Respiratory System - Chapter 15 - PDF
Document Details
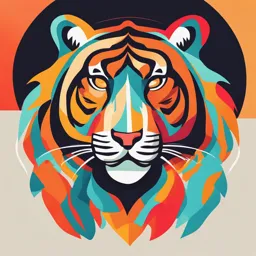
Uploaded by PrudentNaïveArt8869
Tags
Summary
This document provides a comprehensive list of key terms related to the respiratory system. It includes definitions and explanations for various respiratory conditions, processes, and structures. It is intended to be used as a reference guide for medical students or professionals.
Full Transcript
Key Terms acute respiratory distress syndrome (ARDS) adventitious breath sounds air trapping airway remodeling alpha-1 antitrypsin (AAT) anoxia aspiration aspiration pneumonia asthma atelectasis atopic atypical pneumonia auscultation barrel chest bronchiectasis capillary refill cent...
Key Terms acute respiratory distress syndrome (ARDS) adventitious breath sounds air trapping airway remodeling alpha-1 antitrypsin (AAT) anoxia aspiration aspiration pneumonia asthma atelectasis atopic atypical pneumonia auscultation barrel chest bronchiectasis capillary refill central cyanosis centriacinar emphysema chemoreceptors chronic bronchitis chronic obstructive pulmonary disease (COPD) clubbing compliance consolidation cor pulmonale costochondritis crackles cyanosis cystic fibrosis (CF) diffusing capacity diffusion dyspnea emphysema empyema exercise-induced asthma (EIA) expectorate expiration fibrosis forced expiratory volume in 1 second (FEV1) forced vital capacity (FVC) gastroesophageal reflux hemoptysis hepatization hyaline membrane hypercapnia hypoxemia hypoxia impaired diffusion insidiously inspiration mechanical ventilation mucus plugs nosocomial orthopnea oxygen saturation (SaO2) oxyhemoglobin (HbO2) PaO2 PaCO2 panacinar emphysema partial pressure perfusion peripheral cyanosis phlegm pleural effusion pleural friction rub pneumonia pneumothorax pulmonary edema pulmonary embolus pulsus paradoxus pursed lip breathing residual volume (RV) resistance respiration respiratory failure retractions sepsis sputum status asthmaticus surfactant systemic inflammatory response syndrome (SIRS) tidal volume (TV) total lung capacity (TLC) type I alveolar cells type II alveolar cells typical pneumonia ventilation ventilation--perfusion (V/Q) mismatching vital capacity (VC) Introduction The respiratory system is responsible for providing cells with necessary oxygen and ridding the body of carbon dioxide waste. It is made up of an upper respiratory tract and a lower respiratory tract. The two lungs are housed within the thoracic cavity. Gas exchange depends on the partial pressures and diffusion of dissolved gases. Respiratory function changes with age and disease. Take a deep breath. Exhale. Oxygen (O2) from the air goes in; carbon dioxide (CO2) goes out. When you breathe, four major processes are occurring as your chest rises and falls: ventilation, diffusion, perfusion, and respiration. Ventilation is the process of moving air into and out of the trachea, bronchi, and lungs. Diffusion is the process of moving and exchanging the oxygen acquired during ventilation with carbon dioxide waste across the alveolar--capillary membranes. Perfusion is a process of supplying oxygenated blood to the lungs and organ systems via the blood vessels. Respiration is a process in which cells throughout the body use oxygen aerobically to make energy. As a healthcare provider, it is critically important to recognize problems associated with altered ventilation and diffusion. Module 1 Pulmonary Structure and Function The pulmonary, or respiratory, system is responsible for ventilation and diffusion. The major function is the exchange of gases between the environment and the blood. The pulmonary system comprises the lungs, airways, chest wall, and pulmonary circulation (Fig. 15.1). The structural path of the pulmonary system is often divided into the upper and lower airways. The upper airway comprises the nose, mouth, nasopharynx, and oropharynx. The laryngeal pharynx connects the upper and lower airways. The lower airway comprises the trachea, bronchi, and bronchioles. The branching anatomic structure of the pulmonary system is ideal for maximizing surface area to allow for optimal gas diffusion, or movement of gases across the alveoli. Because the pulmonary system is vulnerable to the external environment, defense mechanisms are needed to protect the lungs from environmental injury. These defense mechanisms are highly effective and include: Protective structures, such as hairs and turbinates (shell-shaped structures) in the nose and cilia in the upper and lower airways, which trap and remove foreign particles from the air Mucosal lining of the upper and lower airways, which warms and humidifies air Irritant receptors throughout the nose and airways, which recognize injurious agents and respond by triggering a sneeze or cough reflex to remove foreign particles Immune protections, such as an immune coating in the respiratory tract mucosa and macrophages in the alveoli, which ingest and remove bacteria and other foreign materials via phagocytosis Nath4e-Hand-icon Stop and Consider How are defense mechanisms affected in the person who is a mouth breather? Intercostal muscles and ribs surround the lungs, providing protection against injury. The muscles of the chest cavity and the diaphragm perform the muscular work of breathing. Two major membrane layers line the lungs and chest cavity: the (outer) parietal pleura and (inner) visceral pleura. These layers are separated by a pleural space, which is filled with a lubricating fluid, secreted by the pleura, that allows the two layers to slide over each other with minimal friction. The pulmonary circulation is responsible for delivering oxygen and other nutrients to the lung tissues. It is also responsible for filtering clots, air, or other foreign materials from the circulation. The capillaries of the lungs near the alveoli facilitate the exchange of oxygen and carbon dioxide. Ventilation The process of ventilation involves both acquiring oxygen (inspiration) and removing carbon dioxide (expiration) from the blood. The transport of oxygen through the lungs is the only mechanism for acquiring oxygen. Oxygen is necessary for cellular metabolism, which means that for the cell to expend energy efficiently and perform its designated function, oxygen must be present. The release of carbon dioxide is equally as important. Optimal cell functioning occurs within a very narrow pH range. The release and retention of carbon dioxide is one mechanism for maintaining this balance. The rate and volume of ventilation is regulated by the following components: Respiratory control centers in the brain Lung receptors Chemoreceptors The drive to ventilate is stimulated by the respiratory control center in the brainstem in response to chemical messages in the body. The respiratory control centers comprise neurons in the pons and medulla, which send neural impulses to the diaphragm, intercostal muscles, sternocleidomastoid muscles, and other accessory muscles, causing them to contract or relax. The autonomic nervous system (ANS) also innervates the lungs and acts on the smooth muscles of the conducting airways to promote airway constriction (parasympathetic division) or dilation (sympathetic division). The activity of the ANS will increase or decrease the diameter of the airways and affect the amount of air that is able to get in and out of the lungs. Neural impulses are directed by lung receptors that map the current state of breathing and lung function. Lung receptors are located in the epithelium and smooth muscle of the airways, and they are near the alveolar--capillary junctions. Specific lung receptors have particular roles. For example, lung receptors located in the epithelium of the conducting airways are responsible for sensing irritants in the lungs and stimulating the cough reflex. Lung receptors in the smooth muscles of the airways are activated to promote expiration in order to prevent excessive lung inflation. Lung receptors in the capillaries detect increases in capillary pressure and stimulate a reduction in this pressure. Chemoreceptors detect gas exchange needs based on the partial pressures of oxygen (PaO2) and carbon dioxide (PaCO2), along with the pH levels in the blood and cerebrospinal fluid (CSF). Central chemoreceptors are located near the respiratory control center and respond to pH changes in the CSF. Because CO2 diffuses across the blood--brain barrier until both the arterial PaCO2 and CSF PaCO2 are equal, the CO2 levels detected by the central chemoreceptors reflect that in the blood. The central chemoreceptors then alter the rate of breathing to adapt to the higher or lower levels of CO2 in the body. Recall that carbon dioxide is acidic. If the blood is more acidic, the respiratory centers increase the rate and depth of breathing to release or "blow off" excess carbon dioxide. If the blood is more alkaline, the respiratory centers decrease the rate and depth of breathing to retain carbon dioxide. Peripheral chemoreceptors, which are most sensitive to oxygen levels in the arterial blood (PaO2), are located in the aorta and carotid arteries. Peripheral chemoreceptors trigger an increase in ventilation in response to low oxygen levels in the blood. Cervical and thoracic nerves are then stimulated to adapt breathing as necessary. Nath4e-Hand-icon Stop and Consider How does your breathing change when you exercise? Why do you think this happens? The messages sent by the lung and chemoreceptors are sent to the cervical and thoracic nerves, which act on the pulmonary system to adapt the breathing pattern to maintain homeostasis. The work of breathing is improved with strong muscles of ventilation, such as the intercostal muscles, diaphragm, and sternocleidomastoid muscles, along with proper lung compliance (allows maximal inspiration), elastic recoil (promotes expiration), and low airway resistance. Compliance is distensibility, or expandability, of the lung tissue and chest wall. Airway resistance is based on the area of the inner lumen of the airway and on properties, such as viscosity and