Joint Kinematics (PDF)
Document Details
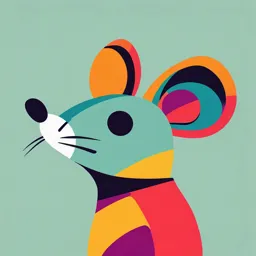
Uploaded by ReadyDystopia
Universiti Kuala Lumpur Royal College of Medicine Perak
Vengata Subramani Manoharan
Tags
Summary
These lecture notes cover joint kinematics, including various kinematic chains, arthrokinematics, and osteokinematics for upper and lower limbs, and axial skeleton. It discusses range of motion, hypermobility, and hypomobility.
Full Transcript
JOINT KINEMATICS KINESIOLOGY 1 & BIOMECHANICS RFB 10303 VENGATA SUBRAMANI MANOHARAN Learning outcome At the end of this topic you can able to: Explain the various kinematic chain. Explain the arthrokinematics and osteokinematics. Explain the kinematics of upper...
JOINT KINEMATICS KINESIOLOGY 1 & BIOMECHANICS RFB 10303 VENGATA SUBRAMANI MANOHARAN Learning outcome At the end of this topic you can able to: Explain the various kinematic chain. Explain the arthrokinematics and osteokinematics. Explain the kinematics of upper limb joints. Explain the kinematics of lower limb joints. Explain the kinematics of axial skeleton joints. Explain the linear and rotational motion. Kinematic chain Kinematic chains, in the engineering sense, are composed of a series of rigid links that are interconnected by a series of pin centered joints. The kinematic chain can be open or closed. In an open kinematic chain, one joint can move independently of others in the chain. When one end of the chain remains fixed, it creates a closed system or closed kinematic chain. Under these conditions, movement at one joint automatically creates movement in other joints in the chain. A. In a closed kinematic chain, knee flexion is accompanied by hip flexion and ankle dorsiflexion. B. Knee motion in an open kinematic chain may occur with or without motion at the hip and ankle. In the diagram, knee flexion is shown without simultaneous motion at the hip and the ankle. The ends of human limbs, especially the upper extremities, frequently are not fixed but are free to move. In these open kinematic chain motions, joint motion is much more varied. The motion of waving the hand may occur at the wrist without causing motion of the more distal finger joints distally or the more proximal elbow or shoulder. The same motion may also occur through medial and lateral rotation at the shoulder. In an open kinematic chain, motion does not occur in a predictable manner because joints may function either independently or in unison. Although the joints in the human body do not always behave in an entirely predictable manner in either a closed or an open chain, the joints are interdependent. A change in the function or structure of one joint in the system will usually cause a change in the function of a joint either immediately adjacent to the affected joint or at a distal joint. For example, if the ROM at the knee were limited, the hip and/or ankle joints would have to compensate in order that the foot could clear the floor when the person was walking, so that he or she could avoid stumbling. Arthrokinematics & Osteokinematics Range of Motion The normal ROM of a joint is sometimes called the anatomic or physiologic ROM, because it refers to the amount of motion available to a joint within the anatomic limits of the joint structure. The extent of the anatomic range is determined by a number of factors, including the shape of the joint surfaces, the joint capsule, ligaments, muscle bulk, and surrounding musculotendinous and bony structures. Range of Motion In some joints there are no bony limitations to motion, and the ROM is limited only by soft tissue structures. For example, the knee joint has no bony limitations to motion. Other joints have definite bony restrictions to motion in addition to soft tissue limitations. The humeroulnar joint at the elbow is limited in extension by bony contact of the ulna on the olecranon fossa of the humerus. The sensation experienced by the examiner performing passive physiologic movements at each joint is referred to as the end-feel. Range of Motion A ROM is considered to be pathologic when motion at a joint either exceeds or fails to reach the normal anatomic limits of motion. When a ROM exceeds the normal limits, the joint is hypermobile. When the ROM is less than what would normally be permitted by the structure, the joint is hypomobile. Hypermobility may be caused by a failure to limit motion by either the bony or soft tissues and may lead to instability. Hypomobility may be caused by bony or cartilaginous blocks to motion or by the inability of the capsule, ligaments, or muscles