Chemical Bonding PDF
Document Details
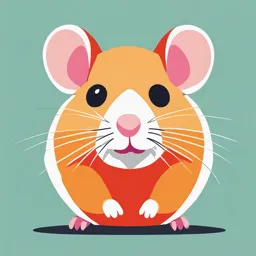
Uploaded by SuitableParrot494
Tags
Summary
This document provides a lecture on chemical bonding. It discusses the different types of chemical bonds, including ionic and covalent bonds. It details the concepts of valence, valence electrons, and bonding and non-bonding electrons.
Full Transcript
Lecture – 11 Chemical Bonding Chemical Bond: A chemical bond is defined as a force that acts between two or more atoms to hold them together as a stable molecule. There are mainly three (03) types of Chemical bonds: 1) Ionic or Electrovalent bond 2) Coval...
Lecture – 11 Chemical Bonding Chemical Bond: A chemical bond is defined as a force that acts between two or more atoms to hold them together as a stable molecule. There are mainly three (03) types of Chemical bonds: 1) Ionic or Electrovalent bond 2) Covalent bond 3) Co-ordinate covalent bond There are some other types of bond – Metallic bond, Hydrogen bond, Polar covalent bond. Valence: The term valence (or, Valency) is often used to state the potential or capacity of an element to combine with other elements. ―The number of bonds formed by an atom in a molecule is called valence‖ Example, Valence of H is 1 Valence of O is 2 Valence of N is 3, 5 etc. Valence Electrons: The electrons in outer energy level of an atom are the ones that can take part in Chemical Bonding. These electrons are, therefore, referred to as the valence electrons. Na (11) = 2, 8, 1 Valence electron = 1 Cl (17) = 2, 8, 7 Valence electron = 7 Bonding and Non-bonding Electrons: The valence electrons actually involves in bond formation are called bonding electrons. The remaining valence electrons still available for bond formation are referred to as non-bonding electrons. Here only one pair electrons are bonding electrons; rests of the electrons are non-bonding electrons. Electronic Theory of Valence: Lewis and Kossel worked independently using the knowledge of Bhor model to explain ―why atoms joined to form molecule‖. All the atoms have a tendency to become stable as like as noble gas. In 1916, they gave the electronic theory of valence. ―In chemical bond formation, atoms interact by losing, gaining or sharing of electrons so as to acquire a stable noble gas configuration.‖ Each noble gas, except Helium (He), has a valence shell of eight electrons. While atoms of noble gases possess a stable outer shell of eight electrons or octet, atoms of most other elements have incomplete octets. Thus, the electronic theory of valance could well be named as the Octet Theory of valence. It may be stated as: ―Atoms interact by electron transfer or sharing, so as to achieve the stable outer shell of eight electrons.‖ ―The tendency for atoms to have eight electrons in the outer shell is also known as the Octet Rule or the Rule of Eight‖ Since Helium (He) has two electrons in the outer shell, for H and Li another rule is apply known as Rule of Two. 1) Ionic Bond: The electrostatic attraction between the cation ( + ) and anion ( – ) produced by the electron transfer constitutes an Ionic or Electrostatic bond or Electrovalent bond. The compounds containing such a bond are referred to as Ionic or Electrovalent Compounds. Let us consider a general case when an atom A has one electron in the valence shell and another atom B has seven electrons. A has one electron in excess and B has one electron short than the stable octet. Therefore, A transfers an electron to B and in this transaction both the atoms acquire a stable electron-octet. The resulting positive ion (cation) and negative ion (anion) are held together by electrostatic attraction. CONDITIONS FOR FORMATION OF IONIC BOND The conditions favourable for the formation of an ionic bond are : (1) Number of valence electrons The atom A should possess 1, 2 or 3 valence electrons, while the atom B should have 5, 6 or 7 valence electrons. The elements of group IA, IIA and IIIA satisfy this condition for atom A and those of groups VA, VIA, and VIIA satisfy this condition for atom B. (2) Net lowering of Energy To form a stable ionic compound, there must be a net lowering of the energy. In other words energy must be released as a result of the electron transfer and formation of ionic compound by the following steps : (a) The removal of electron from atom A (A – e– → A+) requires input of energy, which is the ionization energy (IE). It should be low. (b) The addition of an electron to B (B + e– → B – ) releases energy, which is the electron affinity of B (EA). It should be high. (c) The electrostatic attraction between A+ and B – in the solid compound releases energy, which is the electrical energy. It should also be high. If the energy released in steps (b) and (c) is greater than the energy consumed in step (a), the overall process of electron transfer and formation of ionic compound results in a net release of energy. Therefore, ionisation of A will occur and the ionic bond will be formed. For example, in case of formation of sodium chloride (NaCl), we have The net energy released is 187 + 85 – 119 = 153 kcal. Since the overall process results in a lowering of energy, the ionic bond between Na and Cl will be formed. (3) Electronegativity difference of A and B From the line of argument used in (2), we can say that atoms A and B if they have greatly different electronegativities, only then they will form an ionic bond. In fact, a difference of 2 or more is necessary for the formation of an ionic bond between atoms A and B. Thus Na has electronegativity 0.9, while Cl has 3.0. Since the difference is (3.0 – 0.9) = 2.1, Na and Cl will form an ionic bond. FACTORS GOVERNING THE FORMATION OF IONIC BOND (1) Ionisation Energy The ionisation energy of the metal atom which looses electron(s) should be low so that the formation of +vely charged ion is easier. Lower the ionisation energy greater will be the tendency of the metal atom of change into cation and hence greater will be the ease of formation of ionic bond. That is why alkali metals and alkaline earth metals form ionic bonds easily. Out of these two, alkali metals form ionic bonds easily as compared to alkaline earth metals. In a group the ionisation energy decreases as we move down the group and therefore, the tendency to form ionic bond increases in a group downward. Due to this reason Cs is the most electropositive atom among the alkali metals. (2) Electron Affinity The atom which accepts the electron and changes into anion should have high electron affinity. Higher the electron affinity more is the energy released and stable will be the anion formed. The elements of group VI A and VII A have, in general, higher electron affinity and have high tendency to form ionic bonds. Out of these two, the elements of group VII A (halogens) are more prone to the formation of ionic bond than the elements of group VI A. In moving down a group the electron affinity decreases and, therefore, the tendency to form ionic bond also decreases. (3) Lattice Energy After the formation of cations and anions separately, they combine to form ionic compound. In this process, energy is released. It is called Lattice Energy. It may be defined as ―the amount of energy released when one mole of an ionic compound is formed from its cations and anions.‖ Greater the lattice energy, greater the strength of ionic bond. The value of lattice energy depends upon the following two factors : (a) Size of the ions In order to have the greater force of attraction between the cations and anions their size should be small as the force of attraction is inversely proportional to the square of the distance between them. (b) Charge on Ions Greater the charge on ions greater will be the force of attraction between them and, therefore, greater will be the strength of the ionic bond. Necessary for the formation of an ionic bond between atoms A and B. Thus Na has electronegativity 0.9, while Cl has 3.0. Since the difference is (3.0 – 0.9) = 2.1, Na and Cl will form an ionic bond. SOME EXAMPLES OF IONIC COMPOUNDS i) NaCl: Sodium (Na) gives one (01) electron to Chlorine (Cl) to form NaCl. ii) Al2O3: Two moles Aluminum give six (06) electrons to three moles of Oxygen (two electrons for each oxygen) to form two moles of Al2O3. Characteristics of Ionic bond/ ionic compounds: i) Solids at room temperature: Because of strong electrostatic forces of the opposite ions form crystal lattice solids. ii) High Melting and Boiling Points: To breakdown the strong attractive forces among the atoms in ionic bond need high temperature to melt as well as to boil. iii) Hard and Brittle: Ionic compounds are hard because of the strong electrostatic forces hold