Chapter 9 - Muscles and Muscle Tissue PDF
Document Details
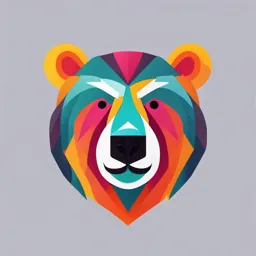
Uploaded by SensitiveSanJose
Great Bay Community College
Tags
Summary
This chapter explores muscles and muscle tissue, starting with an overview of muscle types, and their functions. It examines skeletal muscle, smooth muscle, and cardiac muscle in detail, comparing their structure and function. Finally, it discusses muscle tissue's role in maintaining posture, stabilizing joints, and generating heat.
Full Transcript
9 Muscles and Muscle Tissue In this chapter, you will learn that Muscles use actin and myosin molecules to convert the energy of ATP into force beginning with...
9 Muscles and Muscle Tissue In this chapter, you will learn that Muscles use actin and myosin molecules to convert the energy of ATP into force beginning with 9.1 Overview of muscle types, special characteristics, next exploring and functions then exploring Skeletal muscle Smooth muscle and investigating then asking and asking 9.2 Gross anatomy 9.4 How does a nerve 9.9 How does smooth muscle impulse cause a muscle fiber differ from skeletal muscle? and to contract? and finally, exploring 9.3 Microscopic and anatomy and sliding filament model 9.5 What are the properties of Developmental Aspects whole muscle contraction? of Muscles and 9.6 How do muscles generate ATP? and 9.7 What determines the force, velocity, and duration CAREER CONNECTION of contraction? and 9.8 How does skeletal muscle respond to exercise? Play a video to learn how the chapter content is used in a real healthcare setting @ Mastering A&P > Study Area. 279 280 UNIT 2 Covering, Support, and Movement of the Body Because muscles that are flexing look like mice scur- Cardiac Muscle rying beneath the skin, some scientist long ago dubbed them Cardiac muscle tissue occurs only in the heart, where it con- muscles, from the Latin mus meaning “little mouse.” Muscle is stitutes the bulk of the heart walls. Like skeletal muscle cells, the flesh on your bones, but muscle is also the dominant tissue cardiac muscle cells are striated, but cardiac muscle is not vol- in the heart and in the walls of other hollow organs. In all its untary. Indeed, it can and does contract without being stimu- forms, muscle tissue makes up nearly half the body’s mass. lated by the nervous system. Most of us have no conscious Muscles are distinguished by their ability to transform chem- control over how fast our heart beats. ical energy (ATP) into directed mechanical energy. In so doing, Key words to remember for cardiac muscle are cardiac, stri- they become capable of exerting force. ated, and involuntary. Cardiac muscle usually contracts at a fairly steady rate set There are three types 9.1 by the heart’s pacemaker, but neural controls allow the heart to speed up for brief periods, as when you race across the tennis of muscle tissue court to make that overhead smash. Learning Outcomes Smooth Muscle N Compare and contrast the three basic types of muscle tissue. Smooth muscle tissue is found in the walls of hollow visceral N List four important functions of muscle tissue. organs, such as the stomach, urinary bladder, and respiratory passages. Its role is to force fluids and other substances through 11 9 Types of Muscle Tissue internal body channels. Smooth muscle also forms valves to Chapter 4 introduced the three types of muscle tissue—skeletal, regulate the passage of substances through internal body open- cardiac, and smooth ( pp. 138–140). In this chapter, we first ings, dilates and constricts the pupils of your eyes, and forms examine the structure and function of skeletal muscle. Then we the arrector pili muscles attached to hair follicles. consider smooth muscle more briefly, largely by comparing it Like skeletal muscle, smooth muscle consists of elongated with skeletal muscle. We describe cardiac muscle in detail in cells, but smooth muscle has no striations. Like cardiac muscle, Chapter 18, but for easy comparison, Table 9.3 on pp. 312–313 smooth muscle is not subject to voluntary control. Its contrac- summarizes the characteristics of all three muscle types. tions are slow and sustained. Let’s introduce some terminology before we describe each We can describe smooth muscle tissue as visceral, nonstri- type of muscle. ated, and involuntary. Skeletal and smooth muscle cells (but not cardiac muscle cells) are elongated, and are called muscle fibers. Characteristics of Muscle Tissue Whenever you see the prefixes myo or mys (both are word What enables muscle tissue to perform its duties? Four special roots meaning “muscle”) or sarco (flesh), the reference is to characteristics are key. muscle. For example, the plasma membrane of muscle cells Excitability, also termed responsiveness, is the ability of a is called the sarcolemma (sar″ko-lem′ah), literally, “muscle” cell to receive and respond to a stimulus by changing its mem- (sarco) “husk” (lemma), and muscle cell cytoplasm is called brane potential. In the case of muscle, the stimulus is usually sarcoplasm. a chemical—for example, a neurotransmitter released by a Okay, let’s get to it. nerve cell. Contractility is the ability to shorten forcibly when Skeletal Muscle adequately stimulated. This ability sets muscle apart from Skeletal muscle tissue is packaged into the skeletal muscles, all other tissue types. organs that attach to and cover the skeleton. Skeletal muscle Extensibility is the ability to extend or stretch. Muscle cells fibers are the longest muscle cells and have obvious stripes shorten when contracting, but they can be stretched, even called striations. Although it is often activated by reflexes, beyond their resting length, when relaxed. skeletal muscle is called voluntary muscle because it is the only type subject to conscious control. Elasticity is the ability of a muscle cell to recoil and resume its resting length after stretching. When you think of skeletal muscle tissue, the key words to keep in mind are skeletal, striated, and voluntary. Muscle Functions Skeletal muscle is responsible for overall body mobility. It can contract rapidly and exert tremendous power, but it tires Muscles perform at least four important functions for the easily and must rest after short periods of activity. Skeletal mus- body: cle is also remarkably adaptable. For example, your forearm Produce movement. Skeletal muscles are responsible for all muscles can exert a force of a fraction of an ounce to pick up a locomotion and manipulation. They enable you to respond paper clip—or a force of about 7 pounds to pick up a printed quickly to jump out of the way of a car, direct your eyes, and copy of this book! smile or frown. Chapter 9 Muscles and Muscle Tissue 281 Blood courses through your body because of the rhythmically beating cardiac muscle of your heart and the smooth muscle in the walls of your blood vessels, which helps maintain blood pressure. Smooth muscle in organs of the digestive, urinary, and