velocity, of the gas moving through that airway. INSPIRATION Inspiration is the process of breathing in to acquire oxygen. Similar to inflating a balloon, movement of air is always unidirectional and travels from an area of high pressure to low pressure. In this example, the pressure inside the balloon (the "lungs") is lower than that of the person blowing air into the balloon (the "atmosphere"). A major difference is that the chest cavity changes size to alter the pressure gradient, thereby drawing air into the lungs, as opposed to the air moving in and changing the size, as you would see with a balloon. Neural stimulation and the movement of the diaphragm downward and external intercostal muscles outward allow this increase in chest cavity size, reduce pressure inside the lungs, and pull air into the lungs. EXPIRATION Expiration, the process of removing carbon dioxide out of the body through the lungs, is also regulated within the respiratory centers of the brain. In expiration, the diaphragm and external intercostal muscles relax. The chest wall moves inward and the diaphragm moves upward. The intrathoracic (inside the thoracic cavity) pressure becomes greater than the atmospheric pressure. This stimulates air to flow passively out into the atmosphere. Exhalation can also be forced if the abdominal and intercostal muscles are contracted voluntarily, thereby increasing pressure to move the diaphragm upward and pull the ribs inward. The idea is to compress the lungs and increase the pressure inside the airways. Air then moves from the region of higher to lower pressure, or, in this example, from the lungs to the atmosphere. MEASUREMENT OF VENTILATION Lung volume measurements can determine the effectiveness of inspiration and expiration. Measurements are done by breathing in or out through a spirometer where measurement of air volume occurs. Changes in lung volumes or pulmonary function can be an early sign of impaired ventilation. Predicted values (the measurement readings that are expected) vary based on age, height, and sex. The following are examples of major measurements of ventilatory capacity: Tidal volume (TV) is the amount of air that is exhaled after passive inspiration; this is the volume of air going in and out of the lungs at rest; in adults, this volume is approximately 500 mL. Vital capacity (VC) is the maximal amount of air that can be moved in and out of the lungs with forced inhalation and exhalation. Forced vital capacity (FVC) is the maximal amount of air that is exhaled from the lungs during a forced exhalation. Forced expiratory volume in 1 second (FEV1) is the maximal amount of air that can be expired from the lungs in 1 second. Residual volume (RV) is the volume of air that remains in the lungs after maximal expiration. Total lung capacity (TLC) is the total amount of air in the lungs when they are maximally expanded and is the sum of the VC and RV. DIFFUSING CAPACITY The ability of the alveolar--capillary junction to exchange oxygen and carbon dioxide between the atmosphere and the blood can be measured quantitatively. The diffusing capacity is defined as a measurement of carbon monoxide (CO), oxygen, or nitric oxide transfer from inspired gas to pulmonary capillary blood and reflects the volume of a gas that diffuses through the alveolar--capillary membrane each minute. This volume is determined most commonly by comparing how much carbon monoxide at nontoxic levels is taken up by the blood and dividing this by the pressure across the alveolar--capillary membrane. Individuals with alveolar fibrosis or obstruction would be appropriate candidates for undergoing an analysis of diffusing capacity. During the test, the individual breathes in a gas containing CO and one or more tracer gases to determine the gas-exchanging capability of the lungs. For example, the oxygen-diffusing capacity in a state of rest averages about 21 mL per minute per mmHg. If the average pressure difference between the alveoli and arterial blood is 11 mmHg, the amount of oxygen that diffuses per minute would average 21 × 11 = 230 mL. Nath4e-Hand-icon Stop and Consider Based on what you know about why and how SaO2, PaO2, and PaCO2 are measured, what factors do you think would affect these readings? Module 2 Impaired Ventilation Impaired ventilation is a problem of blocking airflow in and out of the lungs, thereby restricting oxygen intake and carbon dioxide removal from the body. Two major mechanisms are implicated: (1) compression or narrowing of the airways and (2) disruption of the neural transmissions needed to stimulate the mechanics of breathing. Compression or narrowing of the airways anywhere from the nose or mouth to alveoli increases airway resistance and leads to difficulties with airway clearance. This occlusion can be partial or complete. Processes that contribute to ineffective airway clearance include inflammation, edema, and exudate accumulation from an infectious process; a structural narrowing of the passageway; strangulation; or the presence of a foreign body. In these cases, air is restricted from moving in and out of the body. Disruption of neural transmission to the lungs also alters ventilatory capacity by ignoring the messages sent by chemoreceptors and lung receptors and interrupting the mechanics of breathing. Oversedation during a surgical procedure or a drug overdose can promote a loss of neural stimulation in the respiratory centers. Damage to the respiratory centers of the brain, cervical nerves, or thoracic nerves leads to an unresponsive or ineffective breathing pattern that does not adapt to oxygen intake needs and carbon dioxide removal needs of the body. For example, severing the cervical nerves results in cessation of spontaneous lung function and requires external mechanical ventilation. Table 15.1 highlights effective and ineffective breathing patterns related to select physiologic and pathophysiologic processes. When a person's breathing pattern is responsive, the rate, depth, and rhythmic pattern of breathing adapt to physiologic changes occurring in the body. Breathing patterns become ineffective when these qualities (rate, depth, and rhythm) do not successfully maintain homeostasis, particularly in maintaining acid--base balance. All of the clinical models in this chapter demonstrate some level of ineffective airway clearance and modification in breathing patterns related to obstructive, restrictive, or altered neural processes. Impaired Ventilation--Perfusion Matching A second mechanism for impairing gas exchange is termed ventilation--perfusion (V/Q) mismatching. This means either that areas of the lung are ventilated but not perfused or that the lung is perfused but not ventilated. With impaired ventilation, inadequate oxygen comes into the lungs even though the blood flow is ready and able to carry the oxygen that is present. If the perfusion aspect is impaired, the blood flow to the lungs is restricted in one or more areas. Oxygen may be coming into the body but there is no blood flow to carry this away to other body cells. Impaired Diffusion Impaired diffusion is a process of restricting the transfer of oxygen or carbon dioxide across the alveolar--capillary junction. Because the rate of diffusion depends on the solubility and partial pressure of the gas, and on the surface area and thickness of the membrane, impaired gas exchange can occur with changes in any of these properties. All of the clinical models in this chapter demonstrate some level of impaired gas exchange related to a decrease in the functional alveolar--capillary junction surface area. Partial pressure is increased when more molecules are packed into a space, when the temperature increases (promoting an increase in particle collisions), and when the barometric pressure increases. A loss of partial pressure, and subsequent decrease in oxygen, can occur during a state of oxygen deprivation, during hypothermia, or when the body is responding to changes in atmospheric pressure (high altitude). The partial pressure of oxygen generally increases during the administration of high concentrations of oxygen and potentially during a fever. The partial pressure of carbon dioxide is increased during states of greater tissue metabolism, such as occurs with fever or strenuous exercise. This results in higher levels of carbon dioxide in the blood. Changes in the alveolar--capillary membrane also impair diffusion. Damage to the alveoli or capillaries limits the accessible and usable surface area and restricts oxygen and carbon dioxide transport. Many disease processes, such as pneumonia, pulmonary edema, and acute respiratory distress syndrome (ARDS), fill the alveolar--capillary junction with the products of inflammation or infection. This obstructs the passage of gases needed for cell function and for the maintenance of optimal pH in the blood. Chronic injury to the alveoli, such as with emphysema, can lead to fibrosis, or thickening of the alveolar--capillary membrane, and can also impair gas exchange. The Effects of Impaired Ventilation and Diffusion Many conditions, even diseases outside of the respiratory system, can challenge the ventilatory and diffusion capabilities of the body. Any situation that presents a demand for higher levels of oxygen or an increase in cellular metabolism requires pulmonary adaptation to maintain homeostasis. For example, strenuous exercise requires extensive adaptation to provide adequate oxygen to cells and to remove the excess carbon dioxide (as a byproduct of cellular metabolism). When the body is unable to keep up with the demands, either because the demands are too great or the ventilation or diffusion capabilities are restricted, this can lead to: 1\. Hypoxemia 2\. Hypoxia 3\. Hypercapnia These three effects directly result from decreased oxygen presence or utilization, or the retention of carbon dioxide. Basically, the body is unable to take in enough oxygen or is unable to release enough carbon dioxide. These basic effects directly apply to the selected clinical models in this chapter and