MiBi VL7 Regulation der Genexpression PDF
Document Details
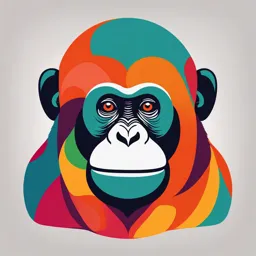
Uploaded by FeasibleJubilation
Freie Universität Berlin
Prof. Haike Antelmann
Tags
Summary
This document is lecture notes on bacterial gene expression regulation. It covers transcriptional, translational, and post-translational control. The document also includes diagrams describing the regulation of gene expression.
Full Transcript
Regulation der bakteriellen Genexpression Prof. Haike Antelmann Institut für Biologie - Mikrobiologie Regulations-Ebenen der bakteriellen Genexpression Transcriptional control Translational control Post-translational control http://www.discoveryandinnovation.com/BIOL2...
Regulation der bakteriellen Genexpression Prof. Haike Antelmann Institut für Biologie - Mikrobiologie Regulations-Ebenen der bakteriellen Genexpression Transcriptional control Translational control Post-translational control http://www.discoveryandinnovation.com/BIOL202/notes/lecture16.html RNA transcription by RNA polymerase Snyder, Champness – Molecular genetics of bacteria, ASM Press, 2003, 2007 Promoter organization upstream downstream Pribnow box Terminator UP-element TTGACA TATAAT 5‘ 3‘ AT rich spacer -40 to - 60 -35 -10 +1 (17±1nt) Open Reading Frame (ORF) Monocistronic operon Promoter TSS RBS ORF Terminator 5‘ 3‘ -35 -10 +1 Transcription elements Translation elements Promoter elements ORF – Open Reading Frame TSS: Transcription start site RBS – Ribosome binding site Terminator (Shine-Dalgarno-Sequence) Open Reading Frame (ORF) Polycistronic operon Promoter TSS RBS ORF RBS ORF RBS ORF Terminator 5‘ 3‘ -35 -10 +1 Transcription elements Translation elements Promoter elements ORF – Open Reading Frame TSS: Transcription start site RBS – Ribosome binding site Terminator (Shine-Dalgarno-Sequence) Transcription by the bacterial RNA-polymerase © David S. Goodsell Snyder, Champness – Molecular genetics of bacteria, ASM Press, 2003, 2007 Subunits of bacterial RNA-polymerase Sigma + Core RNA Pol => Holoenzyme Snyder, Champness – Molecular genetics of bacteria, ASM Press, 2003, 2007 Subunits of RNA-polymerase (E. coli) Subunit gene function α (36 kDa) rpoA dimer of α subunits, formed through their N-terminal domains (NTD) initiates the process of core assembly as follows: α2 + β + β´/ω C-terminal domains (CTDs) of the α subunits have additional regulatory roles such as interacting with DNA or with other factors (9 kDa) rpoZ ω subunit serves as a chaperone for β´ omega (150 kDa) rpoB β and β´subunits form a claw with the reactive Mg2+ and catalytic ´ (155 kDa) rpoC active site at the claw center (70 kDa) rpoD three main functions: sigma 1) recognition of specific promoter sequences; 2) position the RNAP holoenzyme at a target promoter 3) facilitate unwinding of the DNA duplex near the transcript start site RNA polymerase recognition of the promoter and the role of the sigma factor Nat Rev Microbiol. 2004 Jan;2(1):57-65. Transcription cycle by bacterial RNAP RNA DNA Core RNA Holoenzyme polymerase TEC RPc RPi RPo modified Annu. Rev. Microbiol. 2013. 67:113–39 Transcription termination in bacteria Rho-independent transcription termination Rho-dependent transcription termination Stem-loop structures as transcription terminators Stem-loop structures as transcription terminators Housekeeping and alternative factors in E. coli ß 38 70 ß' ß ß 70 32 ß' 38 ß' ß 32 ß' TTGACA N15-17 TATAAT TCTNCCCTTGAA N13-15 CCCCATNTA TTGACA N15 TCTATACTT housekeeping genes heat shock response stationary phase genes Regulation of gene transcription by transcription factors (activators and repressors) Simple signal transduction-activator and repressor Inactive regulator (monomer) Signal Conformational change dimerization Active regulator (dimer) DNA-binding Activation Repression The major groove is binding site of regulatory proteins Major and minor groove dimensions the minor groove is 5Å wide, too narrow to fit entire α-helices the major groove is 12Å wide, binding site of regulatory proteins through