reproductive tracts propels substances (foodstuffs, urine, semen) through the organs and along the tract. Maintain posture and body position. We are rarely aware of the skeletal muscles that maintain body posture. Yet these muscles function almost continuously, making one tiny adjustment after another to counteract the never-ending downward pull of gravity. Stabilize joints. Even as they pull on bones to cause movement, they strengthen and stabilize the joints of the skeleton. (a) Generate heat. Muscles generate heat as they contract, which plays a critical role in maintaining normal body temperature. Check Your Understanding 1. When describing muscle, what does “striated” mean? 2. MAKE CONNECTIONS At right are photomicrographs of three types of muscle tissue (introduced in Chapter 4). For each, identify the type of muscle, state whether it 11 9 is striated, whether it is voluntary, and its major location. Create a short table that summarizes this information. For answers, see Answers Appendix. (b) A skeletal muscle is made up of muscle fibers, 9.2 nerves, blood vessels, and connective tissues Learning Outcome N Describe the gross structure of a skeletal muscle. Each skeletal muscle is a discrete organ, made up of several kinds of tissues. Skeletal muscle fibers predominate, but blood vessels, axons (nerve fibers), and substantial amounts of connective tissue are also present. We can easily examine a skeletal muscle’s shape and its attachments in the body without a microscope. (c) Nerve and Blood Supply In general, one nerve, one artery, and one or more veins serve each muscle. These struc- tures all enter or exit near the central part of the muscle and branch extensively through its connective tissue sheaths (described below). Unlike cells of cardiac and smooth mus- cle tissues, which can contract without nerve stimulation, every skeletal muscle fiber is supplied with a nerve ending that controls its activity. Skeletal muscle has a rich blood supply. This is understandable because contract- ing muscle fibers use huge amounts of energy and require almost continuous delivery of oxygen and nutrients via the arteries. Muscle cells also give off large amounts of metabolic wastes that must be removed through veins if contraction is to remain effi- cient. Capillaries, the smallest of the body’s blood vessels, take a long and winding path through muscle, and have numerous cross-links, features that accommodate changes in muscle length. They straighten when the muscle stretches and contort when the muscle contracts. Connective Tissue Sheaths In an intact muscle, there are several different connective tissue sheaths. Together these sheaths support each cell and reinforce and hold together the muscle, preventing the bulging muscles from bursting during exceptionally strong contractions. 282 UNIT 2 Covering, Support, and Movement of the Body Epimysium Bone Epimysium Perimysium Tendon Endomysium Muscle fiber in middle of a fascicle (b) Blood vessels Perimysium wrapping a fascicle Endomysium (between individual muscle fibers) 11 9 Muscle fiber Fascicle (a) Figure 9.1 Connective tissue sheaths of skeletal muscle: epimysium, perimysium, and endomysium. (b) Photomicrograph of a cross section of part of a skeletal muscle ( 30×). Let’s consider these connective tissue sheaths from exter- elasticity of muscle tissue, and also provide routes for the entry nal to internal (see Figure 9.1 and the top three rows of and exit of the blood vessels and axons that serve the muscle. Table 9.1). Epimysium. The epimysium (ep″ĭ-mis′e-um; “outside the Attachments muscle”) is an “overcoat” of dense irregular connective tissue Most skeletal muscles span at least one movable joint and that surrounds the whole muscle. Sometimes it blends with attach to bones (or other structures) in at least two places. Mus- the deep fascia that lies between neighboring muscles or the cle attachments may be direct or indirect. subcutaneous tissue deep to the skin ( p. 151). In direct, or fleshy, attachments, the epimysium of the mus- Perimysium and fascicles. Within each skeletal muscle, the cle is fused to the periosteum of a bone or perichondrium of muscle fibers are grouped into fascicles (fas′ĭ-klz; “bun- a cartilage. dles”) that resemble bundles of sticks. Surrounding each In indirect attachments, the muscle’s connective tissue wrap- fascicle is a layer of dense irregular connective tissue called pings extend beyond the muscle either as a ropelike tendon perimysium (per″ĭ-mis′e-um; “around the muscle”). (Figure 9.1a) or as a sheetlike aponeurosis (ap″o-nu-ro′sis) Endomysium. The endomysium (en″do-mis′e-um; “within (see Figure 10.12a on p. 347). The tendon or aponeurosis the muscle”) is a wispy sheath of connective tissue that sur- anchors the muscle to the connective tissue covering of a rounds each individual muscle fiber. It consists of fine areo- skeletal element (bone or cartilage) or to the fascia of other lar connective tissue. muscles. As shown in Figure 9.1, all of these connective tissue sheaths Indirect attachments are much more common because of are continuous with one another as well as with the tendons that their durability and small size. Tendons are mostly tough col- join muscles to bones. When muscle fibers contract, they pull lagen fibers, which can withstand the abrasion of rough bony on these sheaths, which transmit the pulling force to the bone projections that would tear apart the more delicate muscle tis- to be moved. The sheaths contribute somewhat to the natural sues. Because of their relatively small size, more tendons than Chapter 9 Muscles and Muscle Tissue 283 Table 9.1 Structure and Organizational Levels of Skeletal Muscle CONNECTIVE TISSUE STRUCTURE AND ORGANIZATIONAL LEVEL DESCRIPTION WRAPPINGS Muscle (organ) A muscle consists of hundreds to thousands of Covered externally by muscle cells, plus connective tissue wrappings, the epimysium Epimysium Muscle blood vessels, and axons (nerve fibers). Tendon Fascicle Fascicle (a portion of the muscle) A fascicle is a discrete bundle of muscle cells, Surrounded by segregated from the rest of the muscle by a perimysium Part of fascicle Perimysium connective tissue sheath. Muscle fiber 11 9 Muscle fiber (cell) A muscle fiber is an elongated multinucleate Surrounded by cell; it has a banded (striated) appearance. endomysium Nucleus Endomysium Sarcolemma Part of muscle fiber Myofibril Myofibril (complex organelle composed of bundles of Myofibrils are rodlike contractile elements — myofilaments) that occupy most of the muscle cell volume. Composed of sarcomeres arranged end to end, they appear banded, and bands of adjacent myofibrils are aligned. Sarcomere Sarcomere (a segment of a myofibril) A sarcomere is the contractile unit, composed — of myofilaments made up of contractile Sarcomere proteins. Thin (actin) filament Thick (myosin) filament Myofilament, or filament (extended macromolecular structure) Contractile myofilaments are of two types— — thick and thin. Thick filaments contain Thick filament Head of myosin molecule bundled myosin molecules; thin filaments contain actin molecules (plus other proteins). The sliding of the thin filaments past the thick filaments produces muscle shortening. Elastic filaments (not shown here) provide elastic recoil when tension is released and help maintain myofilament organization. Thin filament Actin molecules 284 UNIT 2 Covering, Support, and Movement of the Body fleshy muscles can pass over a joint—so tendons also conserve fiber, the dark A bands and light I bands are nearly perfectly space. aligned, giving the cell its striated appearance. (To remember this, think of “A” as in “dark” and “I” as in “light.”) Check Your Understanding As illustrated in Figure 9.2c: 3. How does the term “epimysium” relate to the role and Each A band (dark) has a lighter region in its midsection position of this connective tissue sheath? called the H zone (H for helle; “bright”). 