all other conditions that affect ventilation and diffusion. Hypoxemia and Hypoxia Hypoxemia is decreased oxygen in the arterial blood leading to a decrease in the partial pressure of oxygen (PaO2). The major causes of hypoxemia include oxygen deprivation, hypoventilation, problems with adequate diffusion, and inadequate uptake of oxygen in the blood. Hypoxemia can range from mild to severe and becomes problematic when cells are deprived of adequate oxygen, a condition referred to as hypoxia. When hypoxemia leads to hypoxia, the effects are widespread. All cells that depend on oxygen for efficient cellular metabolism are vulnerable, particularly cells within the vital organs (brain, heart, and lungs). The brain has an incredibly high demand for oxygen and has minimal storage capacity. Oxygen deprivation throughout the body results in reduced cell metabolism and function. This, in turn, forces the cell to use anaerobic metabolism. Anaerobic metabolism leads to the rapid development of metabolic acidosis. The body needs to maintain a relatively constant pH for optimal cell functioning. Cellular death results from extreme or prolonged hypoxia. The cerebral effects of chronic hypoxia can range from restlessness (an early sign, particularly in children) to lethargy, coma, and, eventually, death. Although hypoxemia can lead to hypoxia, hypoxia can result even when there is adequate arterial oxygen. For example, a reduction in circulation caused by an arterial blockage can deprive cells distal to the blockage of oxygen even though the total amount of circulating oxygen is adequate. In this case, cell death results only in those cells deprived of oxygen. Hypercapnia Hypercapnia refers to a state of increased carbon dioxide in the blood. Carbon dioxide is much more easily diffused than oxygen; therefore, hypercapnia presents only in cases of severe alveolar hypoventilation and subsequent hypoxia. Conditions that inhibit ventilation or promote trapping of air in the alveoli contribute to the development of hypercapnia. The major effect is respiratory acidosis caused by CO2 retention. This can lead to electrolyte disturbances, which can alter cardiac conduction and brain function, resulting in an ineffective heart rhythm, coma, and death. Determining the level of hypercapnia requires measurement of arterial blood gases. Figure 15.3 summarizes the processes from ventilation and diffusion to hypoxemia, hypoxia, and hypercapnia GENERAL MANIFESTATIONS OF IMPAIRED VENTILATION AND DIFFUSION Although some subtle variations exist across conditions, the local and systemic clinical manifestations of altered ventilation and diffusion have many commonalities. Local manifestations (those triggered in the airways and lung tissues) are most often related to inflammatory processes in response to injury. The injury triggers vasodilation, increased capillary permeability, exudate formation, and pain in the affected region of the airways, lungs, or chest cavity. Potent chemical mediators regulate these inflammatory processes and are responsible for many of the local and systemic manifestations. The following are potential local manifestations: Cough Excess mucus Hemoptysis Dyspnea Use of accessory muscles Chest pain Barrel chest A cough, with or without excessive sputum, is a common clinical manifestation of altered ventilation and diffusion. The cough reflex is a protective mechanism that is triggered to rid the airways of an irritating agent. An acute cough typically lasts less than 3 to 8 weeks and is commonly associated with viral infections, seasonal allergies, aspiration (inhaling a foreign substance into the lungs), or pulmonary embolus (a blockage that occludes a pulmonary blood vessel). A chronic cough is one that extends beyond 8 weeks and is commonly associated with asthma, gastroesophageal reflux (gastric contents back up into the esophagus and stimulate the cough reflex), or chronic postnasal drainage. In smokers, chronic cough is a common manifestation of chronic bronchitis or lung cancer. Excess mucus production of the airways is related to the inflammatory response. This mucus is often the irritating agent that triggers the cough reflex and often provides clues to the offending agent or process. This condition can be observed by having the individual expectorate or spit out mucus from the throat or lungs. Sputum refers to this expectorated material. Phlegm is a term that describes large amounts of sputum expectorated from the oropharynx. Specific pathogens may produce sputum with a distinct color or odor. For example, bacterial infections are often characterized by thick, purulent sputum. Pneumococcal pneumonia may lead to rust-colored, dark sputum. Typically, the onset of a viral acute upper respiratory infection (a common cold) begins with clear, thin mucus. As phagocytes move into the area of inflammation to ward off any infection, these cells then die and are shed off as yellow or green mucus. Hemoptysis (coughing up blood from the respiratory tract) is defined by the presence of red blood cells in the sputum. Blood in the sputum is often significant and can be caused by heavy exertion during coughing (blood streaking within the mucus) or from tuberculosis, a tumor, or more severe trauma. When combined with edema in the lung tissues (pulmonary edema), the sputum often becomes not only blood-tinged but also frothy or foamy. Difficulty breathing is a common report in individuals with altered ventilation or diffusion. Dyspnea is the subjective feeling of shortness of breath or the inability to get enough air. The stimulus is based on the presence of hypoxemia, hypercapnia, and the subsequent reduction in pH; however, the exact mechanism is often unknown. One theory is that lung receptors or chemoreceptors act on the brain and breathing muscles to stimulate this sensation. Dyspnea often leads to certain physical and emotional responses, such as the physical need to sit in an upright or standing position, a condition called orthopnea, to maximize lung expansion. An individual may also use accessory muscles and demonstrate nasal flaring to help with breathing. Retractions are the pulling in of accessory muscles usually in the intercostal, substernal, and supraclavicular spaces to promote more effective inspiration. Along with position and effort changes, individuals experiencing air trapping may perform pursed lip breathing, a process of holding the lips puckered tightly together while slowly exhaling, to maintain positive airway pressure in the alveoli to minimize air trapping and promote expiration of carbon dioxide. Emotional manifestations of dyspnea include anxiety, a sense of panic, frustration, and anger. Chest pain can originate in the visceral and parietal pleura, the airways, or the chest wall. Inflammatory processes within the pleura often lead to pleural pain, which increases with deep inspiration and is often described as sharp or stabbing pain. Auscultation (listening with a stethoscope) will reveal a grating, scratching sound (called a pleural friction rub) with inspiration. Costochondritis, inflammation of one or more costal cartilages, is also characterized by pain in the anterior chest wall and may be triggered by coughing. Similarly, inflammation of the airways, intercostal muscles, or accessory muscles can also present as localized pain and be triggered by coughing. Chest pain, although frequently of pulmonary origin, must be differentiated from cardiac pain because of the possibility of the individual experiencing a myocardial infarction. Changes in the shape of the chest wall, known as barrel chest, can also occur with chronic lung disease. Typically, the chest wall shape is an oval, in which the anterior--posterior (AP) plane is narrower than the transverse (T) plane (an AP:T ratio of approximately 1:2). Chronic dilation and distention of the alveoli, as seen in emphysema, often results in a barrel chest appearance, in which the AP:T ratio becomes 1:1 (Fig. 15.4). Changes in breathing patterns (see Table 15.1) and in the characteristics of breath sounds are also common local manifestations of altered ventilation and diffusion. The sounds emitted by the lungs during inspiration and expiration also give clues to the presence and extent of altered ventilation and diffusion. Adventitious breath sounds are altered lung sounds resulting from airway constriction or fluid accumulation (Box 15.1). Airway constriction results in wheezing breath sounds. Fluid accumulation leads to fine or coarse crackles. The characteristics of these sounds vary based on anatomic location (larger vs. smaller airways) or type of secretions (thin, watery vs. thicker mucus). BOX 15.1 Adventitious Breath Sounds Crackles are snapping, popping, or bubbling sounds emitted during inspiration and expiration and caused by fluid accumulation in the airways; fine crackles are higher pitched with a shorter duration and signify fluid in smaller airways; coarse crackles are louder and lower pitched and signify fluid in the larger airways. Rales is a previously used term to describe fine crackles from fluid secretions in the airways; the term rales has been replaced with crackles. Wheezing is a continuous, high-pitched, whistling sound; it is significant for obstruction or tightness in the small airways. Rhonchi is a term used to describe both low-pitched wheezing sounds with a snoring quality (sonorous wheezing) when the airway narrowing is in the larger airways, and high-pitched wheezing sounds with a squeaking quality (sibilant wheezing) when the airway narrowing is in the smaller airways. Rhonchi occur when thick mucus partially blocks the airways. Stridor is a harsh, high-pitched, creaking sound, which is significant for obstruction in the upper airway, especially of the trachea or larynx. Diminished breath sounds is a term used to describe quieter breath sounds that are barely audible; this is significant for complete obstruction in one or more airways. Absent breath sounds signifies no air movement through the lungs. Systemic manifestations of altered