to elongate sufficiently to allow a normal ROM. Range of Motion A contracture, which is the shortening of soft tissue structures around a joint, is one cause of hypomobility. Either hypermobility or hypomobility of a joint may have undesirable effects, not only at the affected joint but also on adjacent joint structures. Limitation of hip extension as a consequence of osteoarthritis may lead to excessive lumbar spine movement to achieve adequate movement of the lower extremity during gait. Osteokinematics Osteokinematics refers to the movement of the bones in space during physiologic joint motion. These are the movements in the sagittal, frontal, and transverse planes that occur at joints. The movements are typically described by the plane in which they occur, the axis about which they occur, and the direction of movement. Osteokinematic movements at the humeroulnar joint include flexion or extension (direction) of the ulna on the humerus (or humerus on the ulna) in the sagittal plane about a frontal axis. Osteokinematics Note that movements are always described as if they are occurring from the anatomical position. Sometimes the direction of the movement is described by the direction of the bone in space: that is, anterior movement ulna on the humerus (flexion) or posterior movement of the humerus on the ulna (extension). Arthrokinematics Physiologic joint motion involves motion of bony segments (osteokinematics) as well as motion of the joint surfaces in relation to another. These movements accompany voluntary movement but cannot be independently produced voluntarily under normal conditions. The term arthrokinematics is used to refer to these movements of joint surfaces on one another. Usually one of the joint surfaces is relatively stable and serves as a base for the motion, whereas the other surface moves on this relatively fixed base. The terms roll, slide, and spin are used to describe the type of motion that the moving part performs. Sliding It is a pure translatory motion, refers to the gliding of one component over another, as when a braked wheel skids. The point of contact changes in the fixed component as the sliding component moves over it. In the hand, the proximal phalanx slides over the fixed end of the metacarpal during flexion and extension. Roll It refers to the rolling of one joint surface on another, as in a tire rolling on the road. In the knee, the femoral condyles roll on the fixed tibial surface during knee flexion or extension in standing. The direction of rolling is described by the direction of movement of the bone; thus, the femur rolls forward during knee extension in standing. During a pure rolling motion, a progression of points of contact between the surfaces occurs. Spin It refers to a rotation of the movable component, as when a top spins. Spin is a pure rotatory motion. The same points remain in contact on both the moving and stationary components. At the elbow, the head of the radius spins on the capitulum of the humerus during supination and pronation of the forearm. During human joint motion, combinations of rolling and sliding arthrokinematic movements usually occur in order to maintain joint integrity. The types of arthrokinematic motion that occur at a particular joint depend on the shape of the articulating surfaces. When a concave articulating surface is moving on a stable convex surface, sliding occurs in the same direction as motion of the bony lever. Because the motion of the bony lever is the direction of the roll of the bone, the roll and slide are in the same direction. This allows the joint surfaces to stay in optimum contact with each other. Convex-concave rule: When a convex joint surface moves on a concave surface, the bone must roll in one direction and glide in the opposite direction in order to maintain optimum contact. A. When a convex surface is moving on a fixed concave surface, the convex articulating surface moves in a direction opposite to the direction travelled by the shaft of the bony lever. B. When a concave surface is moving on a fixed convex surface, the concave articulating surface moves in the same direction as the remaining portion of the bony lever (proximal phalanx moving on fixed metacarpal). Most joints fit into either an ovoid or a sellar category. In an ovoid joint, one surface is convex and the other surface is concave. In a sellar joint, each joint surface is both convex and concave. Kinematics of Upperlimb joints Shoulder complex Also known as glenohumeral joint - the articulation between head of humerus and glenoid fossa of