4. MAKE CONNECTIONS What is the difference between a tendon and a ligament? (Hint: See Chapter 4, p. 133.) Each H zone is bisected vertically by a dark line called the M line (M for middle) formed by molecules of the protein For answers, see Answers Appendix. myomesin. Each I band (light) also has a midline interruption, a darker area called the Z disc (or Z line). Skeletal muscle fibers contain 9.3 Sarcomeres calcium-regulated molecular motors The region of a myofibril between two successive Z discs is Learning Outcomes a sarcomere (sar′ko-mĕr; “muscle segment”). Averaging N Describe the microscopic structure and functional roles of 2 µm long, a sarcomere is the smallest contractile unit of a mus- the myofibrils, sarcoplasmic reticulum, and T tubules of cle fiber—the functional unit of skeletal muscle. It contains an A skeletal muscle fibers. band flanked by half an I band at each end. Within each myofi- 11 9 N Describe the sliding filament model of muscle contraction. bril, the sarcomeres align end to end like boxcars in a train. Each skeletal muscle fiber is a long cylindrical cell with mul- Myofilaments tiple oval nuclei just beneath its sarcolemma (plasma mem- If we examine the banding pattern of a myofibril at the molecu- brane) (Figure 9.2b). Skeletal muscle fibers are huge cells. lar level, we see that it arises from orderly arrangement of even Their diameter typically ranges from 10 to 100 µm —up to ten smaller structures within the sarcomeres. These smaller struc- times that of an average body cell—and their length is phe- tures, the myofilaments or filaments, are the muscle equiva- nomenal, some up to 30 cm long. Their large size and multi- lents of the actin-containing microfilaments and myosin motor ple nuclei are not surprising once you learn that hundreds of proteins described in Chapter 3 ( p. 88). As you will recall, embryonic cells fuse to produce each fiber. the proteins actin and myosin play a role in motility and shape Sarcoplasm, the cytoplasm of a muscle cell, is similar to change in virtually every cell in the body. This property reaches the cytoplasm of other cells, but it contains unusually large its highest development in the contractile muscle fibers. There amounts of glycosomes (granules of stored glycogen that pro- are two types of contractile myofilaments in a sarcomere: vide glucose during muscle cell activity for ATP production) and myoglobin, a red-colored protein that stores oxygen and The central thick filaments containing myosin (red in releases it when needed. (Myoglobin is similar to hemoglobin, Figure 9.2c–e) extend the entire length of the A band. They the protein that transports oxygen in blood.) are connected in the middle of the sarcomere at the M line. In addition to the usual organelles, a muscle cell contains The more lateral thin filaments containing actin (blue in three specialized structures: myofibrils, sarcoplasmic reticu- Figure 9.2c–e) extend across the I band and partway into lum, and T tubules. Let’s look at these structures more closely the A band. The Z disc, a protein sheet, anchors the thin fila- because they play important roles in muscle contraction. ments. We describe the third type of myofilament, the elastic filament, in the next section. Myofibrils A close look at myofibril arrangement and banding patterns A single muscle fiber contains hundreds to thousands of rod- reveals that: like myofibrils that run parallel to its length (Figure 9.2b). The A hexagonal arrangement of six thin filaments surrounds each myofibrils, each 1–2 µm in diameter, are so densely packed in thick filament, and three thick filaments enclose each thin fila- the fiber that mitochondria and other organelles appear to be ment. This is shown in Figure 9.2e (far right), which shows a squeezed between them. They account for about 80% of cel- cross section of a sarcomere in an area where thick and thin lular volume. filaments overlap. Myofibrils are made up of a chain of sarcomeres linked The H zone of the A band appears less dense because the end to end. Sarcomeres (shown in Figure 9.2c and described thin filaments do not extend into this region. shortly) contain even smaller rodlike structures called myofila- ments. The bottom three rows of Table 9.1 (p. 283) summarize The M line in the center of the H zone is slightly darker these structures. because of the fine protein strands there that hold adjacent thick filaments together. Striations The myofilaments are held in alignment at the Z discs and Striations, a repeating series of dark and light bands, are evi- the M lines, and are anchored to the sarcolemma at the dent along the length of each myofibril. In an intact muscle Z discs. Chapter 9 Muscles and Muscle Tissue 285 (a) Photomicrograph of portions Nuclei of two muscle fibers (7003). Notice the striations (alternating dark and light A band (dark) bands). I band (light) Fiber (b) Diagram of part of a muscle fiber showing the myofibrils. One Sarcolemma myofibril extends from the cut end of the fiber. Mitochondrion 11 9 Myofibril A band (dark) I band (light) Nucleus Thin (actin) filament Z disc H zone Z disc (c) Small part of one myofibril enlarged to show the myofilaments responsible for the banding pattern. Each sarcomere extends from one Z disc to the next. Thick (myosin) I band A band I band M line filament Sarcomere Z disc M line Z disc (d) Enlargement of one Thin (actin) sarcomere (sectioned filament lengthwise). Elastic (titin) filaments Thick (myosin) filament (e) Cross sections of a sarcomere cut through Myosin in different locations. filament Actin filament I band H zone M line Outer edge of A band thin filaments thick filaments thick filaments linked thick and thin only only by accessory proteins filaments overlap Figure 9.2 Microscopic anatomy of a skeletal muscle fiber. 