ventilation are caused by the effects of hypoxemia, hypoxia, and hypercapnia. With inflammation, systemic manifestations, such as fever, malaise, leukocytosis, and higher levels of circulating plasma proteins, can apply to problems of altered ventilation and diffusion. The intensity of the hypoxic or hypercapnic response is variable depending on the extent of oxygen deprivation and carbon dioxide retention. Responses may include: Dusky or cyanotic mucous membrane color Changes in arterial blood gases Mental status changes Finger clubbing Changes in arterial blood gases, pH, and mental status are effects of hypoxemia, hypoxia, and hypercapnia. Changes in skin and mucous membrane color can also result from severe hypoxemia. Cyanosis is caused by a greater proportion of desaturated hemoglobin in the blood, which gives the blood a bluish hue. Central cyanosis, a problem of low oxygen saturation in the arterial blood, often presents as color changes in the skin and mucous membranes. In individuals with dark skin, the skin color change is often described as ashen or dusky. In light skin, the color is often described as pale or bluish. Because skin color varies among individuals, the most reliable indicator of central cyanosis on examination is the finding of a bluish color of the mucous membranes. Individuals with peripheral cyanosis, a problem of sluggish blood flow in the fingers and toes, often present with sluggish capillary refill (demonstrated by a slow return of blood when the nail bed is compressed and then released) and a pale or bluish hue in the nail beds. Chronic problems with ventilation and diffusion may result in clubbing of the fingers. Clubbing is a painless enlargement and flattening of the tips of fingers or toes caused by chronic hypoxia (Fig. 15.5). Although the exact mechanism is unknown, clubbing is consistently associated with chronic conditions that disturb oxygenation, such as prolonged obstruction or fibrosis of the airways. LABORATORY AND DIAGNOSTIC TESTS Detection of altered ventilation and diffusion is based on a thorough patient history and physical examination supported by relevant diagnostic and laboratory tests. Table 15.2 presents common laboratory and diagnostic tests performed when an individual has a suspected alteration in ventilation and diffusion. TREATING IMPAIRED VENTILATION AND DIFFUSION The treatment of any health condition is based on determining the cause. Occasionally, in conditions of altered ventilation and diffusion, the cause cannot be reversed. The principles that guide improving ventilation are based on: 1\. Removing obstructions (i.e., foreign body, tumor, edema) 2\. Restoring the integrity of the chest wall, lungs, and other respiratory structures 3\. Decreasing inflammation 4\. Decreasing, thinning, and moving mucus out of the airway 5\. Opening and maintaining integrity of the airways 6\. Supplementing oxygen 7\. Controlling infectious processes 8\. Using mechanical ventilation, as indicated Table 15.3 outlines common treatment modalities for impaired ventilation and diffusion odule 3 Clinical Models The following clinical models have been selected to aid in understanding and applying altered ventilation and diffusion processes and effects. Commonalities and unique features of each clinical model should be noted when reading this section. Pneumonia Pneumonia is selected as a clinical model to demonstrate the impact of a common acute infectious process on ventilation and diffusion. Pneumonia refers to inflammation of the lungs occurring commonly in the bronchioles, interstitial lung tissue, or the alveoli. Older and very young people, and those individuals who smoke or are immunosuppressed or hospitalized are most at risk for developing pneumonia. It is in the top 10 leading causes of death in the United States. PATHOPHYSIOLOGY Microorganisms, including bacteria, viruses, and fungi, spread by respiratory droplets, are the most common cause of pneumonia. Pneumonias are often distinguished as either community acquired or hospital acquired (nosocomial) depending on where the disease was contracted. Nosocomial pneumonias, particularly in the immunosuppressed individual, tend to be more severe and lead to a less favorable prognosis than community-acquired pneumonias. Typical community-acquired pneumonia is most commonly caused by Streptococcus pneumoniae, Haemophilus influenzae, and Staphylococcus species. Atypical forms of community-acquired pneumonia are caused by Mycoplasma pneumoniae, Legionella, and Chlamydia species. The influenza virus is the most common viral pathogen known to cause pneumonia. Nosocomial pneumonia is most commonly caused by infection with Pseudomonas aeruginosa or Staphylococcus aureus. Infection with an opportunistic fungal infection in the lungs, such as with Candida albicans or Pneumocystis jirovecii (formerly known as Pneumocystis carinii), indicates immunosuppression or immunodeficiency. Pneumonia can also occur when inhaling items not intended for the lungs, such as foods, fluids, and stomach contents, a condition called aspiration pneumonia. In many cases, the defense mechanisms in the nasopharynx and oropharynx of the upper respiratory tract effectively trap and expel microorganisms before causing infection. For pathogens that happen to escape the cough reflex and mucociliary blanket, macrophages located in the alveoli are often adequate to engulf and destroy the offending microorganism. When these defense mechanisms are inadequate, the inflammatory and immune responses are triggered, particularly in the interstitial lung tissue and alveoli. The inflamed alveoli fill with exudate. Other products of inflammation (red blood cells \[RBCs\], white blood cells \[WBCs\], and fibrin) accumulate as well and cause consolidation, or a solid mass in the lung tissue. These areas of consolidation are evident on radiographs and are often the key diagnostic feature of typical pneumonia (Fig. 15.6). Figure 15.6. Chest radiograph in a 50-year-old patient with pneumonia shows opacity characteristic of lung consolidation. (Reprinted with permission from Crapo, J. D., Glassroth, J., Karlinsky, J. B., & King, T. E. (2004). Baum's textbook of pulmonary diseases (7th ed.). Lippincott Williams & Wilkins.) With typical pneumonia, the presence of another viral infection, such as influenza, promotes attachment of the pneumococcal bacteria to the receptors on the respiratory epithelium. The bacteria can then infect the type II alveolar cells. The pneumococci multiply along the alveolus and penetrate the alveolar epithelium, thereby moving across to infect adjacent alveoli. In contrast, atypical pneumonia causes damage often through immune-mediated mechanisms rather than direct damage caused by the bacteria. The spread of infection with atypical pneumonia is more likely to spread beyond the lobar boundaries and is often bilateral. Histologic changes in the lungs during the progression of pneumonia can be categorized in three stages: 1\. Recent infection shows rapid filling of the alveolar capillaries with a frothy, serous, and blood-tinged fluid. 2\. A "red hepatization" stage follows and is marked by the filling of alveoli with fibrinous exudates, which appear as areas of dry, granular, dark-red lung tissue. Hepatization refers to the conversion of lung tissue into tissue (oftentimes solid) that resembles liver tissue. 3\. Within 72 hours, the "gray hepatization" stage occurs and is marked by WBCs packing into the alveoli as RBCs and epithelial cells degenerate. The pneumococcal bacteria release toxins that contribute to cell death. Eventually, the bacteria are opsonized by WBCs, a yellow exudate forms, these exudates are then absorbed, and resolution begins. The general effects of altered ventilation and diffusion apply to pneumonia: Oxygen diffusion is greatly impaired, hypoxia sets in, metabolic acidosis occurs, and dehydration may result. Dehydration is related to fluid losses through hyperventilation and fever, and it is exacerbated by inadequate fluid intake. Although most cases of pneumonia resolve within 2 weeks with appropriate treatment, individuals with preexisting respiratory disease are more likely to experience a deterioration of respiratory status, leading to respiratory failure and death. Respiratory failure can result from any problem that severely affects ventilation, ventilation--perfusion matching, or diffusion. It can occur quickly or insidiously (gradually with harmful effects). Box 15.2 outlines major causes of respiratory failure and relevant examples. Respiratory failure represents the failure of the lungs to adequately oxygenate the cells of the body and remove carbon dioxide. It is a life-threatening emergency. Respiratory failure can lead to a state of anoxia, a total lack of oxygenation. The cells quickly become hypoxic and the blood can become acidotic. If not reversed, death can ensue within minutes. CLINICAL MANIFESTATIONS Clinical manifestations relevant to pneumonia include sudden onset of fever, chills, cough, sputum production, fatigue, loss of appetite, dyspnea, tachypnea, tachycardia, pleuritic pain, and adventitious breath sounds caused by fluid accumulation in the lungs (crackles). These manifestations are related to the inflammatory and infectious processes. In adults (particularly in elderly people), headaches and even confusion can occur. DIAGNOSTIC CRITERIA Diagnosis is based on a thorough patient history and physical examination, noting the characteristic clinical manifestations. A complete blood count is performed to determine an elevation in the WBC count, which suggests bacterial infection. A chest radiograph or possibly a thoracic CT scan is also needed to identify areas of consolidation and to rule out other diseases or complications that may present with similar symptoms, such as bronchiectasis (irreversible dilation and destruction of the bronchial tree most often caused by chronic obstruction or infection), lung tumors, or heart failure. Identifying the causative microorganism is important for directing the treatment and