each ion in its allocated positions. The positions are as follows: When force is applied ( + ) and ( – ) ions in the two layers thus repel each other and fall apart. The crystal cleaves here. That‘s why ionic compounds are brittle in nature. iv) Soluble in Water: When a crystal of an ionic substance is placed in the water, the polar water molecules detach the ( + ) and ( – ) ions from the crystal lattice by their electrostatic pull. These ions then get surrounded by water molecules and can lead an independent existence and are thus dissolved in water. v) Conductors of Electricity: Solid ionic compounds are poor conductors of electricity because the ions are fixed rigidly in their positions. In the molten state in water solutions, ions are rendered free to move about. Thus molten ionic compounds or their aqueous solutions conduct a current when placed in an electric cell. vi) Do not exhibit isomerism. Ionic compounds have no isomers, and don‘t show any isomerism. vii)Ionic reactions are fast. The reactions that take placed by the ionic compounds are faster than the covalent compounds. Lecture – 12 Covalent Bond 2) Covalent Bond: The electron transfer theory could not explain the bonding in molecules such as H2, O2, Cl2 etc., and in organic molecules, that had no ions. It was G.N. Lewis who suggested that two atoms could achieve stable 2 or 8 electrons in the outer shell by sharing electrons between them. Let us consider a general case where an atom A has one valence electron and another atom B has seven valence electrons. As they approach each other, each atom contributes one electron and the resulting electron pair fills the outer shell of both the atoms. Thus A acquires stable 2 electrons and B, 8 electrons in the outer shell. The attraction force between atoms (non-metals) created by the sharing of an electron pair (one from each atom) is called covalent bond. The compounds containing a covalent bond are called covalent compounds. CONDITIONS FOR FORMATION OF COVALENT BOND The conditions favourable for the formation of covalent bonds are : (1) Number of valence electrons Each of the atoms A and B should have 5, 6 or 7 valence electrons so that both achieve the stable octet by sharing 3, 2 or 1 electron-pair. H has one electron in the valence shell and attains duplet. The non-metals of groups VA, VIA and VIIA respectively satisfy this condition. (2) Equal electronegativity The atom A will not transfer electrons to B if both have equal electronegativity, and hence electron sharing will take place. This can be strictly possible only if both the atoms are of the same element. (3) Equal sharing of electrons The atoms A and B should have equal (or nearly equal) electron affinity so that they attract the bonding electron pair equally. Thus equal sharing of electrons will form a nonpolar covalent bond. Of course, precisely equal sharing of electrons will not ordinarily occur except when atoms A and B are atoms of the same element, for no two elements have exactly the same electron affinity. Examples: i) Hydrogen gas (H2): ii) Chlorine gas (Cl2) Characteristics of Covalent Bond / Covalent Compounds: (i) Gases, Liquids or Solids at room temperature: They are often gases, liquids or relatively soft solids under ordinary conditions/ at normal room temperature. This is so because of the weak intermolecular forces between the molecules. (ii)Low melting and boiling points: Solid compounds have generally low melting points because molecules are held together in the solid crystal lattice by weak forces. On application of heat, the molecules are readily pulled out and these then acquire kinetic energy for free movements as in a liquid. For the same reasons, the liquid molecules are easily obtained in the gaseous from which explains low boiling points of covalent liquids. (iii)Neither hard nor brittle: There are weak forces holding the molecules in the solid crystal lattice. A molecule layer in the crystal easily slips relatively to other adjacent layers and there are no ―forces of repulsion‖ like those in ionic compounds. Thus the crystals are not broken and there is no sharp cleavage between layers on application of external force. (iv) Insoluble in water but soluble in organic solvents: They dissolved in non-polar organic solvents (benzene, ether). They don‘t show polarity because they formed