286 UNIT 2 Covering, Support, and Movement of the Body Longitudinal section of filaments within one sarcomere of a myofibril Z disc Z disc 11 9 In the center of the sarcomere, the thick filaments lack myosin heads. Myosin heads are present only in areas of myosin-actin overlap. Thick filament Thin filament Each thick filament consists of many myosin molecules A thin filament consists of two strands of actin subunits whose heads protrude at opposite ends of the filament. twisted into a helix plus two types of regulatory proteins (troponin and tropomyosin). Portion of a thick filament Portion of a thin filament Myosin head Tropomyosin Troponin Actin Actin-binding sites Heads Tail ATP- binding Myosin- site binding sites Flexible hinge region Myosin molecule Actin subunits Figure 9.3 Composition of thick and thin filaments. Molecular Composition of Myofilaments together to form myosin’s rodlike tail, and each heavy chain Muscle contraction depends on the myosin- and actin-contain- ends in a globular head that is attached to the tail via a flexible ing myofilaments. As noted earlier, thick filaments are com- hinge (Figure 9.3). posed primarily of the protein myosin. Each myosin molecule The globular heads, each associated with two light chains, consists of six polypeptide chains: two heavy (high-molecular- are the “business end” of myosin. During contraction, they link weight) chains and four light chains. The heavy chains twist the thick and thin filaments together, forming cross bridges, Chapter 9 Muscles and Muscle Tissue 287 and swivel around their point of attachment, acting as motors to H OMEOSTATIC CLINICAL generate force. Myosin itself splits ATP (acts as an ATPase) and IMBALANCE 9.1 uses the released energy to drive movement as we describe later. The term muscular dystrophy refers to a group of inherited mus- Each thick filament contains about 300 myosin molecules cle-destroying diseases that generally appear during childhood. bundled together, with their tails forming the central part of the The affected muscles initially enlarge due to deposits of fat and thick filament and their heads facing outward at the end of each connective tissue, but the muscle fibers atrophy and degenerate. molecule (Figure 9.3). As a result, the central portion of a thick The most common and serious form is Duchenne muscular filament (in the H zone) is smooth, but its ends are studded with dystrophy (DMD), which is inherited as a sex-linked reces- a staggered array of myosin heads. sive disease. It is expressed almost exclusively in males (one The thin filaments are composed chiefly of the protein in every 3600 male births) and is diagnosed when the child is actin (blue in Figure 9.3). Actin filaments consist of kidney- between 2 and 7 years old (Figure 9.4). Active, normal-appear- shaped polypeptide subunits, called globular actin or G actin. ing children become clumsy and fall frequently as their skeletal Each G actin has a myosin-binding site (or active site) to which muscles weaken. The disease progresses relentlessly from the the myosin heads attach during contraction. G actin subunits extremities upward, finally affecting the head and chest mus- polymerize into long actin filaments called filamentous, or F, cles, and cardiac muscle. The weakness continues to progress, actin. Two intertwined actin filaments, resembling a twisted but with supportive care, DMD patients are living into their 30s double strand of pearls, form the backbone of each thin fila- and beyond. ment (Figure 9.3). Thin filaments also contain several regulatory proteins. 11 9 Polypeptide strands of tropomyosin (tro″po-mi′o-sin), a rod- shaped protein, spiral about the actin core and help stiffen and stabilize it. Successive tropomyosin molecules are arranged end to end along the actin filaments, and in a relaxed muscle fiber, they block myosin-binding sites on actin so that myosin heads on the thick filaments cannot bind to the thin filaments. Troponin (tro′po-nin), the other major protein in thin fila- ments, is a globular protein with three polypeptide subunits (Figure 9.3). One subunit attaches troponin to actin. Another subunit binds tropomyosin and helps position it on actin. The third subunit binds calcium ions. Both troponin and tropomyosin help control the myosin-actin interactions involved in contraction. Several other proteins help form the structure of the myofibril. The elastic filament we referred to earlier is composed of the giant protein titin (Figure 9.2d). Titin extends from the Figure 9.4 A child with Duchenne muscular dystrophy Z disc to the thick filament, and then runs within the thick (DMD). Physiotherapy can help maintain mobility. filament (forming its core) to attach to the M line. It holds the thick filaments in place, maintaining the organization of DMD is caused by a defective gene for dystrophin, the cyto- the A band, and helps the muscle cell spring back into shape plasmic protein described above. Dystrophin links the cytoskel- after stretching. (The part of the titin that spans the I bands is eton to the extracellular matrix and, like a girder, helps stabilize extensible, unfolding when the muscle stretches and recoiling the sarcolemma. The fragile sarcolemma of DMD patients tears when the tension is released.) Titin does not resist stretching during contraction, allowing entry of excess Ca 2 + , which dam- in the ordinary range of extension, but it stiffens as it uncoils, ages the contractile fibers. Inflammatory cells (macrophages helping the muscle resist excessive stretching, which might and lymphocytes) accumulate in the surrounding connective tis- pull the sarcomeres apart. sue. As the regenerative capacity of the muscle is lost, damaged Another important structural protein is dystrophin, which cells undergo apoptosis, and muscle mass drops. links the thin filaments to the integral proteins of the sar- There is still no cure for DMD. Treatment with corticos- colemma (which in turn are anchored to the extracellular teroids helps those with DMD remain mobile longer, but the matrix). effects are temporary and there are significant side effects. Newer nucleic acid–based drugs, such as eteplirsen, are treat- Other proteins that bind filaments or sarcomeres together ment options for patients with certain genetic mutations. They and maintain their alignment include nebulin, myomesin, and target the defective dystrophin pre-mRNA to restore the dystro- C proteins. Intermediate (desmin) filaments extend from the phin protein. The first gene therapy to treat DMD was approved Z disc and connect each myofibril to the next throughout the in 2023. width of the muscle cell. 288 UNIT 2 Covering, Support, and Movement of the Body Part of a skeletal I band A band I band muscle fiber (cell) Z disc H zone Z disc M line Sarcolemma Myofibril Triad: T tubule Terminal Sarcolemma cisterns of the SR (2) Tubules of the SR 11 9 Myofibrils Mitochondria Figure 9.5 Relationship of the saclike terminal cisterns next to the A-I cisterns. (See detailed view in Focus sarcoplasmic reticulum and T tubules junctions. The T tubules (gray) are Figure 9.2, pp. 