for predicting the severity of disease and prognosis. This is completed through a Gram stain and culture and sensitivity tests of expectorated sputum. In addition, certain characteristics of sputum may suggest a specific pathogen. For example, individuals with pneumococcal pneumonia often present with bloody or rust-colored, dark sputum. Individuals with infections caused by Haemophilus or Pseudomonas are likely to expectorate green sputum. Anaerobic infections are typically foul smelling. Pleural fluid may also be aspirated via thoracentesis and tested if there is a pleural effusion (fluid in the pleural space) or empyema (lung abscess). Monitoring the ventilation and perfusion status of the individual with pneumonia requires measurement of oxygenation through the use of pulse oximetry and arterial blood gases. A nuclear (V/Q) lung scan may also be necessary to determine ventilation and perfusion efficacy. TREATMENT The goal of treatment for pneumonia is to restore optimal ventilation and diffusion. The plan of care, particularly the location of treatment (hospital or home) and the appropriate antibiotic, depends on the type of pneumonia (community or hospital acquired), the severity of disease, the presence of comorbid conditions, and the type of pathogen. For example, the initial antibiotic to treat community-acquired pneumonias in low-risk patients is a macrolide, such as azithromycin. Macrolides help to eradicate Gram-positive microorganisms, along with Mycoplasma and Legionella species. Community-acquired pneumonias in high-risk individuals (those older than 60 or with a comorbid condition) would be prescribed a macrolide along with an antibiotic that provides coverage of Gram-negative bacteria. Severe pneumonia requires hospitalization to provide adequate oxygen therapy, intravenous antibiotics (if bacterial), and intravenous fluids to prevent or reverse dehydration. Chest physiotherapy, deep breathing, and coughing may be needed to help loosen secretions and promote sputum expectoration. Treatment may also involve fever management and comfort measures. Chronic Obstructive Pulmonary Disease Chronic obstructive pulmonary disease (COPD) is a generic term that describes all chronic obstructive lung problems, including asthma, emphysema, and chronic bronchitis, separately or in combination. COPD is one of the leading causes of death worldwide. COPD is primarily used to denote the presence of both emphysema and chronic bronchitis (and, to some extent, asthma). Inflammatory processes in both the alveoli and in the bronchi/bronchioles characterize COPD. The disease is progressive, unremitting, and irreversible, although progression can be slowed if treatment is implemented early in the course of the disease. Although smoking has been implicated in the development of COPD, approximately 10% to 20% of those affected have never smoked. Because of significant lung reserves, symptoms may only become apparent when lung function is at or below 50%. In this section, emphysema, chronic bronchitis, and asthma are discussed separately. Emphysema Emphysema is an irreversible enlargement of the air spaces beyond the terminal bronchioles, most notably in the alveoli, resulting in destruction of the alveolar walls and obstruction of airflow. The most notable cause of emphysema is chronic smoking, although in nonsmokers, development of emphysema is often due to the genetically inherited deficiency of alpha-1 antitrypsin (AAT). Less commonly, pulmonary vascular damage from the insoluble fillers (e.g., cornstarch or cotton fibers) found in intravenous drugs (such as methadone or cocaine), immune deficiency syndromes, or connective tissue disorders (such as Marfan syndrome) can lead to emphysema development. PATHOPHYSIOLOGY In early or mild emphysema, the primary source of obstruction is the development of inflammation in the small airways distal to the respiratory bronchioles. If diagnosed early, the inflammation in the small airways can be reduced, thereby providing some relief from airway obstruction. In moderate-to-severe disease, the loss of elastic recoil in the alveoli is the primary mechanism of airflow obstruction. This is not reversible. Vascular changes in the lungs develop simultaneously along with the airway obstruction. The inner lining of the arteries and arterioles that perfuse the lungs becomes thick and fibrotic. This further contributes to alveolar and capillary destruction. Patterns of alveolar destruction can occur in the respiratory bronchioles and spread peripherally, termed centriacinar, or uniformly destroy the entire alveolus, termed panacinar. Centriacinar emphysema is typically associated with chronic smoking and primarily affects the upper half of the lungs, whereas panacinar emphysema occurs in those with AAT deficiency Chronic smoking impairs alveolar function through the following cascade of events: 1\. Inhalation of smoke triggers the inflammatory response. 2\. Neutrophils and later macrophages are activated and retained in the lung tissue. 3\. Neutrophils and macrophages release proteolytic enzymes, such as proteinases and elastases, that destroy components of the extracellular matrix, such as elastin, in the lungs. 4\. The elasticity of the lung is significantly reduced leading to the inability of the alveoli to recoil and release CO2 into the atmosphere. Elasticity is essential in the alveoli. Loss of elasticity affects the ability of the alveoli to contract and move air back out of the body. The air spaces become enlarged and ineffective. If the alveolar walls collapse, air remains trapped in the alveoli (Fig. 15.8). Air trapping decreases effective O2 intake and especially CO2 release. The experience of air trapping is demonstrated through taking a full breath and releasing half of the air and then again taking a full deep breath and releasing half of the air. When a person takes a deep breath and releases only half of the air, hyperinflation occurs in the lungs. The alveoli become further stretched and continue to lose elasticity. CO2 is retained and blood pH is reduced The lungs require protection against the destruction imposed by proteolytic enzymes. This is the primary role of antiprotease enzymes, particularly alpha-1 antitrypsin, an enzyme that is synthesized by cells in the lungs. This enzyme works to combat the destructive enzymes from digesting structural proteins and helps to maintain the integrity of the lungs. With emphysema, an imbalance of the destructive (proteolytic) and protective (antiproteolytic) enzymes exists, favoring the destructive. In smokers, lung destruction is a manifestation of excess proteinase release and a loss of antiproteinase defenses within the lungs. The development of emphysema in nonsmokers is most often related to a genetic disorder attributed to a reduction or absence of antiprotease enzymes. In AAT deficiency, the affected individual has inherited an autosomal-codominant disorder located on the long arm of chromosome 14. In codominant disorders, the phenotype is expressed in the individual who has inherited two equally dominant genes. This genetic defect causes an acceleration of the breakdown of cells and tissues in the lungs with the early onset of panacinar emphysema. CLINICAL MANIFESTATIONS Clinical manifestations are related to obstruction of the small airways and alveoli, chronic hypoxemia, and hypercapnia. Most patients with emphysema have smoked heavily for 20 or more years and present with a chronic productive cough, most notable upon waking in the morning. Dyspnea and wheezing may occur with minimal exertion. As the disease progresses, a barrel chest develops because of chronic hyperinflation of the airways. Patients with emphysema commonly perform pursed lip breathing to increase the pressure in the airways, to prevent alveolar wall collapse, and to allow the air to escape the alveoli. In severe disease, simple activities, such as brushing one's teeth, can cause tachypnea and respiratory distress. DIAGNOSTIC CRITERIA Diagnosis of emphysema is based on a thorough patient history and physical examination, noting significant smoking history and characteristic clinical manifestations. Expiratory airflow should be measured using pulmonary function tests. In nonsmokers who have suspected emphysema, AAT levels can be measured and will be found to be well below the expected range. In general, individuals with AAT deficiency and an increased risk for the development of emphysema will have 10% to 15% of the expected AAT level. Physical examination includes auscultation of lung sounds and observation of expiratory effort with simple activities. The respiratory rate tends to increase in proportion to disease severity. Cyanosis and peripheral edema may also be observed. Chest radiograph will show signs of hyperinflation. Pulmonary function tests are critical in the diagnosis of emphysema and the level of airflow obstruction. Spirometry determines disease progression and the response to treatment. FEV1 is the most common test to determine airflow obstruction; a prolonged forced expiratory time (FET) greater than 6 seconds indicates severe disease. Hypercapnia, measured in the arterial blood gas panel, is commonly observed when the FEV1 falls below 30% of the predicted volume. For example, if a patient (based on age, sex, and height) is expected to forcefully exhale 4 L of air per second, hypercapnia will present when forced exhalation expels less than 1.2 L of air per second. This indicates severe outflow obstruction. Hypoxemia is often present in early disease and continues throughout the disease progression; however, hypercapnia predominates as the disease progresses. TREATMENT Because emphysema is irreversible, the goals of treatment are to maintain optimal lung function in order to allow the individual to perform the desired activities of daily living. Treatment begins with smoking cessation and may also include drug therapy (with danazol or tamoxifen) to increase production of AAT by the liver or by administering exogenous AAT via intravenous infusion or