Gene Regulation in Bacteria PDF
Document Details
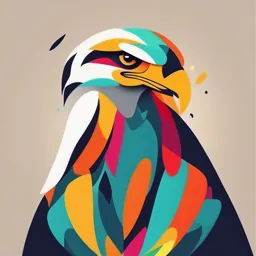
Uploaded by SimplerFoxglove
University of Minnesota
Robert J. Brooker
Tags
Summary
This chapter discusses gene regulation in bacteria, focusing on transcriptional control mechanisms. It details the role of regulatory proteins, such as repressors and activators, and the influence of small effector molecules on gene expression. The lac operon is presented as a key example of gene regulation.
Full Transcript
Because learning changes everything. ® Chapter 14 Gene Regulation in Bacteria Genetics: Analysis & Principles EIGHTH EDITION Robert J. Brooker © McGraw Hill LLC. All rights reserved. No reproduction or distribution without the prior written consent of McGraw Hill LLC....
Because learning changes everything. ® Chapter 14 Gene Regulation in Bacteria Genetics: Analysis & Principles EIGHTH EDITION Robert J. Brooker © McGraw Hill LLC. All rights reserved. No reproduction or distribution without the prior written consent of McGraw Hill LLC. Gene Regulation in Bacteria © McGraw Hill © SPL/Science Source 2 Introduction The term gene regulation means that the level of gene expression can vary under different conditions Genes that are unregulated are termed constitutive They have essentially constant levels of expression Frequently, constitutive genes code proteins that are continuously necessary for the survival of an organism The benefit of regulating genes is that coded proteins will be produced only when required © McGraw Hill 3 Introduction Gene regulation is important for cellular processes such as: 1. Metabolism 2. Response to environmental stress 3. Cell division Regulation can occur at any of the points on the pathway to gene expression Refer to Figure 14.1 © McGraw Hill 4 Common Points of Gene Regulation in Bacteria Fig 14.1 © McGraw Hill 5 14.1 Overview of Transcriptional Regulation The most common way to regulate gene expression in bacteria is by influencing the initiation of transcription The rate of RNA synthesis can be increased or decreased Transcriptional regulation involves the actions of proteins called regulatory transcription factors (R T Fs) Two types: Repressors Bind to DNA and inhibit transcription Activators Bind to DNA and increase transcription © McGraw Hill 6 14.1 Overview of Transcriptional Regulation Negative control refers to transcriptional regulation by repressor proteins Positive control refers to regulation by activator proteins © McGraw Hill 7 Small Effector Molecules Small effector molecules affect transcriptional regulation They bind to regulatory transcription factors but not to DNA directly A small effector molecule may increase transcription These molecules are termed inducers Bind to activators and cause them to bind to DNA Bind to repressors and prevent them from binding to DNA Genes that are regulated in this manner are termed inducible © McGraw Hill 8 Small Effector Molecules A small effector molecule may inhibit transcription Corepressors bind to repressors and cause them to bind to DNA Inhibitors bind to activators and prevent them from binding to DNA Genes that are regulated in this manner are termed repressible © McGraw Hill 9 In the absence of the inducer, this repressor protein blocks transcription. The presence of the inducer causes a conformational change that inhibits the ability of the repressor protein to bind to the DNA. Transcription proceeds. (a) Repressor protein, inducer molecule, inducible gene Fig 14.2a © McGraw Hill 10 This activator protein cannot bind to the DNA unless an inducer is present. When the inducer is bound to the activator protein, this enables the activator protein to bind to the DNA and activate transcription. (b) Activator protein, inducer molecule, inducible gene Fig 14.2b © McGraw Hill 11 In the absence of a corepressor, this repressor protein will not bind to the DNA. Therefore, transcription can occur. When the corepressor is bound to the repressor protein, a conformational change occurs that allows the repressor to bind to the DNA and inhibit transcription. (c) Repressor protein, corepressor molecule, repressible gene Fig 14.2c © McGraw Hill 12 This activator protein will bind to the DNA without the aid of an effector molecule. The presence of an inhibitor causes a conformational change that inhibits the ability of the activator protein to bind to the DNA. This inhibits transcription. (d) Activator protein, inhibitor molecule, repressible gene Fig 14.2d © McGraw Hill 13 14.2 Regulation of the lac Operon At the turn of the 20th century, scientists made the following observation A particular enzyme appears in the cell only after the cell has been exposed to the enzyme’s substrate This observation became known as enzyme adaptation François Jacob and Jacques Monod at the Pasteur Institute in Paris were interested in this phenomenon They focused their attention on lactose metabolism in E. coli © McGraw Hill 14 The lac Operon An operon is a regulatory unit consisting of a few protein- coding genes under the control of one promoter An