrons supplied by ALL the atoms in the compound. Determine which element is the central element. This will be the element that is the LEAST electronegative element of those present in the compound, with the exception of hydrogen. Hydrogen can NEVER be the central element, since H can never form more than one bond. Arrange the other atoms around the central atom. Lewis Structure Rules Example: Consider the molecule CHF3 CHF3 = 1C + 1 H + 3 F = 1(4) + 1(1) + 3(7) = 26 valence electrons The least electronegative element is H, but H cannot be the central atom; carbon has the next lowest EN so the central atom will be carbon. Arranging the other elements around C we write: F the arrangement of the outer atoms is arbitrary – H C F typically, if the molecule has 3 atoms we place them in a straight line, for 4 atoms we use a T-shape, and for 5 F atoms we use a + sign pattern as shown here. Lewis Structure Rules 4. Form a single bond between the central element and all the other elements in the compound. Each single bond requires 2 electrons. Subtract the number of e- used in making the single bonds from the total number of electrons available, which you determined in step #1. F It requires 8 electrons to form the 4 : H : C: F single bonds needed to attach the outside elements to the carbon atom F : This leaves: (26 — 8) = 18 electrons Lewis Structure Rules 5.IF the number of electrons needed to make the single bonds is LESS than the total number of electrons available, then place electrons, IN PAIRS, around the outside elements until their valence requirements are met. IF the number of e- needed for this equals the number of e- available, you are finished add electrons in pairs around each F : : : F: atom until each has a total of 8 electrons; none are added to H because H : C : F: : : it already has 2 electrons. : F: : : This used an additional 18 electrons, which is all that were left – we are done. This is the Lewis structure for CHF3. Lewis Structure Rules Some definitions used with Lewis structures : : :F: : : H : C:F: :F: : : One shared electron pair makes a single covalent bond. Unshared electron pairs are also called “lone pairs.” These electrons are “owned” by the individual atom. They are not part of the covalent bond. Lewis Structure Rules We often show single bonds as a single line, rather than as 2 dots. We still show all the lone pairs as dots, however. : : : :F: :F: : : : : H : C:F : H C F: :F: :F: : : : Lewis Structure Rules Example: consider the molecule OF2 total number of valence e- = 2(7) + 1(6) = 20 e- Arranging the elements with O as the central atom and forming the 2 bonds required to attach the F’s to the O requires 4 electrons. This leaves (20 – 4) = 16 e- : : :F :O :F : : : Next we place 6 e- around each F to fill their valence needs. This uses 12 e-. So far we have used 4 + 12 = 16 electrons. This leaves us with 4 e- left over: (20 – 16) = 4 Lewis Structure Rules 6.IF there are any electrons left over after filling the valence needs of the outer atoms, add these extra electrons to the central atom, in pairs. If the valence requirements of all atoms has been met at this point, you are finished. : : : : : : :F :O :F : :F O F : : : : : : : When we add the 4 remaining electrons to the central oxygen atom (in pairs), we have now met the valence needs for every atom in the compound. We are done. Lewis Structure Rules Example: consider the molecule CH2O total number of valence e- = 1(4) + 2(1) + 1(6) = 12 e- Arranging the elements with C as the central atom and forming the 3 bonds required to attach the H’s and the O to the C requires 6 electrons. This leaves (12 – 6) = 6 e- : :O : : H :C:H The 2 H’s now have 2 e- each, so their valences are filled. The remaining e- are placed on the oxygen atom. At this point, the oxygen’s valence needs are met. However, the carbon only has 6 electrons in its valence, and it needs 8 – and we are out of electrons! Lewis Structure Rules 7.If there are insufficient electrons to meet the valence requirements of every element, you must share 2 or more pairs of electrons with the central atom. shift this pair of electrons down : :O : so that there are now 4 electrons : being shared between carbon H :C:H and oxygen… : :O Now every element has a filled octet (or a filled shell, which only :: H :C:H requires 2 e- in the case of H) Lewis Structure Rules When an atom shares 4 electrons (2 pairs) we say the bond is a double bond. We represent a double bond as 2 lines. : : : :O O :: H :C:H H C H Double bonds are stronger than single bonds. Lewis Structure Rules Example: Consider HCN The compound contains 1 + 4 + 5 = 10 e-. We form the bonds needed to attach the H and N to the central C atom. Next we place the remaining 6 e- around the nitrogen atom. : : H: C : N: To meet the valence needs of the carbon, we will have to shift TWO pairs of electrons from the nitrogen, so that C and N share 3 electron pairs. : : H: C : N: H : C : :: N : Lewis Structure Rules When an atom shares 6 electrons (3 pairs) we say the bond is a triple bond. We represent a triple bond as 3 lines. H : C : :: N : OR H C N: Triple bonds are stronger than double bonds. Example: Write the Lewis structure of nitrogen trifluoride (NF3). Step 1 – N is less electronegative than F, put N in center Step 2 – Count valence electrons N = 5 and F = 7 5 + (3 x 7) = 26 valence Step 3 – Draw single bondselectrons between N and F atoms and complete octets Step 4 - Add on outside remaining twoFelectrons atoms. This uses 24atom. to central e- Check, do all atoms have filled