inhalation. Bronchodilators, steroid anti-inflammatory drugs, mucolytic agents (to reduce thickness and promote clearance of sputum), and antibiotics are the mainstays of therapy for individuals with emphysema. Supplemental oxygen is often required. Lung volume reduction or lung transplant is a possible surgical treatment. Chronic Bronchitis Chronic bronchitis is defined by the presence of a persistent, productive cough with excessive mucus production that lasts for 3 months or longer for two or more consecutive years. The chronic bronchitis label is applied only after all other potential causes of chronic cough are excluded. The cause of chronic bronchitis is most commonly chronic smoking or exposure to environmental pollutants that irritate the airways. PATHOPHYSIOLOGY Chronic bronchitis results from several changes in the bronchi and bronchioles of the lungs in response to chronic injury including: Chronic inflammation and edema of the airways Hyperplasia of the bronchial mucous glands and smooth muscles Destruction of cilia Squamous cell metaplasia Bronchial wall thickening and development of fibrosis The chronic inflammatory process, metaplasia, fibrosis, and mucous gland hyperplasia cause airway deformities and obstruct airflow by narrowing the airway lumen and further occluding this narrowed lumen through overproducing mucus (Fig. 15.9). This is particularly problematic in the smaller airways and accounts for most airway obstructions. The loss of ciliated epithelium allows fine particles to enter the airway easily and predispose the individual to infection. LINICAL MANIFESTATIONS The clinical manifestations of chronic bronchitis are often similar to those of emphysema because the two conditions often occur simultaneously. A chronic productive cough, often with purulent sputum, is frequent. Dyspnea occurs with minimal exertion. Chronic bronchitis leads to a prolonged expiratory phase along with wheezing and crackles upon auscultation of the lungs. Hypoxemia, hypercapnia, and cyanosis are more commonly found in those with chronic bronchitis compared with individuals with emphysema because of excessive bronchial mucus and obstructed ventilation. DIAGNOSTIC CRITERIA The diagnosis of chronic bronchitis is based on the clinical presentation of a persistent, productive cough over a period of 3 months or more within two consecutive years. A history of smoking is often present along with recurrent upper and lower respiratory infections. Arterial blood gases may be significant for hypoxemia and hypercapnia. Laboratory tests may indicate polycythemia (the overproduction of RBCs) as a compensatory measure to combat chronic hypoxemia. As with emphysema, pulmonary function tests are performed and demonstrate a reduced FEV1 and prolonged FET. Sputum specimens are often examined and tested for the presence of pathogens. TREATMENT As with emphysema, treatment of chronic bronchitis is aimed at alleviating symptoms, improving airway and lung function, slowing the progression of the disease, and improving overall quality of life. Treatment strategies are most effective when implemented early in the course of the disease. Smoking cessation is a critical component of success, as are pulmonary rehabilitation, bronchodilator therapy, steroid anti-inflammatory drugs, mucolytic agents, supplemental oxygen when indicated, and antibiotic therapy and immunizations to treat and protect against infection. Asthma Asthma is a chronic inflammatory disorder of the airways that results in intermittent or persistent airway obstruction because of bronchial hyperresponsiveness, inflammation, bronchoconstriction, and excess mucus production. The development of asthma often occurs in childhood, but the condition can emerge at any point in the life span. Individuals most likely to develop asthma include those considered atopic or having a genetic predisposition to developing hypersensitivities. PATHOPHYSIOLOGY Although the exact cause is unknown, asthma is increased in individuals who are frequently exposed to environmental allergens, such as cigarette smoke or dust mites. This discussion of asthma combines what is known about hypersensitivity reactions and the inflammatory process, because both of these concepts play a major role. The inflammatory and immune response is often stimulated through exposure to an allergen. This allergen varies based on the individual and is often referred to as the asthma "trigger." Common environmental exposures that are known to result in hypersensitivity reactions and trigger the inflammatory response include smoke, dust, dust mites, mold, or animal hair. Other common triggers that can result in bronchospasm include exercise, temperature extremes, illness, and anxiety. Exercise-induced asthma (EIA) is a condition in which exercise or vigorous physical activity triggers acute bronchospasm, coughing, and wheezing in susceptible persons. After an individual is exposed to the trigger, an IgE-mediated hypersensitivity reaction is immediate (Fig. 15.10A). IgE mast cells are stimulated to release chemical mediators. These chemical mediators promote increased edema and subsequent bronchoconstriction in the airways. Further products of inflammation then move into the area. Mast cells call forth additional chemical mediators, such as histamine and prostaglandins. Hours later, leukotrienes are released. These chemical mediators stimulate further bronchospasm, swelling, and excessive mucus production in the airways (Fig. 15.10B). The late inflammatory response occurs approximately 6 to 24 hours after exposure to the trigger and is marked by airway edema and the formation of mucus plugs from exudate and cell debris in the airways. The mucus plugs can take weeks to resolve. Over time, the cells of chronic inflammation, along with eosinophils, infiltrate the airways and cause destruction of the respiratory epithelium, smooth muscle hyperplasia, and narrowing of the airways. These structural changes, called airway remodeling, strongly affect the irreversibility of the condition. CLINICAL MANIFESTATIONS Clinical manifestations depend on the state of airway hyperreactivity and inflammation. In periods of remission, the individual is symptom free. As an exacerbation is emerging, the individual may not notice any symptoms, although pulmonary function tests begin to decline. In times of exacerbation, hyperreactivity and inflammation in the airways causes wheezing, breathlessness, chest tightness, excessive sputum production, and coughing, particularly at night or in the early morning. During an asthma episode, the individual may exhibit anxiety, tachypnea, and the use of accessory muscles. Hyperventilation initially leads to respiratory alkalosis; however, compensation is usually temporary, and acidosis develops because of ineffective expiration. Even with a partial airway obstruction, hypoxia quickly results. Nath4e-Hand-icon Stop and Consider Based on the basic pathophysiologic processes, construct a treatment plan for a person with asthma. What environmental modifications would you make? What would be the goals of pharmacologic therapy? DIAGNOSTIC CRITERIA The patient may request an evaluation for asthma during a time of remission or may present to the health professional in acute distress. The diagnosis includes evaluating signs and symptoms and determining the triggers to determine the likelihood that the manifestations are actually attributable to asthma. The patient's history is often significant for atopy or asthma in the family. The patient may or may not be currently experiencing the characteristic clinical manifestations. Physical findings indicative of asthma include: Evidence of respiratory distress Pulsus paradoxus, an exaggerated decrease in systolic blood pressure during inspiration Wheezing breath sounds A prolonged expiratory phase Atopic dermatitis, eczema, or other allergic skin conditions that may indicate hypersensitivities Laboratory findings may reveal eosinophilia (indicating allergy) and arterial blood gases indicative of hypoxemia and hypercapnia. Pulse oximetry is useful in screening for hypoxemia and may be used to grade asthma severity. Spirometry is used to determine the effectiveness of airflow. The peak expiratory flow rate (PEFR) is a common measure used to track forced expiration. The peak flow meter is a convenient, portable, inexpensive tool. Measurements over time can provide information about the patient's response to therapy and can indicate the emergence of an asthma exacerbation. Chest radiograph may demonstrate hyperinflation or infiltrates of the lung fields and can be used to rule out other sources of pleuritic chest pain, such as pneumonia or pneumothorax (the presence of air in the pleural space that causes the lung to collapse). Once diagnosed, asthma can then be classified based on severity, frequency, and duration of disease. The classification guide and treatment measures are found in Box 15.3. BOX 15.3 Asthma Classification and Treatment Based on Severity Mild intermittent Intermittent symptoms occurring less than once a week with brief exacerbations Nocturnal (nighttime) symptoms occurring less than twice a month Asymptomatic with normal lung function between exacerbations No daily medication needed FEV1 or PEFR greater than 80%, with less than 20% variability Mild persistent Symptoms occurring more than once a week but less than once a day Exacerbations affecting activity and sleep Nocturnal symptoms occurring more than twice a month Low-dose inhaled anti-inflammatory one to four times per day and inhaled bronchodilator as needed FEV1 or PEFR greater than 80% predicted, with variability of 20% to 30% Moderate persistent Daily symptoms Exacerbations affecting activity and sleep Nocturnal symptoms occurring more than once a week Medium-dose inhaled anti-inflammatory one to four times per day, a long-acting bronchodilator, especially for nighttime symptoms, and short-acting bronchodilator as needed FEV1 or PEFR 60% to 80% of predicted, with variability greater than 30% Severe persistent Continuous