the scapula A synovial joint of the ball and socket variety The shoulder joint are link by three interdependent linkage; - SC joint - AC joint - GH joint The freedom of movement of the shoulder joint developed at the expense of stability The freeing of the upper limb motion is also partly due to mobility of the pectoral girdle that link the limb to the trunk Shoulder complex Shoulder complex Acromioclavicular Joint Glenohumeral Joint Shoulder complex Scapulothoracic Joint Glenohumeral Ligament Shoulder complex Corocohumeral Ligament Shoulder complex Shoulder Girdle Muscles ◦ Trapezius ◦ Upper Trapezius- Elevates scapula ◦ Middle Trapezius- Retract scapula ◦ Lower Trapezius- Retract and depress scapula Shoulder complex Shoulder Girdle Muscles Serratus Anterior- Protracts scapula Shoulder complex Glenohumeral Muscles Rotator Cuff Supraspinatus- Shoulder abduction Infraspinatus- Lateral / External rotation of shoulder Teres Minor- Lateral / External rotation of shoulder Subscapularis- Medial / Internal rotation of shoulder Shoulder complex Glenohumeral Muscles oLatissimus Dorsi- Extension, adduction and medial rotation of shoulder Shoulder complex Glenohumeral Muscles ◦ Deltoid Anterior – flexion and medial rotation Middle – abduction Posterior – extension and lateral rotation Shoulder complex Biceps- Elbow flexion Triceps- Elbow extension Shoulder complex Range of motion Flexion - 150 - 1800 Extension - 50 - 600 Abduction - 150 - 1800 External rotation - 900 Internal rotation - 70 - 900 Shoulder Arthtokinematics Movement of the joint surface Sliding and rolling Convex surface of on concave surface Movement of the bone is in opposite direction to the body segment Shoulder Osteokinematics oAbduction oAdduction oFlexion oExtension oExternal rotation oInternal rotation Rotatorcuff Approximates humerus to function Supraspinatus assists deltoid in abduction Subscapularis, infraspinatus & teres minor depress humeral head Subscapularis Effective restraint to ER (external rotation) with arm at side Ineffective restraint to ER(external rotation) with arm abducted to 900 Force Couple oAnterior vs Posterior components o Medial vs lateral components o Maintain stability of the joint oTransverse plane: Anterior vs posterior Rotator cuff o Coronal plane: Deltoid vs. Rotator cuff Scapulothoracic motion Force Couple at ST Joint oSerratus anterior produces anterio-lateral movement of inferior angle o Upper trapezius pulls scapula medially Scapulohumeral Rhythm The overall ratio of 20 of GH to 10 of ST motion during arm elevation is commonly used, and the combination of concomitant GH and ST motion most commonly referred to as scapulohumeral rhythm. Total elevation: 1200 at G-H joint 600 at S-T joint Scapulohumeral Rhythm Scapular motion The scapula contributes to elevation of the arm not only by upwardly rotating on the thorax but also through other ST motions. Upward/Downward Rotation Upward rotation of the scapula on the thorax is the principal motion of the scapula observed during active elevation of the arm and plays a significant role in increasing the range of elevation of the arm overhead. Scapular motion Elevation/Depression Scapular elevation and depression can be isolated by shrugging the shoulder up and depressing the shoulder downward. Protraction/Retraction Protraction and retraction of the scapula on the thorax are often described as translatory motions of the scapula away from or toward the vertebral column respectively. Scapular motion Internal/External Rotation Internal/external rotation of the scapula on the thorax should normally accompany protraction/retraction of the clavicle at the SC joint. Anterior/Posterior Tipping Anterior/posterior tipping of the scapula on the thorax occurs at the AC joint and normally will accompany anterior/posterior rotation of the clavicle at the SC joint. Biomechanics of Scapular Rotation Scapulothoracic motion occurs in close kinetic chain involving: AC joint SC joint Biomechanics of Scapular Rotation Phase I Upper & lower portions of trapezius & serratus anterior produce upward rotatory force on scapula. Motion at A-C joint prevented by coracoclavicular ligament Rotation of scapula occurs as elevation of clavicle at S-C joint Biomechanics of Scapular Rotation Phase II Further motion at S-C joint prevented by costoclavicular ligament Continued upward rotation of scapula pulls on costoclavicular ligament causing posterior rotation of clavicle Posterior rotation of clavicle allows further upward rotation of scapula Elbow complex Elbow complex Elbow complex Elbow complex Elbow