operon encodes a polycistronic mRNA that contains the coding sequence for two or more protein-encoding genes This allows a bacterium to coordinately regulate a group of genes that code proteins with a common functional goal In E. coli, two transcriptional units are involved in lactose utilization: lac operon lacI gene © McGraw Hill 15 The lac Operon DNA elements that control transcription Promoter Binds RNA polymerase Operator Binds the lac repressor protein CAP site Binds the Catabolite Activator Protein (CAP) Terminator Ends transcription © McGraw Hill 16 The lac Operon Protein-coding genes lacZ Codes b-galactosidase Enzymatically cleaves lactose and lactose analogs Also converts lactose to allolactose lacY Codes lactose permease Membrane protein required for transport of lactose and analogs lacA Codes galactoside transacetylase Covalently modifies lactose and analogs Prevents toxic buildup of nonmetabolizable lactose analogs © McGraw Hill 17 (a) Organization of DNA sequences in the lac region of the E. coli chromosome Fig 14.3a © McGraw Hill 18 (b) Functions of lactose permease and β-galactosidase Fig 14.3b © McGraw Hill 19 The lac Operon is Regulated By a Repressor Protein The lac operon can be transcriptionally regulated 1. By a repressor protein 2. By an activator protein The first method is an inducible, negative control mechanism It involves lac repressor The inducer is allolactose Allolactose binds to lac repressor and prevents the repressor from binding to the DNA A process known as allosteric regulation © McGraw Hill 20 In the absence of the inducer allolactose, the repressor protein is tightly bound to the operator site, thereby inhibiting the ability of RNA polymerase to transcribe the operon. (a) No lactose in the environment Fig 14.4a © McGraw Hill 21 When allolactose is available, it binds to the repressor. This alters the conformation of the repressor protein, which prevents it from binding to the operator site. Therefore, RNA polymerase can transcribe the operon. (b) Lactose present Fig 14.4b © McGraw Hill 22 Fig 14.5 © McGraw Hill 23 Experiment 14A: The lacI Gene Codes a Diffusible Repressor Protein In the 1950s, Jacob and Monod, and their colleague Arthur Pardee, had identified a few rare mutant strains of bacteria with abnormal lactose adaptation One type of mutant involved a defect in the lacI gene It was designated lacI– It resulted in the constitutive expression of the lac operon even in the absence of lactose The lacI– mutations mapped very close to the lac operon © McGraw Hill 24 Experiment 14A Jacob, Monod, and Pardee used bacterial conjugation methods to introduce different portions of the lac operon into different strains They identified F’ factors (plasmids) that carried portions of the lac operon For example: Consider an F’ factor that carries the lacI gene Bacteria that receive this will have two copies of the lacI gene One on the chromosome and the other on the F’ factor These are called merozygotes, or partial diploids © McGraw Hill 25 Merozygotes are partial diploids Merozygotes were instrumental in allowing Jacob, Monod, and Pardee to elucidate the function of the lacI gene There are two key points 1. The two lacI genes in a merozygote may be different alleles lacI– on the chromosome lacI+ on the F’ factor 2. Genes on the F’ factor are not physically connected to those on the bacterial chromosome © McGraw Hill 26 The Hypothesis Jacob, Monod and Pardee hypothesized that the lacI– mutation results in the synthesis of an internal inducer If correct: The inducer protein produced from the chromosome can diffuse and activate the lac operon on the F’ factor Alternative hypothesis is that lacI– mutation eliminates the function of lac repressor that can diffuse throughout the cell If correct: The repressor coded on the F’ factor can diffuse and turn off the lac operon on the bacterial chromosome © McGraw Hill 27 (a) Correct explanation Fig 14.6a © McGraw Hill 28 (b) Internal activator hypothesis Fig 14.6b © McGraw Hill 29 Testing the Hypothesis 1. Grow mutant strain and merozygote strain separately. 2. Divide each strain into two tubes. 3. To one of the two tubes, add lactose. 4. Incubate the cells long enough to allow lac operon induction. 5. Lyse the cells with a sonicator. This allows β- galactosidase to escape from the cells. © McGraw Hill 30 Testing the Hypothesis 6. Add β-o-nitrophenylgalactoside (β-ONPG). This is a colorless compound. If β-galactosidase is present, it will cleave the compound to produce galactose and o- nitrophenol (O-NP). O-Nitrophenol has a yellow color. The deeper the yellow color, the more β-galactosidase was produced. 7. Incubate the sonicated cells to allow β-galactosidase time to cleave β-o-nitrophenylgalactoside. 8. Measure the yellow color produced with a spectrophotometer. (See the Appendix A for a description of spectrophotometry.) © McGraw Hill 31 Fig 14.7 © McGraw Hill 32 Fig 14.7 © McGraw Hill 33 The Data Addition of Amount of β-galactosidase Strain lactose (percentage of parent strain) Mutant No 100% Mutant Yes 100% Merozygote No