symptoms with frequent exacerbations, nocturnal asthma symptoms Physical activities limited by asthma symptoms High-dose inhaled and oral anti-inflammatory, a long-acting bronchodilator, and short-acting bronchodilator as needed FEV1 or PEFR less than 60%, with variability greater than 30% TREATMENT The treatment of asthma has four major components: 1\. Monitoring lung function through peak flow testing 2\. Controlling environmental triggers 3\. Pharmacologic therapy to reverse inflammation, bronchoconstriction, and mucus secretion 4\. Patient education to facilitate adherence to the treatment plan Alternative and complementary therapies have been implemented as possible treatments for conditions of altered ventilation and diffusion. One group of researchers explored the effectiveness of acupressure in promoting relaxation, decreasing anxiety, and alleviating dyspnea in patients with COPD. In this randomized clinical trial, patients were either provided true acupoint acupressure or a sham treatment. Those patients undergoing true acupoint acupressure demonstrated significant improvements in pulmonary function, dyspnea scores, 6-minute walking distance measurements, and state anxiety scale scores. A written action plan is an essential aspect of care for the individual with asthma. The action plan outlines methods for avoiding triggers and describes what to do when an asthma exacerbation is occurring. The plan is based on the measurement of the PEFR using a peak flow meter. The individual determines their highest PEFR, or "personal best," over a symptom-free period of 1 to 3 weeks. The inability to expire at or above 80% of the personal best requires attention because an asthma exacerbation may be occurring. Pharmacologic treatments are prescribed based on the classification of asthma severity. Medications used to treat asthma are divided into two major categories: bronchodilators and anti-inflammatory drugs. Inhaled beta2-adrenergic agonists (bronchodilators), such as albuterol, provide quick relief of bronchoconstriction and can prevent exercise-induced asthma. Anticholinergics and methylxanthines are other drug categories used to promote bronchodilation. These medications relax the smooth muscles of the airway but do not effectively reduce airway inflammation. Long-term control medications, such as glucocorticoids, cromolyn, and leukotriene modifiers, are needed to prevent the frequency and severity of the inflammatory component of asthma. These medications also prevent chronic damage to the airways. For example, inhaled glucocorticoids suppress the activation of mast cells and other components active in inflammation and decrease airway hyperreactivity. Asthma, although most often reversible, can lead to total airway obstruction if the inflammation is severe. In status asthmaticus, or intractable asthma, bronchospasm is not reversed by the patient's medications or other measures. Obstruction leads to decreased expiration, air trapping, hypoxemia, hypercapnia, and acidosis. Absent lung sounds and significant elevations in the PaCO2 (above 70 mmHg) indicate a poor prognosis. Status asthmaticus is life-threatening and requires immediate emergency treatment. Cystic Fibrosis Cystic fibrosis (CF) is an autosomal recessive disorder of electrolytes and subsequently water transport that affects certain epithelial cells, such as those lining respiratory, digestive, and reproductive tracts. Cystic fibrosis most commonly affects people of European descent and is the most common lethal inherited disease in that group. Incidence appears greatest in homogenous populations. This disorder leads to the production of excessive and thick exocrine secretions (e.g., mucus) leading to obstruction, inflammation, and infection. CF is also associated with impaired local immune defenses in the lungs as well as pancreatic insufficiency. Most affected individuals are diagnosed by 1 year of age. A small percentage of individuals exhibit a mild presentation and are not diagnosed until after 10 years of age. End-stage lung disease is the most common cause of death. PATHOPHYSIOLOGY Cystic fibrosis is caused by a mutation of the CF The condition results when the cystic fibrosis transmembrane conductance regulator (CFTR) gene causes the CFTR protein to become dysfunctional. gene located on the long arm of chromosome 7. Because CF is an autosomal recessive disease, a deleterious mutation is required on both inherited CFTR alleles for development of the disease. More than 1,000 possible CFTR gene mutations, if found on both alleles in any combination, have been identified. Consistent with autosomal recessive inheritance, those with one mutated allele and one nonmutated allele are considered carriers and have no symptoms of the disease. The CFTR gene mutation leads to impaired electrolyte transportation across epithelial cells on mucosal surfaces (Fig. 15.11). For example, the effective functioning of chloride ion channels depends on the CFTR gene, which encodes for a protein that serves as a chloride channel and is regulated by cyclic adenosine monophosphate (cAMP). Mutations in the CFTR gene lead to impairment in the cAMP-regulated chloride transport across many different types of epithelial cells on mucosal surfaces, such as those found lining the respiratory tract, pancreas, bile ducts, sweat ducts, and vas deferens. The inability of these epithelial cells to conduct chloride and therefore transport water across the mucosal surfaces leads to thick secretions and obstruction in the respiratory tract, pancreas, gastrointestinal tract, sweat glands, and other exocrine tissues. Although the manifestations appear mostly related to impaired transport of electrolytes, the complete mechanism of disease, particularly those leading to the complications associated with the lungs, is unknown.CF is most strongly associated with mucus plugging, inflammation, and infection in the lungs with respiratory failure as the most common cause of death. Mucus plugging is a result of: A greater volume of mucus produced by a larger number of mucus-secreting cells in the airways Airway dehydration and thickened mucus caused by impaired chloride secretion, excessive sodium absorption, and decreased water content in the airway tissues Adherence of the mucus to the epithelium with impaired ciliary action required to clear the mucus from the airways Mucus plugging, the formation of tenacious secretions, and a reduced ability to clear the secretions are optimal conditions for the growth and retention of bacteria. Progressive lung disease often begins shortly after birth with the emergence of a persistent respiratory infection. Impairment of local immune function leads to failure of opsonization and phagocytosis and contributes to this persistent lung infection with distinctive bacteria, such as Staphylococcus aureus and Pseudomonas aeruginosa. The progression from bronchitis to bronchiolitis, to bronchiectasis, and eventually to permanent lung damage largely depends on an intense, chronic inflammatory response dominated by neutrophils. The significance of the excessive presence of neutrophils is the release of proteases by these cells. Proteases promote an intense inflammatory response, cause destruction of lung tissue, inhibit destruction or neutralization of bacteria, and promote additional mucus production. Over time, the airways and lung tissue in CF are characterized by air trapping, hyperinflation, abscess formation, lung tissue consolidation, persistent pneumonia, lung tissue fibrosis, and cyst formation (notably in the pancreas), giving the condition its name. Erosion of lung tissues and nearby bronchial arteries can lead to hemoptysis. Eventually, pulmonary involvement can lead to right-sided heart failure (cor pulmonale) and end-stage lung disease. CF also has effects that go beyond ventilation and diffusion. Reduced chloride secretion and restricted water into the intestinal tract (along with inflammation, scarring, and strictures) lead to obstruction of intestinal contents. Along with intestinal motility problems, most individuals with CF (90% to 95%) have pancreatic insufficiency. Pancreatic insufficiency decreases digestion and absorption of intestinal contents. The role of pancreatic enzymes, digestion, and absorption is described in detail in Chapter 17. The liver may also be affected in CF because the mutation of the CFTR gene leads to impairment of passive transport of chloride and water across the epithelial cells that line the biliary ductules. This leads to increased bile viscosity. Biliary obstruction can lead to obstructive cirrhosis. CLINICAL MANIFESTATIONS The presenting clinical manifestations, age at diagnosis, symptom severity, and rate of disease progression vary widely among individuals with CF. Clinical manifestations are associated with tenacious (thick) secretions leading to respiratory and gastrointestinal impairment. Recurrent respiratory infections, along with a chronic cough often accompanied by mucoid or purulent sputum, are common manifestations of CF. Coughing can be forceful and can lead to vomiting. Other respiratory manifestations include tachypnea, recurrent wheezing or crackles, hemoptysis, dyspnea on exertion, chest pain, and respiratory distress with chest retractions, cyanosis, barrel chest, recurrent sinusitis, and the development of nasal polyps. Finger clubbing can also be caused by chronic hypoxia. Newborns may present with intestinal obstruction (meconium ileus) at birth with delayed or absent passage of meconium stool. Infants and children most commonly demonstrate increased frequency of large, greasy, malodorous stools, which indicate fat malabsorption. This condition can be accompanied by recurrent abdominal pain, distention, and poor absorption of fat-soluble vitamins. Weight loss or poor weight gain is common. Additional clinical manifestations include sweat abnormalities and excessive salt depletion. A child, when kissed by a caregiver, may have a salty taste to the skin. Jaundice, gastrointestinal bleeding, and rectal prolapse