complex Elbow complex Humeroulnar articulation True elbow joint Hinge joint Allow flexion & extension of elbow Humeroradial articulation It slides along the capitulum No physiological movement Proximal radioulnar articulation Allow supination and pronation Reinforce by annular and interosseous ligament Elbow complex Elbow complex Elbow complex Brachialis Action: Elbow flexion in pronation Innervation: Musculocutaneous nerve (C5, C6) Elbow complex Biceps brachii Action: Elbow flexion in supination Innervation: Musculocutaneous nerve (C5, C6) Elbow complex Brachioradialis Action: Elbow flexion in neutral position Innervation: Radial nerve (C5, C6, C7) Elbow complex Triceps Action: Elbow extension Innervation: Radial nerve (C6, C7, C8) Elbow complex Anconeus Action: Elbow extension (lock extension) Innervation: Radial nerve (C6, C7, C8) Elbow complex Pronators Elbow complex Supinator Action: Supination of forearm Innervation: Radial nerve (C6, C7, C8) Elbow complex Humeroulnar joint: Hinge joint; allow motion only in 1 plane Arthrokinematic: rolling of olecranon process of ulna inside olecranon fossa. Movement of the concave surface on convex surface; joint motion is in the same direction as body segment (refers concave convex rule) Osteokinematic: flexion and extension of elbow Elbow complex Elbow complex Radioulnar Joint: It is a uniaxial pivot joint; motion in 1 plane. Arthrokinematic: sliding of head of radius on capitulum of humerus. Osteokinematic: supination and pronation forearm. Elbow complex Motion of elbow axis The axis for flexion and extension is a fixed axis that passes horizontally through the center of the trochlea and capitulum and bisects the longitudinal axis of the shaft of the humerus. Cubital angle Lateral deviation of the ulna in relation to the humerus that results from the throchlear notch projection on the medial side. This angulation also known as cubitus valgus or carrying angle. The average angle is 15 degree. Cubital angle An increase in the carrying angle beyond the average is considered to be abnormal, especially if it occurs unilaterally. A varus angulation (medially deviated) at the elbow is referred to as cubitus varus and is usually abnormal. Normally, the carrying angle disappears when the forearm is pronated and the elbow is in full extension and when the supinated forearm is flexed against the humerus in full elbow flexion. Wrist Motion & Kinematics FLEXION / EXTENSION The movement at radiocarpal and midcarpal joints take place at the same time during flexion and extension of the wrist. Total ROM of flexion and extension of the wrist is therefore 85 degree in each direction Arthrokinematic: sliding at the radiocarpal articulation and rolling at the mid carpal articulation The joint surface motion apply the convex move on concave rule. The axis for flexion and extension is at the transverse axis of the capitate Wrist Motion & Kinematics RADIAL / ULNAR DEVIATION The total range for radial and ulnar deviation is the sum of possible motion at the radiocarpal and mid carpal joints Radial deviation has range of 15 degree and ulnar deviation has the range of 45 degree The radial deviation is limited by the projection of radial styloid process that located more distally. Arthrokinematic: Sliding and rolling motion at the radiocarpal mid carpal joint. The axis for radial and ulnar deviation is proximal to the axis of flexion and extension on capitate as it passing through the axis Kinematics of Lowerlimb joints Anatomical Structures of the Hip Acetabulum Acetabular labrum Articular cartilage Femoral head Joint capsule and ligaments Ligamentum teres Hip musculature Femoral Angle of Inclination Angle of Inclination – angle within the frontal plane between the femoral neck and the medial side of the femoral shaft Average adult measurement of 125 degrees Newborns born with 140-150 degree angle which reduces to approximately 125 degrees with onset of standing/walking Coxa Vara – A of I markedly less than 125 degrees Coxa Valga – A of I markedly greater than 125 degrees Femoral Angle of Torsion Torsion angle – relative rotation (twist) that exists between the shaft and neck of the femur Average adult measurement of 10-15 degrees of anterior rotation (Anteversion) of femoral head Newborn TA typically 30 degrees of anteversion; which reduces to 10-15 degrees by 6 years of age Femoral Angle of Torsion Normal Anteversion – 10-15 degrees allows optimal alignment and joint congruency Excessive Anteversion – TA significantly greater than 15 degrees Often associated with congenital dislocation