294–295.) to myofibrils of skeletal muscle. The invaginations of the sarcolemma that run tubules of the SR (blue) fuse to form the deep into the cell between the terminal Sarcoplasmic Reticulum and T Tubules Figure 9.5. The lumen (cavity) of the T tubule is continuous with the extracellular space. T tubules allow changes in the mem- Skeletal muscle fibers contain two sets of intracellular tubules brane potential to rapidly penetrate deep into the muscle fiber. that help regulate muscle contraction: (1) the sarcoplasmic Along its length, each T tubule runs between the paired reticulum and (2) T tubules. terminal cisterns of the SR, forming triads (Figure 9.5), suc- Sarcoplasmic Reticulum cessive groupings of the three membranous structures (termi- nal cistern, T tubule, and terminal cistern). As they pass from Shown in blue in Figure 9.5, the sarcoplasmic reticulum one myofibril to the next, the T tubules also encircle each (SR) is an elaborate smooth endoplasmic reticulum. The SR sarcomere. regulates intracellular levels of ionic calcium. It stores calcium Muscle contraction is ultimately controlled by nerve- and releases it on demand when the muscle fiber is stimulated initiated electrical impulses that travel along the sarcolemma. to contract. As you will see, calcium provides the final “go” Because T tubules are continuations of the sarcolemma, they signal for contraction. conduct impulses to the deepest regions of the muscle cell and Interconnecting tubules of SR surround each myofibril the every sarcomere. These impulses trigger the release of calcium way the sleeve of a loosely knitted sweater surrounds your from the adjacent terminal cisterns. Think of the T tubules as arm. Most SR tubules run longitudinally along the myofibril, a rapid communication or messaging system that ensures that communicating with each other at the H zone. Others called every myofibril in the muscle fiber contracts at virtually the terminal cisterns (“end sacs”) form larger, perpendicular cross same time. channels at the A band–I band junctions, and they always occur in pairs. Closely associated with the SR are large numbers of Triad Relationships mitochondria and glycogen granules, both involved in produc- ing the energy used during contraction. The roles of the T tubules and SR in providing signals for con- traction are tightly linked. At the triads, membrane-spanning T Tubules proteins from the T tubules and SR link together across the gap At each A band–I band junction, the sarcolemma of the muscle between the two membranes. cell protrudes deep into the cell interior, forming an elongated The protruding integral proteins of the T tubule act as voltage tube called the T tubule (T for “transverse”), shown in gray in sensors. Chapter 9 Muscles and Muscle Tissue 289 The integral proteins of the SR form gated channels through 1 Fully relaxed sarcomere of a muscle fiber which the terminal cisterns release Ca 2 + (see top right of Focus Figure 9.2 on p. 295). Sliding Filament Model of Contraction We almost always think “shortening” when we hear the word contraction, but to physiologists contraction refers only to the activation of myosin’s cross bridges, which are the force-generating sites. Shortening only occurs if the Thick filament M line Thin filament cross bridges generate enough tension on the thin filaments to exceed the forces that oppose shortening, such as when you lift a bowling ball. Contraction ends when the cross bridges become inactive, the tension declines, and the mus- cle fiber relaxes. In a relaxed muscle fiber, the thin and thick filaments overlap only at the ends of the A band (Figure 9.6 1 ). The sliding filament model of contraction states that during contraction, the thin filaments slide past the thick ones so that the actin and myosin filaments overlap to a greater degree. Neither the thick Z Z 11 9 H nor the thin filaments change length during contraction. At the microscopic level, the following things occur as a muscle fiber I A I shortens: The I bands shorten. 2 Fully contracted sarcomere of a muscle fiber The distance between successive Z discs shortens. As the thin filaments slide centrally, the Z discs to which they attach are pulled toward the M line (Figure 9.6 2 ). The H zones shorten and eventually disappear. Neighboring A bands move closer together, but their length does not change. As these events occur in sarcomeres throughout the cell, the muscle cell shortens. Check Your Understanding 5. Name proteins a, b, c, and d. Which protein can act as an enzyme to hydrolyze (split) ATP? Which binds Ca 2 + ? Which must move out of the way in order for cross bridges to form? Z Z a b c I A I Figure 9.6 Sliding filament model of contraction. At full contraction, the Z discs approach the thick filaments and the thin filaments overlap each other. Photomicrographs are 33,000×. d sides of the original drawing. Label an A band, an I band, and 6. Which region or organelle—cytosol, mitochondrion, or SR— an H zone. Draw and label an M line and a Z disc. contains the highest concentration of calcium ions in a resting 8. MAKE CONNECTIONS Consider a phosphorus atom that is part muscle fiber? Which structure provides the ATP needed for of the membrane of the sarcoplasmic reticulum in the biceps muscle activity? muscle of your arm. Using the levels of structural organization 7. DRAW Draw four thick filaments in each of two columns. Add (described in Chapter 1), name in order the structure that a column of four thin filaments between the thick filaments, as corresponds to each level of organization. Begin at the atomic they would appear in a relaxed myofibril. Then add two more level (the phosphorus atom) and end at the organ system level. columns, each with four thin filaments, on the left and right For answers, see Answers Appendix. 290 UNIT 2 Covering, Support, and Movement of the Body changes in the membrane potential (as we will see shortly). Motor neurons stimulate 9.4 Receptors for acetylcholine are an example of this class. An skeletal muscle fibers to contract ACh receptor is a single protein in the plasma membrane that is both a receptor and an ion channel. Learning Outcomes Voltage-gated ion channels open or close in response to N Explain how muscle fibers are stimulated to contract changes in membrane potential. They underlie all action by describing events that occur at the neuromuscular potentials. In skeletal muscle fib- junction. ers, the initial change in membrane N Follow the events of excitation-contraction coupling that potential is created by chemically lead to cross bridge activity. gated channels. In other words, N Describe the steps of a cross bridge cycle. chemically gated ion channels cause The sliding filament model tells us that myofilaments slide past a small local depolarization (a each other as the sarcomeres contract. In this module, we will decrease in the membrane potential) see exactly how this happens. But before we begin, let’s fill that then triggers the voltage-gated Voltage-gated ion channel in some background information that will help you understand ion channels to create an action this topic, and then look at the big picture of how muscle con- potential. traction works. (We will describe ion channels in more detail in Chapter 11.) 