α-helices Figure 7-6 Molecular Biology of the Cell (© Garland Science 2008) General structure of DNA binding gene regulatory proteins Regulation Dimerization DNA binding The Helix-Turn-Helix (HTH) motif: most common DNA-binding motifs Figure 7-10 Molecular Biology of the Cell (© Garland Science 2008) The Helix-Turn-Helix (HTH) motif: most common DNA-binding motifs Some helix-turn-helix DNA-binding proteins. Figure 7-11 Molecular Biology of the Cell (© Garland Science 2008) Interaction between DNA and HTH motif of DNA-binding proteins Inverted or direct repeats are “operators” for recognition by transcriptional regulators head-to-head dimer head-to-tail dimer 5´ TTACGnnnnnnCGTAA 3´ 3´ AATGCnnnnnnGCATT 5´ inverted repeat 5´ TTACGnnnnnnTTACG 3´ 3´ AATGCnnnnnnAATGC 5´ direct repeat 5´ TTACGCGTAA 3´ 3´ AATGCGCATT 5´ palindrome Mechanisms of RNAP activation by transcriptional regulators Class I activators Class II activators Activation by conformational changes Class I activators Class I activation. The activator is bound to an upstream site and contacts the αCTD of RNA polymerase, thereby recruiting the RNA polymerase to the promoter Flexible linker between -CTD and -NTD allows binding to several promoters RNAP sigma RBS ORF T -35 -10 +1 Class I CRP-cAMP activates the lac promoter by Class-I mechanism (binding to -61.5) CAP: catabolite activator protein or CRP: cAMP receptor protein FEMS Microbiol Rev 34 (2010) 611–627 Class II activators Class II activation. The Class-II activator binds adjacent to the –35 promoter element and interacts with sigma factor. (Example: CRP-cAMP binding to galP1 promoter) RNAP sigma RBS ORF T -41 -35 -10 +1 Class II CRP-cAMP activates the galP1 promoter through a Class II mechanism, binding to a DNA site centred at 41.5 FEMS Microbiol Rev 34 (2010) 611–627 Activation by conformational changes Activation by conformation changes. The activator binds to the promoter elements and realigns the –10 and–35 elements that the RNA polymerase holoenzyme can recognize the promoter. Conformational promoter changes compensate for the overlong 19bp spacer that RNAP can recognize the promoter (Example are MerR-type regulators that bind drugs, e.g. BmrR) RNAP sigma RBS ORF T -35 -10 +1 Activation by conformational changes in the bmr promoter by MerR-family cationic drug-responsive BmrR BmrR dimer bound to DNA bmr promoter 19-bp spacer BmrR bound spacer 17-bp spacer Nature 409, 378-382, 2001 Mechanisms of RNAP repression by transcriptional regulators: Repression by steric hindrance Repression by looping Repression by steric hindrance Repression by steric hindrance. a) The repressor binding site overlaps promoter elements and blocks promoter recognition by the RNA polymerase holoenzyme. b) The repressor binding site overlaps with the RBS or start of coding sequence and blocks elongation. RNAP sigma RBS ORF T -35 -10 +1 The tryptophan-repressor (TRAP) Figure 7-35 Molecular Biology of the Cell (© Garland Science 2008) Repression by looping P S RBS ORF T -35 -10 +1 RBS ORF T S Repression by looping. +1 P Repressors bind to distal sites of the promoter and interact by looping, repressing the intervening promoter. -35 -10 Repression of the lac operon by LacI Aus: Slonczewski, Foster, Mikrobiologie, 2.Auflage, Springer Verlag (2012) Sometimes transcription factors can as activators and repressors of transcription Figure 7-38 Molecular Biology of the Cell (© Garland Science 2008) AraC: repressor and activator of the araBAD operon Kontrolle der Gene des Arabinosekatabolismus (araBAD) durch AraC AraC: repressor and activator of the araBAD operon - Arabinose AraC Repressor + Arabinose AraC Activator AraC: repressor and activator of the araBAD operon - Arabinose AraC Repressor + Arabinose AraC Activator Examples for complex regulation of carbon-catabolite repression and lactose utilization in E. coli The lac-operon of E. coli The lac-operon of E. coli 1920-2013 Lactose utilization in E. coli Lactose = galactose-(β1-4)-glucose H2O β-Galactosidase + Galactose Glucose Lactose