in the infant; marked joint incongruence; and increased degenerative wear Compensated excessive anteversion may result in “toeing-in” gait pattern (“pigeon toed”) Retroversion – TA significantly less than 15 degrees (i.e. 5 degrees of anteversion) Less common than excessive anteversion Femoral Angle of Torsion Close pack position of hip CPP – unique position of the hip joint where the articular surface is most congruent and the ligaments are maximally taut Full passive hip extension coupled with slight internal rotation and abduction maximally stretches the capsule and maintains congruency Passive hip flexion coupled with internal rotation and abduction stretches the capsule but is moving away from joint congruency; this is a common pathological “capsular pattern” Osteokinematics of hip Femoral on Pelvic Motion F on P Motion occurs when hip joint action occurs as a result of the femur moving on a relatively fixed or stationary pelvis F on P motion can be closed or open chain movement Closed Chain: i.e. Squat; Stance phase of gait Open Chain: Standing hip extension exercise; Swing phase of gait Passive hip flexion limited to 80-90 degrees by hamstrings when knee is extended; otherwise to 120 degrees Passive hip extension is to 20 degrees with an extended knee; or to approximately 0 degrees with fully flexed knee due to rectus femoris tightness Abduction is to 40 degrees limited by capsular ligaments, adductor and hamstring muscles Femoral on Pelvic Motion Adduction limited to about 25 degrees due to interference from other limb, and/or due to abductor muscles, iliotibial band or hip capsule Internal Rotation is to about 35 degrees External Rotation is to about 45 degrees; may be limited by hip capsule, iliotibial band or tensor fascia latae muscle Lumbopelvic rhythm LPR – the kinematic relationship between the lumbar spine and hip joints during sagittal plane movement Ipsi-directional LPR – when pelvis and lumbar spine move in same direction; useful for activities such as extending the reaching capacity of the upper extremities Contra-directional LPR – when the pelvis and the lumbar spine move in opposite directions; seen in walking, dancing, or any other activity in which the position of the supralumbar trunk must be held fixed Lumbopelvic rhythm Pelvic on Femoral Movement Flexion/Extension Standing Flexion – limited to 80-90 degrees; ipsi-directional LPR Standing Extension – limited to about 20 deg; contra directional LPR Pelvic tilt – anterior or posterior movement of the pelvis (reference point on iliac crest) on stationary femoral heads, offset by contra-directional LPR Pelvic tilt Anterior Pelvic Tilt Posterior Pelvic Tilt Pelvic on Femoral Movement Abduction – active hip hiking of opposite hip/pelvis via contra directional LPR; limited to 30 degrees by lumbar spine ROM Adduction – accomplished through lowering of opposite hip/pelvis via contra directional LPR; total adduction determined by lateral myofascial elements in non- pathological hip rather than by lumbar spine ROM Pelvic on Femoral Movement Internal Rotation – forward rotation (anterior) of opposite hip/pelvis in the horizontal plane External Rotation – backward (posterior) of opposite hip/pelvis in the horizontal plane Lumbopelvic stabilization Dual action of muscle Hip Abductors & Pelvic Stabilization Poor pelvic stabilization can occur as a result of functionally weak hip abductor muscles; or as a result of underlying pathology (muscular dystrophy, hip arthritis, hip instability). Trendelenburg sign – dropping of the opposite hip and pelvis upon single leg support; indicates poor pelvic stabilization; does not identify cause of instability May be compensated for by leaning over the unstable hip Posterior Pelvic tilt Knee Joint Knee joint proper (tibiofemoral joint) Classified as a ginglymus joint Sometimes referred to as trochoginglymus joint internal & external rotation occur during flexion Some argue for condyloid classification Patellofemoral joint Arthrodial classification Gliding nature of patella on femoral condyles Osteokinematics of Knee The ligaments and menisci provide static stability and the muscles and tendons dynamic stability. The main movement of the knee is flexion - extension. For that matter, knee act as a hinge joint, whereby the articular surfaces of the femur roll and glide over the tibial surface. During flexion and extension, tibia and patella act as one structure in relation to the femur. The quadriceps muscle group is made up of four different individual muscles. They join together forming one single tendon