11 9 Background and Overview Anatomy of Motor Neurons and the Remember that skeletal muscle contractions are voluntary. Neuromuscular Junction For example, you decide when you want to contract your Motor neurons that activate skeletal muscle fibers are called biceps muscle to pick up your cell phone. Making that deci- somatic motor neurons, or motor neurons of the somatic (vol- sion involves many neurons in your brain, but the contraction untary) nervous system (Figure 9.7). These neurons reside in of a skeletal muscle ultimately comes down to activating a few the spinal cord (except for those that supply the muscles of the motor neurons in the spinal cord. Motor neurons are the way head and neck). Each neuron has a long threadlike extension that the nervous system connects with skeletal muscles and called an axon that extends from the cell body in the spinal cord “tells” them to contract. to the muscle fiber it serves ( see p. 140 to review the parts of Both neurons and muscle fibers are excitable cells. That a neuron). These axons exit the spinal cord and pass throughout is, they respond to external stimuli by changing their resting the body bundled together as nerves. membrane potential. (Remember that all cells have a resting The axon of each motor neuron branches extensively as it membrane potential, which is a voltage across the plasma mem- enters the muscle so that it can innervate (supply) multiple mus- brane; pp. 79–80.) These changes in membrane potential act cle fibers. When it reaches a muscle fiber, each axon divides as signals. One type of electrical signal is called an action again, giving off several short, curling branches that collectively potential (AP; sometimes called a nerve impulse). An AP is a form an oval neuromuscular junction, or motor end plate, large change in membrane potential that spreads rapidly over with a single muscle fiber. long distances within a cell. Generally, APs don’t spread from Each muscle fiber has only one neuromuscular junction, cell to cell. For this reason, the signal has to be converted to a located approximately midway along its length. The end of chemical signal—a chemical messenger called a neurotransmit- the axon, called the axon terminal, and the muscle fiber are ter ( p. 82) that diffuses across the small gap between excit- exceedingly close (50–80 nm apart), but they remain separated able cells to start the signal again. The neurotransmitter that by a space, the synaptic cleft (Figure 9.7), which is filled with motor neurons use to “tell” skeletal muscle fibers to contract is a gel-like extracellular substance rich in glycoproteins and col- acetylcholine (as″ĕ-til-ko′lēn), or ACh. lagen fibers. Within the mound-like axon terminal are synaptic vesicles, Ion Channels small membranous sacs containing the neurotransmitter acetyl- Rapidly changing the membrane potential in neurons and choline. The trough-like part of the muscle fiber’s sarcolemma muscle fibers requires the opening and closing of membrane that helps form the neuromuscular junction is highly folded. channel proteins that allow certain ions to pass across the mem- These junctional folds provide a large surface area for the brane ( p. 70). The movement of ions through these ion chan- thousands of ACh receptors located there. nels changes the membrane voltage. To summarize, the neuromuscular junction is like a sand- Two classes of ion channels are impor- Chemical messenger (e.g., ACh) wich: It is made up of part of a neuron (the axon terminals), tant for excitation and contraction of part of a muscle fiber (the junctional folds), and the “filler” skeletal muscle: between them (the synaptic cleft). Chemically gated ion channels are opened by chemical messengers The Big Picture (e.g., neurotransmitters). This class Chemically gated ion Figure 9.7 presents an overview of skeletal muscle contrac- of ion channel creates small local channel tion and divides this process into four groups of steps. We will Chapter 9 Muscles and Muscle Tissue 291 Brain Spinal cord Axon of motor neuron Motor neuron: The neuromuscular junction is the Cell body region where the motor neuron contacts the skeletal muscle. It Axon consists of multiple axon terminals and the underlying junctional folds Axon of the sarcolemma. terminals Muscle fiber Sequence of events leading to contraction: Axon of motor 11 9 neuron Axon terminal of A motor neuron fires an action potential motor neuron (AP) down its axon. Synaptic vesicle with ACh 1 Events at the The motor neuron’s axon terminal Synaptic cleft neuromuscular releases acetylcholine (ACh) into the junction (see synaptic cleft. Focus Figure 9.1) Cytoplasm of skeletal muscle fiber ACh binds receptors on the junctional folds of the sarcolemma. Junctional folds of the sarcolemma ACh binding causes a local depolarization called an end plate potential (EPP). 2 Muscle fiber explore each group of steps in more detail in the rest of this The local depolarization (EPP) triggers an excitation (see Figure 9.8) AP in the adjacent sarcolemma. module: 1 Events at the neuromuscular junction (see Focus Figure 9.1 on p. 292). The motor neuron releases ACh that stimu- lates the skeletal muscle fiber, causing a local depolariza- AP in sarcolemma travels down T tubules. tion (decrease in membrane potential) called an end plate potential (EPP). 3 Excitation- 2 Muscle fiber excitation. The EPP triggers an action poten- contraction coupling Sarcoplasmic reticulum releases Ca2+. tial that travels across the entire sarcolemma (see Figure 9.8 (see Focus Figure 9.2) on p. 293). 3 Excitation-contraction coupling (see Focus Figure 9.2 on Ca2+ binds to troponin, which shifts tropomyosin to uncover the myosin-binding pp. 294–295). The AP in the sarcolemma propagates along sites on actin. Myosin heads bind actin. the T tubules and causes release of Ca 2 + from the terminal cisterns of the SR. Ca 2 + is the final trigger for contraction. It is the internal messenger that links the AP to contraction. Ca 2 + 4 Cross bridge cycle binds to troponin and this causes the myosin-binding sites on Contraction occurs via cross bridge cycling. (see Focus Figure 9.3) actin to be exposed so that myosin heads can bind to actin. 