by electrons sharing not by electron transferring. That‘s why these types of compounds don‘t produce any charged ions. But there are some exceptions, such as alcohol, amines, carboxylic acids are dissolved in water because of having H- bond. (v)Non-conductors of electricity: Since there are no ( + ) and ( – ) ions in the covalent molecules, the covalent compounds even in the molten or solution form are incapable of conducting electricity. (vi) Exhibit isomerism: Some of the covalent compounds show isomerism. Example, C2H6O (a covalent compound) has two isomers: CH3 – O – CH3 CH3CH2OH Dimethyl ether Ethanol (vii) Molecular reactions are slow: Chemical reactions of covalent compounds are slower than the ionic compounds. Home Work / Assignment: Difference between Ionic and Covalent Bond [Reference: Physical Chemistry. B S Bhall] 3) Co-ordinate Covalent Bond: When a bond is formed between two atoms by sharing an electron pair where both electrons come from the one of the two atoms (or ions) is called co-ordinate covalent bond. Coordinate covalent bond is denoted by ―Arrow‖ ( → ) sign. Donor Acceptor or, A → B Coordinate Compound The atom A donates the lone pair called donor, while B accepts the pair called acceptor. Examples, (1) Ammonium ion (NH4 +) Ammonia Hydrogen ion Ammonium Ion Here, N donates a pair of electron to H+ to form NH4 +. So, N is donor and H+ isacceptor. (2) Hydronium ion (H3O+) Water Hydrogen ion Hydronium ion Here, O donates a pair of electron to H+ to form H3O+. So, O is donor and H+ isacceptor. (3) Fluroborate ion (BF4) Boron trifluoride Fluoride ion Fluroborate ion Here, F donates a pair of electron to B to form BF4. So, F is donor and B is acceptor. (4) Ozone (O3) Oxygen gas Oxygen atom Ozone (5) Carbon monoxide (CO) Carbon Oxygen Carbon monoxide Polar Covalent Bond: When the two electros constitute a covalent bond that is equally shared by the two identical nuclei is called nonpolar covalent bond. Due to even distribution of the (+) and (–) charged, the two bonded atoms remain electrically neutral. ―A covalent bond in which electrons are shared unequally and the bonded atoms acquire a partial positive and negative charge is called polar covalent bond.‖ Covalent Bond Polar Covalent Bond Bonding pair (:) Bonding pair (:) equally shared unequally shared Dipole Moment: A molecule having partial positive and negative charged separated by a distance is commonly known as a dipole (two poles). Where atoms in a molecule share electrons unequally and one atom is more electronegative than another, resulting in that atom pulling more tightly on the shared pair electrons. This phenomenon is called dipole moment. Electronegativity: The chemical property or the tendency of an atom to attract the shared pair electrons towarditself is called electronegativity. Weak bonds Weak bonds are those forces of attraction that, in biological situations, do not take a large amount of energy to break. For example, hydrogen bonds are broken by energies in the order of 4 - 5 kcal/mol.; van der Waals interactions have energies around Kcal/mol. Hydrogen bonds and van der Waals interactions are considered weak bonds. Some weak bonds (roughly in order from weak to strong): Van der Waals Interactions; Hydrogen bonds; Weak covalent bonds Lecture – 13 Non-covalent interactions Non-covalent interactions are chemical bonds that play a vital role in biological systems by influencing the structure and stability of molecules and complexes. In chemistry, a non-covalent interaction differs from a covalent bond in that it does not involve the sharing of electrons, but rather involves more dispersed variations of electromagnetic interactions between molecules or within a molecule. Noncovalent interactions are individually weak but collectively strong. What are the major non-covalent interactions in biological systems? 1. electrostatic (Coulombic) interactions – interactions between charged groups, partial charges or dipoles 2. hydrogen bonds – X-H...Y interaction, where X and Y are eletronegative atoms, they are not special from the physical point of view because they can be viewed as a combination of electrostatic and London dispersion forces 3. alternative hydrogen bonds and other interactions (pi-pi, CH/pi, cation/pi, halogen bonds...) 