octets? F N F N F F F F Lewis Structure Rules Resonance When drawing out Lewis structures, you may encounter a situation where a double bond is needed, but there are two or more different atoms that could donate the extra electrons. For example, consider the molecule O3 (ozone). When you draw out its Lewis structure, you reach this structure after putting in all the available electrons: The question becomes, which of the two O O O oxygens will donate a second e- pair with the central atom with which the required double bond can be made? Does it make any difference? Lewis Structure Rules The answer turns out to be – BOTH of the oxygens will donate electrons. If only one did, that would imply there is something unique about that particular O—O bond, when in fact, all O—O bonds should be identical. they’re I’m an oxygen, an oxygen, wouldn’t you like to be an oxygen, too? Lewis Structure Rules The problem is, how do we show that both oxygens are simultaneously donating electrons? This would require that the oxygen atoms share 3 electrons – that is, we need to show there are two “1½ bonds” to the central atom… O O O O O O ?? ?? Lewis Structure Rules We show this by drawing both possible Lewis structures, in which the central oxygen forms a double bond with one oxygen, and then the other. We use a double arrow between the two to show that the true structure is a blending of the two possible Lewis structures: O O O O O O The double bond is NOT switching back and forth between the left and right hand oxygens! This “double” Lewis structure is called a resonance structure. Lewis Structure Rules When your Lewis structure calls for resonance structures, you must show all possible resonance structures to receive full credit! Shown below are two compounds – one requires resonance and the other does not. Can you tell which one requires resonance? NOT resonance – atoms are not the same! identical atoms – this requires resonance Some “Special Case” Types of Covalent Bonding Some molecular compounds do not exhibit the properties typical of most molecules. Some of these special molecules have extremely high melting and boiling points; some have an actual electric charge to them. The two types of special molecules are: network solids polyatomic ions Some “Special Cases” Network Solids Network solids are a type of crystal in which every atom is covalently bonded to its neighbors in a vast, complex interconnected “network.” Quartz crystals (SiO2) and diamonds are examples of these network solids. Some “Special Cases” Because every atom is covalently bonded to its neighbor, network solids can be thought of as giant “macro- molecules.” In order to melt network solids, you must break multiple covalent bonds, much as we have to overcome multiple ion-ion attractions in ionic solids. As a result, network solids tend to have extremely high melting points, and tend to be very hard. diamond: melting point = 3550 oC diamond is the hardest substance known Polyatomic Ions Polyatomic ions are groups of atoms covalently bonded together, which have a net charge. Most polyatomic ions are anions, in which the group of atoms picks up one or more electrons to fill the valence needs of one of the atoms in the group. cyanide anion (CN-) nitrate anion (NO3-) sulfate anion (SO42-) Common Compounds with Polyatomic Ions calcium carbonate CaCO3 antacids, limestone sodium hydroxide NaOH drain cleaner calcium sulfate CaSO4 plaster of paris sodium bicarbonate NaHCO3 baking soda sodium hypochlorite NaClO Clorox bleach Metallic Bonding Metallic Bonding A metallic bond can be thought of as a mobile “sea” of delocalized electrons, which are mutually attracted to and “shared” by a cluster of metal cations. + + + + + + The (-) electrons act as + + + the “glue” that holds the + + + + + (+) cations together. + + + + + + Metallic Bonding Properties of metals Metals are good conductors of heat & electricity Metals are malleable and ductile Metals are lustrous Our model of the metallic bond can be used to explain why metals have these properties. Metallic Bonding Metals are Good Conductors Recall that to be a conductor of electricity requires that there be charges that are separated in order to create an electric field. There are (+) cations and (-) electrons that are separated in metallic bonds, which creates the necessary electric field. In addition, the mobile “sea” of electrons themselves are able to move easily = current of electricity. Heat is a measure of the transfer of kinetic energy between particles. The mobile e- are able to collide with other e- and cations, transferring kinetic energy as they do so. Thus, metals conduct heat very well. Metallic Bonding Metals are Malleable and Ductile Malleability and ductility requires that the bonding between atoms be very “flexible” so that, even when the substance is distorted, the atoms are still bonded. When a force is applied, the cations are free to move – as long as there are some force e- between the cations, the atoms are still bonded together… + + + + + + + + + + + + + + + + + + + + Metallic Bonding Metals are Lustrous An object that appears lustrous or shiny is reflecting or emitting multiple photons of light with very similar, but slightly different, energies. The delocalized e- in metals move between shells that are close together in energy levels. This e- shift produces photons of similar energy = “lustrous” quality of the metal. Larger shells are close together in energy states, so the photons emitted are also similar in energy – this makes it shiny.