may occur. Males with CF are frequently sterile because of a congenital bilateral absence of the vas deferens, preventing the sperm from ever becoming part of the semen and exiting the penis. The male child may also have undescended testicles or hydrocele. Secondary sexual development is often delayed in females with CF, although fertility is maintained or somewhat decreased. DIAGNOSTIC CRITERIA The diagnosis of CF is based on a thorough patient history and physical examination, noting the characteristic clinical manifestations. The diagnosis can be confirmed by a sweat test, which will reveal a sweat chloride concentration of 60 mEq/L or greater. Values of 40 to 60 mEq/L are considered borderline, and the test must be repeated because values in this range can be inconsistent with the diagnosis in some patients with typical features. Genetic testing may be performed to detect certain CFTR mutations. The identification of two CFTR mutations with associated clinical symptoms is diagnostic; however, negative results on genotype analysis do not exclude the diagnosis. The ability to sequence the entire gene will enable clinicians to detect all known mutations in any given individual and will have greater reliability in diagnosing CF. Other diagnostic tests, such as chest and sinus radiography and sputum analysis, can also contribute to the diagnosis. Chest radiography will reveal hyperinflation and peribronchial thickening. Sinusitis throughout all sinuses is uncommon in children and young adults, and its presence strongly suggests CF. The fluid obtained from a bronchoalveolar lavage usually shows a high percentage of neutrophils and the detection of Pseudomonas aeruginosa supports the diagnosis of CF in an individual without the typical presentation. Because early detection and treatment is critical in the course and prognosis of disease, newborn screening has been recommended in many developed countries. TREATMENT Treatment goals for CF involve maximizing ventilation, diffusion, and nutrition through: Liquefying and clearing the airways of mucus Avoiding and controlling respiratory infections Reducing inflammation and promoting bronchodilation in the airways Providing or encouraging optimal nutrition through the use of enzyme supplements to reduce malabsorption, multivitamin and mineral supplements, and a high-calorie diet Managing disease complications, such as diabetes mellitus, bowel obstruction, fatty liver, biliary cirrhosis, and portal hypertension Individuals with end-stage lung disease may consider lung transplantation. Currently, the median age of survival is 46 years; males survive significantly longer than females, although many individuals with CF are living into their 50s. Acute Respiratory Distress Syndrome Acute respiratory distress syndrome (ARDS) is a condition of severe acute inflammation and pulmonary edema without evidence of fluid overload or impaired cardiac function (Fig. 15.12). This condition was previously referred to as adult respiratory distress syndrome, but ARDS does occur in children PATHOPHYSIOLOGY Damage to the alveolar epithelium and vascular endothelium triggers the onset of severe inflammation. Injury can result from inhalation of excessive smoke or toxic chemicals, overwhelming lung infections, aspiration of gastric contents into the lungs, lung trauma, anaphylaxis, lack of pulmonary blood flow, and other conditions that impair the alveoli. The presence of sepsis (bacterial infection in the blood) and the systemic inflammatory response syndrome (SIRS) are clinical conditions associated with the development of ARDS. In ARDS, the time from lung injury to respiratory distress is about 24 to 48 hours. During the inflammatory response, chemical mediators promote vasodilation and increased capillary permeability in the lungs. Inflammatory cells, proteins, and fluids escape the intravascular space and leak into the interstitium (lung tissues) and alveolar space, resulting in pulmonary edema, which damages the alveolar--capillary junction. Damage to type II alveolar cells, increased protein, and fluid accumulation disrupts the production of surfactant resulting in atelectasis (alveolar nonaeration and collapse). Other potential causes of atelectasis are summarized in Box 15.4. Initially, oxygen diffusion is greatly impaired, but CO2 is still able to cross the alveolar--capillary junction to be expired. As the process of alveolar impairment advances, a hyaline membrane forms, and CO2 release is also interrupted. The hyaline membrane is a thin, clear basement membrane that is impervious to gases. This leads to a state of impaired ventilation and diffusion marked by poor lung expansion, hypoxemia, hypercapnia, and acidosis. BOX 15.4 Potential Causes of Atelectasis Atelectasis, a condition of collapse and nonaeration of the alveoli, can have a number of causes, including: Compression of the alveoli by a mass or fluid accumulation, such as with a tumor or pleural effusion, which exerts pressure on the lung and prevents air from entering the alveoli. Obstruction, which prevents air from entering the airways and alveoli; existing air is reabsorbed into the tissues and the alveoli become empty. Destruction of surfactant, as with the inflammatory response, increases surface tension in the alveoli and promotes collapse. Fibrosis, such as with emphysema, restricts alveolar expansion and promotes collapse. ARDS can be reversed if the symptoms are identified early. Healing involves reabsorption of the alveolar edema, regeneration of the epithelial cells and type II alveolar cells, and structural remodeling of the airway. In some cases, the patient has an uncomplicated acute inflammatory response with rapid resolution. Other patients experience a permanent loss of lung volume and function attributed to lung tissue edema, necrosis, and alveolar fibrosis in affected areas. If untreated, death can ensue within 48 hours. The mortality rate, as a result of multisystem organ failure, is approximately 30% to 40%. CLINICAL MANIFESTATIONS Early clinical manifestations include tachypnea, dyspnea, retractions, crackles caused by fluid accumulation, and restlessness. Some patients may present in the course of disease without any signs or symptoms except for mild tachypnea. Within 48 hours, severe respiratory distress becomes apparent. DIAGNOSTIC CRITERIA The diagnosis of ARDS is based on a careful patient history, physical examination, laboratory, and imaging studies. A high index of suspicion is required when encountering any individual who has lung injury. Arterial blood gases often depict hypoxemia with early respiratory alkalosis quickly progressing to hypercapnia and respiratory alkalosis. Blood cultures may be needed to detect sepsis, the most common cause of ARDS. Chest radiograph is often normal early in the course of disease but then reveals bilateral diffuse infiltrates advancing to total opacity as the disease progresses. Arterial blood gas analyses measure arterial blood and determine levels of pH, PaO2, and PaCO2. In respiratory failure, pH falls below 7.3, PaO2 falls below 50 mmHg (the expected range is 80 to 100 mmHg), and PaCO2 rises above 50 mmHg (the expected range is 35 to 45 mmHg). TREATMENT Treatment strategies are supportive and focused on removing the causative factors triggering the inflammatory response. Administration of 100% oxygen is often warranted to keep oxygen saturations above 90%. If the oxygen saturations remain below this level and the patient becomes fatigued or acidotic, intubation with mechanical ventilation may be necessary. If mechanical ventilation is ineffective in maintaining oxygen saturations above 90%, positive end-expiratory pressure (PEEP) is instituted to maintain pressure in the airways during expiration and promote reinflation of alveoli. In patients with ARDS, maintenance of adequate alveolar ventilation is an important marker for survival. Summary The process of ventilation involves both acquiring oxygen (inspiration) and removing carbon dioxide (expiration) from the blood. The transport of oxygen through the lungs is the only mechanism for acquiring oxygen. Oxygen is necessary for cellular metabolism, which means that for the cell to expend energy efficiently and perform its designated function, oxygen must be present. Optimal cell functioning occurs within a narrow pH range, and the release and retention of carbon dioxide is one mechanism for maintaining this balance. Oxygen and carbon dioxide are exchanged at the alveolar--capillary junctions in the process of diffusion. Impaired ventilation is a problem of blocking airflow in and out of the lungs, thereby restricting oxygen intake and carbon dioxide removal from the body. Two major mechanisms are implicated: (1) compression or narrowing of the airways and (2) disruption of the neural transmissions needed to stimulate the mechanics of breathing. Impaired diffusion is a process of restricting the transfer of oxygen or carbon dioxide across the alveolar--capillary junction. Because the rate of diffusion depends on the solubility and partial pressure of the gas, and surface area and thickness of the membrane, impaired gas exchange can occur with changes in any of these properties. Any situation that presents a demand for higher levels of oxygen or an increase in cellular metabolism requires pulmonary adaptation to maintain homeostasis, because oxygen deprivation and carbon dioxide retention affect all cells in the body. The major implications of altered ventilation and diffusion include hypoxemia, hypoxia, hypercapnia, and acidosis. Local manifestations of impaired ventilation and diffusion include cough, mucus production, sputum changes, dyspnea, use of accessory muscles, adventitious breath sounds, chest pain, barrel chest, and pursed lip breathing. Systemic manifestations of altered ventilation are caused by the effects of inflammation, hypoxemia, hypoxia, and hypercapnia. These manifestations include fever, malaise, leukocytosis, cyanosis, changes in arterial blood gases, mental status changes, and finger clubbin