which inserts into the anterior tibial tuberosity, embedded in the tendon is the patella, a triangular sesamoid bone and its function is to increase the efficiency of the quadriceps contractions. Osteokinematics of Knee Contraction of the quadriceps pulls the patella upwards and extends the knee. Range of motion: extension 0o. The hamstring muscle group consists of the biceps femoris, semitendinosus and semimembranosus. They are situated at the back of the thigh and their function is flexing or bending the knee as well as providing stability on either side of the joint line. Range of motion: flexion 140o. Secondary movement is internal - external rotation of the tibia in relation to the femur, but it is possible only when the knee is flexed. Arthrokinematics of Knee Open kinetic chain - During knee extension, tibia glides anteriorly on femur. More precisely, from 20o knee flexion to full extension, tibia rotates externally. During knee flexion, tibia glides posteriorly on femur and from full knee extension to 20o flexion, tibia rotates internally. Closed kinetic chain - During knee extension, femur glides posteriorly on tibia. To be more specific, from 20o knee flexion to full extension, femur rotates internally on stable tibia. During knee flexion, femur glides anteriorly on tibia and from full knee extension to 200 flexion, femur rotates externally on stable tibia. Screw home mechanism The "screw-home" mechanism, considered to be a key element to knee stability, it is the rotation between the tibia and femur. It occurs at the end of knee extension, between full extension (0o) and 20o of knee flexion. The tibia rotates internally during the open chain movements (swing phase) and externally during closed chain movements (stance phase). External rotation occurs during the terminal degrees of knee extension and results in tightening of both cruciate ligaments, which locks the knee. The tibia is then in the position of maximal stability with respect to the femur. Screw home mechanism Foot & Ankle The ankle or tibiotalar joint constitutes the junction of the lower leg and foot. The osseous components of the ankle joint include the distal tibia, distal fibula, and talus. The anatomic structures below the ankle joint comprise the foot, which includes: 1. Hindfoot: The hindfoot, the most posterior aspect of the foot, is composed of the talus and calcaneus, two of the seven tarsal bones. The talus and calcaneus articulation is referred to as the subtalar joint, which has three facets on each of the talus and calcaneus. 2. Midfoot: The midfoot is made up of five of the seven tarsal bones: navicular, cuboid, and medial, middle, and lateral cuneiforms. The junction between the hind and midfoot is termed the Chopart joint, which includes the talonavicular and calcaneocuboid joints. 3. Forefoot: The forefoot is the most anterior aspect of the foot and includes the metatarsals, phalanges (toes), and sesamoid bones. There are a metatarsal and three phalanges for each digit apart from the great toe, which only has two phalanges. The articulation of the midfoot and forefoot forms the Lisfranc joint Talocrural (TC) Joint The talocrural joint is formed between the distal tibia-fibula and the talus, and is commonly known as the ankle joint. The distal and inferior aspect of the tibia is connected to the fibula via tibiofibular ligaments forming a strong mortise which articulates with the talar dome distally. It is a hinge joint and allows for dorsiflexion and plantarflexion movements in the sagittal plane. Subtalar Joint It is also known as the talocalcaneal joint and is formed between the talus and calcaneus. There are three facets on each of the talus and calcaneus. The posterior subtalar joint constitutes the largest component of the subtalar joint. The subtalar joint allows for ankle and hindfoot inversion and eversion. Midtarsal Joint It is also known as transverse tarsal joints or Chopart’s joint. It is an S- shaped joint when viewed from above and consists of two joints – the talonavicular joint and calcaneocuboid joint. 1.Talonavicular (TN) Joint - Formed between the anterior talar head and the concavity on the navicular. It does not have its own capsule, but rather shares one with the two anterior talocalcaneal articulations. 