4. van der Waals interactions – other than H-bonds and electrostatic Interactions, much weaker interactions between charged or uncharged atoms originating from London dispersion forces 5. hydrophobic interactions – are not real (physical) interactions measurable in vacuum at 0 K, but rather an interplay between solvation and desolvation in water environment, entropy driven There are three principle kinds of noncovalent forces: ionic interactions hydrophobic interactions hydrogen bonds Where are non-covalent interactions important in biological systems? Protein structure formation, protein-protein, protein-nucleic acid, protein-ligand interactions, cytoskeleton, membranes, formation of DNA double-helix, RNA structure formation, antibody-antigen, receptor-hormone, drug-target interactions, enzyme-substrate interactions. This bond is important in: holds the two strands of the DNA double helix together (hydrogen bonds) folds polypeptides into such secondary structures as the alpha helix and the beta conformation enables enzymes to bind to their substrate enables antibodies to bind to their antigen enables transcription factors to bind to each other enables transcription factors to bind to DNA enables proteins (e.g. some hormones) to bind to their receptor permits the assembly of such macromolecular machinery as o ribosomes o actin filaments o microtubules and many more Hydrogen Bond: When hydrogen (H) is covalently bonded to a highly electronegative atom X (O, N, F), the shared electron pair is pulled so close to X that a strong dipole results. Since the shared pair is removed farthest from H atom, its nucleus (the proton) is practically exposed. The H atom at the positive end of a polar bond nearly stripped of its surrounding electrons, exerts a strong electrostatic attraction on the lone pair of electrons around X in a nearby molecule. Thus : Hydrogen bond H — X ----- H — X Electrostatic attraction δ+ δ– δ+ δ– δ– δ+ H — X:+ H — X: H— X H— X Or, H — X ------ H — X The electrostatic attraction between an H atom covalently bonded to a high electronegative atom X and a lone pair of electrons on X in another molecule is called Hydrogen bonding. Hydrogen bond is represented by ―dash‖ or, ―dotted line‖ ( ------). Points to be Remember: (1) Only O, N and F which have very high electronegative and small atomic size, are capable of forming hydrogen bonds. (2) H – bond is longer and much weaker than a normal covalent bond. Hydrogen bond energy is less than 10 kcal/mole, while that of covalent bond is about 120 kcal/mole. (3) Hydrogen bonding results in long chains or clusters of a large number of ‗associated‘ molecules like many tiny magnets. (4) Like a covalent bond, hydrogen bond has a preferred bonding direction. This is attributed to the fact that hydrogen bonding occurs through p orbitals which contain the lone pair of electrons on X atom. This implies that the atoms X—H----X will be in a straight line. CONDITIONS FOR HYDROGEN BONDING The necessary conditions for the formation of hydrogen bonding are (1) High electronegativity of atom bonded to hydrogen The molecule must contain an atom of high electronegativity such as F, O or N bonded to hydrogen atom by a covalent bond. The examples are HF, H2O and NH3. (2) Small size of Electronegative atom The electronegative atom attached to H-atom by a covalent bond should be quite small. Smaller the size of the atom, greater will be the attraction for the bonded electron pair. In other words, the polarity of the bond between H atom and electronegative atom should be high. This results in the formation of stronger hydrogen bonding. For example, N and Cl both have 3.0 electronegativity. But hydrogen bonding is effective in NH3 in comparison to that in HCl. It is due to smaller size of N atom than Cl atom. Example: (1) HF: (2) H2O: (3) NH3: (4) 2 – Nitrophenol: Types of H – Bond: (1) Intermolecular Hydrogen Bond: Hydrogen bond between an H atom of one molecule with O, N or F of another molecule is called intermolecular H – bond. [Note: Between two molecules, same or different] Example: HF, H2O, NH3 etc. H — F ----- H — F ----- H — F (2) Intramolecular Hydrogen Bond: Hydrogen bond between an H atom of a molecule with O, N or F of the same molecule is called intramolecular H – bond. [Note: Within a molecule] Example: 2 – Nitrophenol, 2 – Nitrobenzoic acid etc. 2 – Nitrophenol 2 – Nitrobenzoic acid Characteristics