4 Cross bridge cycling (see Focus Figure 9.3 on p. 297). The muscle contracts as a result of a repeating cycle of steps Figure 9.7 Overview of skeletal muscle contraction. that cause myofilaments to slide relative to each other. FOCUS FIGURE 9.1 Events at the Neuromuscular Junction When an action potential reaches a Play A&P Flix Video: Events at the Neuromuscular neuromuscular junction, acetylcholine (ACh) Junction @ Mastering A&P > Study Area is released. Upon binding to sarcolemma receptors, ACh causes a change in sarcolemma Axon of permeability leading to a change in Action motor neuron membrane potential. potential (AP) Axon terminal of neuromuscular junction Sarcolemma of the muscle fiber 1 Action potential arrives at axon terminal of motor neuron. Ca2+ 2 Voltage-gated Ca2+ Ca2+ channels open. Ca2+ enters the Synaptic vesicle axon terminal, moving down its containing ACh electrochemical gradient. Axon terminal Synaptic cleft of motor neuron 3 Ca2+ entry causes ACh (a Fusing neurotransmitter) to be released synaptic by exocytosis. vesicles ACh Junctional folds of sarcolemma 4 ACh diffuses across the synaptic cleft and binds to ACh receptors on the sarcolemma. Sarcoplasm of muscle fiber 5 ACh binding opens chemically gated ion channels that allow simultaneous passage of Na+ into Na+ K+ Postsynaptic membrane the muscle fiber and K+ out of ion channel opens; the muscle fiber. More Na+ ions ions pass. enter than K+ ions exit, which produces a local change in the membrane potential called the end plate potential. 6 ACh effects are terminated by ACh Degraded ACh Ion channel closes; its breakdown in the synaptic Na+ ions cannot pass. cleft by acetylcholinesterase and diffusion away from the junction. + Acetylcholinesterase K 292 Chapter 9 Muscles and Muscle Tissue 293 ACh-containing Open voltage- Closed voltage- Axon terminal of synaptic vesicle gated Na1 channel gated K1 channel neuromuscular junction Na1 Sarcolemma 22222222 22222 2 2 2 2 2 21 1 1 1 Ca21 Ca21 Synaptic cleft 11111111 1111 1111 2 2 2 2 1 K Action potential 2 Depolarization: Generating and propagating an action potential Wave of (AP). Depolarization of the sarcolemma opens voltage-gated sodium depolarization channels. Na1 enters, following its electrochemical gradient. At a certain membrane voltage, an AP is generated (initiated). The AP spreads to adjacent areas of the sarcolemma and opens voltage-gated Na1 channels 1 An end plate potential (EPP) is generated at the there, propagating the AP. The AP propagates along the sarcolemma in all neuromuscular junction (see Focus Figure 9.1). The EPP causes directions, just like ripples from a pebble dropped in a pond. a wave of depolarization that spreads to the adjacent sarcolemma. Figure 9.8 Summary of events in the generation and 11 9 propagation of an action potential in a skeletal muscle fiber. Closed voltage- Open voltage- gated Na1 channel gated K1 channel Na1 Events at the Neuromuscular Junction 11111111 1111 1111111111 How does a motor neuron stimulate a skeletal muscle fiber? Focus on Events at the Neuromuscular Junction ( Focus 22222222 2222 2222222222 Figure 9.1) covers this process step by step. Study this figure before continuing. K+ The result of the events at the neuro- Play Interactive Physiology 2.0: muscular junction is The Neuromuscular Junction a transient change in @ Mastering A&P > Study Area 3 Repolarization: Restoring the sarcolemma to its initial polarized membrane potential state (negative inside, positive outside). The repolarization wave is also a consequence of opening and closing ion channels—voltage-gated Na1 that causes the interior of the sarcolemma to become less nega- channels close and voltage-gated K1 channels open. The potassium ion tive (a depolarization). This local depolarization is called an concentration is substantially higher inside the cell than in the extracellular end plate potential (EPP). The EPP spreads to the adjacent fluid, so K1 diffuses out of the muscle fiber. This restores the negatively charged conditions inside that are characteristic of a sarcolemma at rest. sarcolemma and triggers an AP there. After ACh binds to the ACh receptors, its effects are quickly terminated by acetylcholinesterase (as″ĕ-til-ko″lin-es′ter-ās), an enzyme located in the synaptic cleft. Acetylcholinesterase breaks down ACh to its building blocks, acetic acid and choline. Removing ACh prevents continued muscle fiber contraction in Generation of an Action Potential across the absence of additional nervous system stimulation. the Sarcolemma Now let’s consider the electrical events that trigger an action potential along the sarcolemma. An action potential is the result H OMEOSTATIC of a predictable sequence of electrical changes. Once initiated, CLINICAL an action potential sweeps along the entire surface of the sarco- IMBALANCE 9.2 Many toxins, drugs, and diseases interfere with events at the lemma. Three steps are involved in triggering and then propa- neuromuscular junction. For example, myasthenia gravis gating an action potential. These three steps—generation of an ( asthen = weakness; gravi = heavy ) , a disease character- end plate potential followed by action potential depolarization ized by drooping upper eyelids, difficulty swallowing and talking, and repolarization—are shown in Figure 9.8. A tracing of the and generalized muscle weakness, involves a shortage of ACh receptors. Myasthenia gravis is an autoimmune disease in which the immune system destroys ACh receptors. (Text continues on p. 296.) FOCUS FIGURE 9.2 Excitation-Contraction Coupling Excitation-contraction (E-C) coupling is the sequence of events by which transmission of an action potential along the sarcolemma leads to the sliding of myofilaments. Play A&P Flix Video: Excitation-Contraction Coupling @ Mastering A&P > Study Area Setting the stage The events at the neuromuscular junction (NMJ) set the stage for E-C coupling by providing excitation. Released acetylcholine binds to receptor proteins on the sarcolemma and triggers an action potential in a muscle fiber. Axon terminal of Synaptic motor neuron at NMJ cleft Action potential is generated ACh Sarcolemma T tubule Terminal cistern of SR Ca2+ Muscle fiber One sarcomere One myofibril 294 Steps in E-C Coupling: Sarcolemma Voltage-sensitive T tubule 1 The action potential (AP) propagates tubule protein along the sarcolemma and down the T tubules. Ca2+ release channel 2 Calcium ions are released. Transmission of the AP along the T tubules of the triads causes the Terminal voltage-sensitive tubule proteins to cistern change shape. This shape change opens of SR the Ca2+ release channels in the terminal cisterns of the sarcoplasmic reticulum (SR), allowing Ca2+ to flow into the cytosol. Ca2+ Actin Troponin Tropomyosin blocking myosin-binding sites Myosin 3 Calcium binds to troponin and removes the blocking action of Ca2+ tropomyosin. When Ca2+ binds, troponin changes shape, exposing myosin-binding sites on the thin filaments. Myosin-binding sites exposed and ready for myosin binding 4 Contraction begins: Myosin binding to actin forms cross bridges and contraction (cross Myosin bridge cycling) begins. At this cross point, E-C coupling is over. bridge The aftermath When the muscle AP ceases, the voltage-sensitive tubule proteins return to their original shape, closing the Ca2+ release channels of the SR. Ca2+ levels in the sarcoplasm fall as Ca2+ is continually pumped back into the SR by active transport. Without Ca2+, the blocking action of tropomyosin is restored, myosin-actin interaction is inhibited, and relaxation occurs. Each time an AP arrives at the neuromuscular junction, the sequence of E-C coupling is repeated. 