2.Calcaneocuboid (CC) Joint - Formed between the anterior facet of the calcaneus and the posterior cuboid. Both articulating surfaces present a convex and concave surface, with the joint being convex vertically and concave transversely. Very little movement occurs at this joint. Midtarsal Joint Talocrural (TC) Joint The tip of the medial malleoli is anterior and superior to the lateral malleoli, which makes its axis oblique to both the sagittal and frontal planes. The axis of rotation is approximately 13°-18° laterally from the frontal plane and at angle of 8°-10° from the transverse plane. Motion in other planes is required (like horizontal and frontal plane) to achieve a complete motion for plantarflexion and dorsiflexion. The reported normal available range for dorsiflexion varies in the literature between 0°-16.5° and 0°-25°, and this changes when in weightbearing. The normal range of plantarflexion has been reported to be around 0°- 50° Subtalar Joint The axis of the subtalar joint lies about 42° superiorly to the sagittal plane and about 16° to 23° medial to the transverse plane. The literature presents vast ranges of subtalar motion ranging from 5° to 65°. The average ROM for pronation is 5° and 20° for supination. Inversion and eversion ROM has been identified as 30° and 18°, respectively. Total inversion-eversion motion is about 2:1 and a 3:2 ratio of inversion-to- eversion movement. Midtarsal Joint The midtarsal joint rotates at two axes due to its anatomy, making its motion complex. The longitudinal axis (image 'A' below) lies about 15° superior to the horizontal plane and about 10° medial to the longitudinal plane. The oblique axis lies about 52° superior to the horizontal plane and 57° from the midline. The longitudinal axis is close to the subtalar joint axis and the oblique axis is similar to the talocrural joint axis. Joint Closed- Open-Packed Capsular Pattern Concave Surface Convex Concave- Packed Position Surface convex Position rule Roll & glide Talocrural Full 10o of Limitation of Proximal - Mortise Distal - Opposite joint dorsiflexion plantarflexion and plantarflexion, formed by Tibia, Trochlear direction midway between although clinically tibiofibular surface of pronation and dorsiflexion limitation ligament and Talar dome supination is more common fibula Subtalar Full inversion Inversion/plantarfl Limitation of inversion Proximal - Distal – Opposite joint exion in chronic arthritis. Anterior, middle Calcaneal direction Limitation of eversion and posterior Anterior, in traumatic facet of talus middle and posterior talar articular surface Joint Closed- Open-Packed Capsular Pattern Concave Convex Surface Concave- Packed Position Surface convex rule Position Roll & glide Talonavicula Full Midway between Limitation of Proximal - Head Distal - Concavity Same r joint supination extreme ROM dorsiflexion, of Talus on Navicular bone direction plantarflexion, for talus adduction and internal rotation. Calcaneocu Full Midway between Limitation of Distal - Cuboid Proximal - Flexion- boid joint supination extreme ROM dorsiflexion, is concave Calcaneus is extension = plantarflexion, during flexion- convex during Same adduction and extension. flexion-extension. direction internal rotation. Calcaneus is Cuboid is convex Adduction- concave during during adduction- abduction = adduction- abduction Opposite abduction direction Joint Closed-Packed Open-Packed Position Capsular Concave Convex Concave- Position Pattern Surface Surface convex rule Roll & glide Lisfranc joint Full supination Midway between supination and pronation 1st MTP joint Hyperextension Slight (10o) extension Loss of motion Same Proxmial - more in Distal - Base direction Head of extension than of phalanx Metatarsal flexion 2nd to 5th MTP Maximum Slight (10o) extension Loss of flexion Distal - Base Proximal - Same joint flexion of Head of direction phalanges metatarsals Interphalangeal Full extension Slight flexion Restriction in all Same Joint direction with Distal Proximal dirextion more in Phalanx Phalanx extension Arches of foot The arches of the foot provide functions of force absorption, base of support and acts as a rigid lever during gait propulsion. The medial longitudinal arch, lateral longitudinal arch and transverse arch are the 3 arches that compromises arches of foot. Medial Longitudinal Arch (MLA) It is the longest and highest of all the arches. Bony components include the calcaneus, talus, navicular, the three cuneiform bones and the first 3 metatarsals. The arch consists of two pillars: the anterior and posterior pillars. The anterior pillar consists of the head of first 3 metatarsal heads whereas the posterior pillar consists of the tuberosity of the calcaneus. The plantar aponeurosis forms the supporting beam connecting the two pillars. The apex of the MLA is the superior articular surface of talus. In