of Hydrogen Bond: (1) Abnormally high melting and boiling point: This is because here relatively more energy is required to separate the molecules as they enter the gaseous state or the liquid state. Thus the hybrid of fluorine (HF), oxygen (H2O) and nitrogen (NH3) have abnormally high boiling and melting points compared to other hybrids of the same group which form no H – bonds. (2) High solubility of some covalent compounds: Due to formation H – bond between O, N and F atom of some covalent compounds with H – atom of water, they are soluble in water. Example: Methanol, NH3 etc. (3) Formation of three dimensional crystal lattice: Example, H2O (as like as diamond structure – tetrahedral structure) In water, O – atom can form two H – bond and acts as like as C – atom (four hands) because of having another two covalent bonds. Significance of H – Bond in biology: Hydrogen bonds occur in inorganic molecules, such as water, and organic molecules, such as DNA and proteins. The two complementary strands of DNA are held together by hydrogen bonds between complementary nucleotides (A&T, C&G). Hydrogen bonding in water contributes to its unique properties, including its high boiling point (100 °C) and surface tension. In biology, intramolecular hydrogen bonding is partly responsible for the secondary, tertiary, and quaternary structures of proteins and nucleic acids. The hydrogen bonds help the proteins and nucleic acids form and maintain specific shapes. Hydrogen bonding plays a vital role in physiological systems. Proteins contain chains of amino acids. The amino acid units are arranged in a spiral form somewhat like a stretched coil spring (forming a helix). The N-H group of each amino acid unit and the fourth C=O group following it along the chain, establishes the N–H---O hydrogen bonds. These bonds are partly responsible for the stability of the spiral structure. Hydrophobic Interactions Hydrophobic interactions describe the relations between water and hydrophobes (low watersoluble molecules). Hydrophobes are nonpolar molecules and usually have a long chain of carbons that do not interact with water molecules. The mixing of fat and water is a good example of this particular interaction. The tendency of non-polar molecule in a polar solvent (usually water) to interact with one another is called hydrophobic effect. Biological Importance of Hydrophobic Interactions Hydrophobic Interactions are important for the folding of proteins. This is important in keeping a protein stable and biologically active, because it allow to the protein to decrease in surface are and reduce the undesirable interactions with water. Besides from proteins, there are many other biological substances that rely on hydrophobic interactions for its survival and functions, like the phospholipid bilayer membranes in every cell of our body. Intermolecular forces Intermolecular forces (IMF) are the forces which mediate interaction between molecules, including forces of attraction or repulsion which act between molecules and other types of neighboring particles, e.g., atoms or ions. Intermolecular forces are weak relative to Intramolecular forces – the forces which hold a molecule together. For example, the covalent bond, involving sharing electron pairs between atoms, is much stronger than the forces present between neighboring molecules. Attractive intermolecular forces are categorized into the following types: Hydrogen bonding, Ionic bonding, dipole–dipole interactions, van der Waals forces etc. Intramolecular forces The forces between the atoms within a single molecule are called Intramolecular forces. Intermolecular forces are weaker than Intramolecular forces. Comparison between Intermolecular and Intramolecular Forces: Intermolecular forces act between molecules. In contrast, Intramolecular forces act within molecules. Intermolecular forces are weaker than Intramolecular forces. Examples of intermolecular forces include the dipole-dipole interaction, van der Waals forces etc. On the other hand, Covalent bond and H – bonding in a molecule are the example of Intramolecular forces. Lecture – 14 CONCEPT OF HYBRIDIZATION While formation of simple molecules could be explained adequately by overlap of atomic orbitals, the formation of molecules of Be, B and C present problems of greater magnitude having no solution