295 296 UNIT 2 Covering, Support, and Movement of the Body 2 …depolari- 3 Voltage-gated Muscle Fiber Contraction: zation, which is due to Na+ entry. Na+ channels close; voltage-gated K+ Cross Bridge Cycling +30 As we have noted, cross bridge formation requires Ca 2 +. Let’s channels open, causing… see how calcium ions promote muscle cell contraction. Membrane potential (mV) When intracellular calcium levels are low, the muscle cell is 0 4 …repolarization, relaxed because tropomyosin molecules physically block the 1 Voltage-gated Na+channels which is due to K+ exit. myosin-binding sites on actin. As Ca 2 + levels rise, the ions bind open, causing… to regulatory sites on troponin. Two calcium ions must bind to a troponin, causing it to change shape and roll tropomyosin into the groove of the actin helix, away from the myosin-bind- 5 Voltage-gated K+ ing sites. In short, the tropomyosin blockade is removed when Resting channels have closed. membrane sufficient calcium is present. Once binding sites on actin are potential exposed, the events of the cross bridge cycle occur in rapid suc- –90 cession, as depicted in Focus on the Cross Bridge Cycle (Focus 0 5 10 15 20 Figure 9.3). The steps of the cycle are analogous to the steps Time (ms) you would take to pull a rope toward you if you were using only Figure 9.9 Recording of an action potential (AP) in a muscle one hand (Figure 9.10). The cycle repeats and with each cycle, fiber. An AP is a brief change in membrane potential. the myosin head attaches to a myosin-binding site on an actin 11 9 further along the thin filament. The thin filaments continue to slide as long as calcium and adequate ATP are present. The many myosin heads along the length of a thick filament resulting membrane potential changes is shown in Figure 9.9. can be at different steps of the cross bridge cycle. As a result, the We will describe action potentials in more detail in Chapter 11. thin filaments cannot slide backward during muscle fiber contrac- During repolarization, a muscle fiber is said to be in a tion because roughly half of the myosin heads are always in con- refractory period, because the cell cannot be stimulated tact with actin. (The other myosin heads are randomly seeking again until repolarization is complete. Note that repolarization their next binding site.) Contracting muscles routinely shorten restores only the electrical conditions of the resting (polarized) by 30–35% of their total resting length, so each myosin head state. The ATP-dependent Na + -K + pump restores the ionic completes many cross bridge cycles during a single contraction. conditions of the resting state, but thousands of action poten- As soon as Ca 2 + is released from the SR, the Ca 2 + pumps tials can occur before ionic imbalances interfere with contrac- of the SR begin to reclaim it from the cytosol. As Ca 2 + levels tile activity. drop, Ca 2 + comes off of troponin, which again changes shape Once initiated, the action potential is unstoppable. It ulti- and pulls tropomyosin up to block actin’s myosin-binding sites. mately results in contraction of the muscle fiber. Although the action potential itself lasts only a few milliseconds Marker (ms), the contraction phase of a muscle fiber may persist for 100 ms or more and far outlasts the electrical event that triggers it. 1 “Grab rope” = cross bridge formation Excitation-Contraction Coupling Excitation-contraction (E-C) coupling is the sequence of events by which transmission of an action potential along the sarcolemma causes myo- filaments to slide. The Play Interactive Physiology 4 “Reach forward” = 2 “Pull” = power stroke action potential is brief 2.0: Excitation-Contraction cocking myosin head and ends well before any Coupling @ Mastering A&P signs of contraction are > Study Area obvious. As you will see, the electrical signal does not act directly on the myofilaments. Instead, it causes the rise in intracellular 3 “Let go” = cross bridge detachment levels of calcium ions, which triggers a sequence of events that ultimately leads to sliding of the filaments. Figure 9.10 Steps of the cross bridge cycle parallel those Focus on Excitation-Contraction Coupling ( Focus used to pull a rope with one hand. F igure 9.2) on pp. 294–295 illustrates the steps in this pro- cess. It also reveals how the integral proteins of the T tubules Play Interactive Physiology 2.0: Cross Bridge and terminal cisterns in the triads interact to provide the Ca 2 + Cycling @ Mastering A&P > Study Area necessary for contraction to occur. FOCUS FIGURE 9.3 Cross Bridge Cycle The cross bridge cycle is the series of events Play A&P Flix Video: The Cross Bridge Cycle during which myosin heads pull thin filaments @ Mastering A&P > Study Area toward the center of the sarcomere. Actin Ca2+ Thin filament ADP Myosin cross bridge Pi Thick filament Myosin 1 Cross bridge formation. Energized myosin head attaches to an actin myofilament, forming a cross bridge. ADP ADP ATP Pi Pi hydrolysis 4 Cocking of the myosin head. Myosin 2 The power (working) stroke. The hydrolyzes ATP to ADP and Pi. This causes myosin head pivots and bends, pulling the the myosin head to return to its prestroke actin filament toward the M line. This high-energy, or "cocked," position.* leaves the myosin head in its low-energy state. ADP and Pi are also released. In the absence of ATP, myosin heads will not detach, causing rigor mortis. ATP ATP *This cycle will continue as long as ATP is 3 Cross bridge detachment. ATP binds to 2+ available and Ca is bound to troponin. If myosin and causes the myosin head to detach ATP is not available, the cycle stops from actin (the cross bridge "breaks"). between steps 2 and 3. 297 298 UNIT 2 Covering, Support, and Movement of the Body The contraction ends, and the muscle fiber relaxes. When cross Check Your Understanding bridge cycling ends, the myosin heads remain in their upright 9. From the time an action potential reaches the axon terminal of high-energy configuration (Focus Figure 9.3 4 ), ready to bind a motor neuron until cross bridge cycling begins, several sets actin when the muscle is stimulated to contract again. of ion channels are activated. List these channels in the order Except for the brief period following muscle fiber excitation, that they are activated and state what causes each to open. calcium ion concentrations in the cytosol are kept almost unde- 10. What is the final trigger for contraction? What is the initial trigger? tectably low. When action potentials arrive in quick succes- 11. What prevents the filaments from sliding back to their original sion, intracellular Ca 2 + levels soar due to successive “puffs” or posit