addition to the plantar aponeurosis the MLA is also supported by the spring ligament and the deltoid ligament. The Tibialis anterior and posterior muscles play an important role in raising the medial border of the arch, whereas Flexor hallucis longus acts as bowstring. Lateral Longitudinal Arch (LLA) It is the lowest arch and compromises of the calcaneus, cuboid and fourth & fifth metatarsal as its bony component. Like the Medial Longitudinal Arch (MLA) the posterior pillar consists of the tuberosity of the calcaneus whereas the anterior pillar is formed by the metatarsal heads of 4th and 5th metatarsals. The plantar aponeurosis, and long & short plantar ligaments provide support for the LLA. The Peroneus longus tendon plays an important role in maintaining the lateral border of the arch. Transverse Arch It is concave in non-weight bearing and runs medial to lateral in the midtarsal and tarsometatarsal area. The bony component of the arch consists of the metatarsal heads, cuboids and 3 cuneiform bones. The medial and lateral pillars of the arch is formed by the medial and lateral longitudinal arch respectively. The arch is maintained by the Posterior tibialis tendon and the Peroneus longus tendon which cross the plantar surface from medial to lateral and lateral to medial respectively. Spine Intervertebral disc The intervertebral disc has two principle function which are: i. Separate between two vertebral bodies (allowing more available motion) ii. Transmit load from one body to the next body The size of the discs are varies depending on the amount of motion and magnitude of loading (increase in size and thickness from cervical to lumbar) The available motion of the vertebrae is depending on the ratio of the disc thickness to the vertebral body height (the greater the ratio, the higher the mobility) The intervertebral disc composed of three parts: (i) nucleus pulposus, (ii) annulus fibrosus & (iii) vertebral end plate Ligaments & Capsule The ligamentous system of vertebral column exhibits an extensive variability depending on the regions The main ligaments are: i. Anterior and posterior longitudinal ligaments ii. Ligamentum flavum iii. Interspinous ligament iv. Supraspinous ligament v. Intertransverse ligament Ligaments & Capsule Spinal motion Degrees of freedom is a useful concept in the description. No of unique independent motion one vertebra can have with respect to another. Six degrees of freedom Three translational, Three rotational, along three axes Significance of facet joint orientation In cervical spine, facets are oriented 45 degree to horizontal, almost in the coronal plane. In thoracic spine, the orientation is intermediate allowing axial rotation. In the lumbar spine, rotation is prevented by relatively sagittal orientation of the facets while flexion and extension is free. Coupling It is defined as obligatory movements of the spine (translations and rotations) that accompany a primary motion. Principal motion is defined as the motion produced in the plane of the force. Any associated out of phase motion is coupled Instantaneous axis of rotation It is defined as the axis perpendicular to the plane of motion and passing through the points within or outside the body which is static during the motion. Example, when opening a door, the axis of rotation passes through the hinges. Spinal stability Paramount concept: Ability of spine to maintain its pattern of displacement under physiologic loads without producing Incapacitating pain. Deformity. Neurological deficit. Cervical joint Cervical spine most mobile part of axial skeleton. Average range of flexion –extension at occipitoatlantal joint 13- 15 degree. Additional 10° occurs at atlantoaxial joint. Flexion extension evenly distributed over entire cervical spine Maximally at C5 –C6. Anterior-posterior translation between dens and anterior atlantal arch 3mm. When alar ligaments also rupture, >5 mm separation occurs. Cervical joint Axial rotation occurs only at atlantoaxial joint. Maximum limit of rotation is 40-45 degrees. Beyond 45 degree facets get locked. Rotation >32 degrees leads to angulation of contralateral vertebral artery. Thorocolumbar spine In thoracic spine lateral bending is evenly distributed. Upper thoracic spine- more of axial rotation. Lower thoracic spine more of flexion-extension. In lumbar spine, large amount of flexion-extension. Hence, PIVD more common at L4-L5 and L5-S1 levels. The bending moment developed at the vertebra is dependent on the length of the column and distance between the line of gravity and the centrum