with the previous theory. To explain fully the tendency of these atoms to form bonds and the shape or geometry of their molecules, a new concept called Hybridization is introduced. According to this concept, we may mix any number of atomic orbitals of an atom, which differ in energy only slightly, to form new orbitals called Hybrid orbitals. Thus hybridization may precisely be defined as the phenomenon of mixing up (or merging) of orbitals of an atom of nearly equal energy, giving rise to entirely new orbitals equal in number to the mixing orbitals and having same energy contents and identical shapes. Rules of Hybridization (1) Orbitals on a single atom only would undergo hybridization. (2) There should be very little difference of energy level between the orbitals mixing to form hybrid orbitals. (3) Number of hybrid orbitals generated is equal to the number of hybridizing orbitals. (4) The hybrid orbitals assume the direction of the dominating orbitals, for example, S–P hybrid, direction is determined by p – Orbitals because s – Orbitals has no directioncharacter. (5) It is the orbitals that undergo hybridization and not the electrons. For example, four orbitals of an O – atom (2s2 2px2 2py1 2pz1) belonging to second level (ie. 2s, 2px, 2py, 2pz) can hybridize to give four hybrid orbitals, two of which have two electrons each and the other two have one electron each. (6) The electron waves in hybrid orbitals repel each other and thus tend to be farthest apart. Types of Hybridization: a) sp Hybridization b) sp2 Hybridization c) sp3 Hybridization a) sp Hybridization Mixing of an s and a p – orbital. 50 % s – character and 50 % p – character. Electrons of new generated hybrid orbitals repel and are farther apart. Angle is 180° between the axes of the two orbitals. Linear hybrid One S One P Two SP 180° SP SP Example: Ethyne (CH ≡ CH) In ethyne sp hybridization is present. Here one 2s and only one 2p orbital hybridize to form two equivalent collinear orbitals; the other two 2p orbitals remain undistributed, perpendicular to the axis. One of the two sp hybrid orbitals on each of the two carbons in ethyne molecule form one σ bond by linear overlap and 2Py and 2Pz overlap with each other form two π bonds by side wise overlap. Two pure p – orbitals Promotion sp Energy 2p of Electron 2p Hybridization 2s sp hybrid orbitals 2s Ground State Excited State Hybrid State Pz Py π π H C C H H C C H π π or H C C H b) sp2 Hybridization Mixing of one s and two p – orbital. 33 % s – character and 67 % p – character. Three orbitals lie in a plane as well as new orbitals lie farthest apart. Angle formed 120°. Trigonal hybrids. One s Two p Three sp2 sp2 sp2 120° sp2 Example: Ethene (CH2 = CH2) Here one 2s and two 2p (2Px, 2Py) orbitals of the excited carbon atom are hybridized, the new orbitals lie in the xy plane while fourth pure 2Pz orbitals lie at right angles to the hybridized orbitals. In the formation of ethene two carbon atoms form one σ bond by head on overlap of two sp2 orbitals and one π bond by side wise overlap of 2Pz orbitals of two carbon atoms. Other two sp2 orbitals of each carbon form σ bonds with H – atoms. one pure p – orbital Promotion sp2 Energy 2p of Electron 2p Hybridization 2s sp2 hybrid orbitals 2s Ground State Excited State Hybridized State Pz Pz H H π bond H π H σ bond C C C C H π H sp2 – sp2 H H or H H π bond C C H H c) sp3 Hybridization Mixing of one s and three p – orbital. 25 % s – character and 75 % p – character. The mixing orbitals lie in a plane as well as new hybrid orbitals. Angle formed is 109.5°. Also called tetrahedral hybridization. One s Three p four sp3 H 109.5 ° sp3 sp3 C sp3 H H sp3 H Example: Methane (CH4) It is assumed that the orbitals of carbon atom first undergo hybridization before forming bonds. In the excited state, all the four valence shell orbitals may mix up to give identical sp3 hybrid orbitals also four in number. Each of these four sp3 orbitals possess one electron and overlaps with 1s orbitals of four H – atoms thus forming four equivalent (σ bonds) in the methane molecules. It is tetrahedral in structure and bond angles of H – C – H are identical and it is 109.5°. No Promotion sp3 p – orbital Energy 2p of Electron Hybridization 2s sp3 hybrid orbitals 2s Ground State Excited State Hybridized State H H sp3 109.5 ° sp3 H C C H sp3 H H sp3 H H