utilization in E. coli Lactose = galactose-(β1-4)-glucose Allolactose = galactose-(β1-6)-glucose The lac operon of E. coli Aus: Slonczewski, Foster, Mikrobiologie, 2.Auflage, Springer Verlag (2012) Repression of the lac operon by LacI Without lactose repression by LacI: Aus: Slonczewski, Foster, Mikrobiologie, 2.Auflage, Springer Verlag (2012) Inactivation of LacI by allolactose + Lactose: inactivation of LacI by the inductor allolactose: Activation of lacZYA operon expression by CRP-cAMP + Lactose: activation by CRP-cAMP: O3 CRP O1 O2 P P 5‘ +1 +1 3‘ lacI lacZ lacY lacA CRP: cAMP receptor protein or CAP: catabolite activator protein Aus: Slonczewski, Foster, Mikrobiologie, 2.Auflage, Springer Verlag (2012) Activation of lacZYA operon expression by CRP-cAMP - Lactose Promotor schwach http://upload.wikimedia.org/wikipedia/commons/thumb/e/e7/DNA_simple.svg/220px-DNA_simple.svg.png Keine Proteine für + Lactose Verwertung weiterer C-Quellen cAMP CRP CRP http://upload.wikimedia.org/wikipedia/commons/thumb/e/e7/DNA_simple.svg/220px-DNA_simple.svg.png CRP CRP RNAP CRP CRP http://upload.wikimedia.org/wikipedia/commons/thumb/e/e7/DNA_simple.svg/220px-DNA_simple.svg.png Enzyme/Proteine für Aufnahme/Verwertung von Lactose Activation of lacZYA operon expression by CRP-cAMP Class-I-activator Without lactose, LacI blocks the CRP binding site Activation of lacZYA operon expression + Lactose: Inactivation of LacI and activation by CRP-cAMP: Zusammenhang PTS und C-Katabolitenrepression in E. coli Glucose bevorzugte C-quelle und Energiequelle Anwesenheit von Glucose unterdrückt die Aufnahme alternativer C-quellen (e.g. Lactose, Maltose, Mannose…) = C-Katabolitenrepression Frage: Wie wird durch das PTS die Aufnahme und Verwertung alternativer C-quellen reguliert ? Glucose repression of the lac operon (+ glucose/+lactose) + glucose/+lactose Diauxie Growth experiment CCR= carbon catabolite repression Glucose represses utilization of lactose as alternative carbon source Aus: Michael T. Madigan, John M. Martinko u.a.; Brock Mikrobiologie,13th edition (2013), Pearson C-Katabolitenrepression in E. coli: Das Phosphotransferase-System (PTS) PEP EI HPr~P EIIA EII Glc Pyruvat B C EI ~P HPr EIIA~P Glucose – wichtigster Nährstoff (C- und Energiequelle) Glucosehunger wird gemessen über: Auslastung der Transportkapazität des PTS: viel Glucose EIIA hohe Konzentration wenig Glucose EIIA~P hohe Konzentration cAMP Aktivierung des cAMP-Rezeptorprotein (CRP) und Expression kataboler Gene für alternative C-quellen C-Katabolitenrepression in E. coli: Das Phosphotransferase-System (PTS) a) Glucose im Überschuss Induktorausschluß: -viel EIIA -Hemmung der Lactosepermease LacY LacY PEP EI HPr~P EIIA EII Glc Pyruvat B C EI ~P HPr EIIA~P Bei Glucose und Lactose: keine Aufnahme von Lactose, da Lactosepermease durch EIIA reprimiert =Inductorausschluß C-Katabolitenrepression in E. coli: PTS und Inductor-Exclusion + glucose Viel EIIA: Hemmung der Transport-Systeme für alternative C-Quellen Josef Deutscher et al. Microbiol. Mol. Biol. Rev. 2014;78:231-256 C-Katabolitenrepression in E. coli: Das Phosphotransferase-System b) Glucosehunger: cAMP-CRP activates expression of catabolic genes (e.g. lac operon by lactose) EII Glc PEP EI HPr~P EIIA B C Pyruvat EIIA~P EIIA~P + AC Adenylat-Cyclase EI ~P HPr wird aktiviert cAMP-Level hoch cAMP cAMP aktiviert cAMP- Rezeptorprotein (CRP) Aus: Slonczewski, Foster, Mikrobiologie, 2.Auflage, Springer Verlag (2012) C-Katabolitenrepression in E. coli: PTS, Induktorausschluß und CRP-cAMP + glucose - glucose + alternative carbon sources Boris Görke & Jörg Stülke, Nature Reviews Microbiology vol 6: 613–624 (2008)doi:10.1038/nrmicro1932 + LacI bound (Inductor exclusion) + CAP-cAMP cannot bind Figure 7-39 Molecular Biology of the Cell (© Garland Science 2008) Die lac operon regulation ohne Lactose mit Lactose mit Lactose ohne Lactose ohne Glucose ohne Glucose mit Glucose mit Glucose Bildung von Allolactose LacI Repressor EIIGlcA-P / EIIGlcA Induktor- ausschluss (IA) AC Aktivität cAMP Spiegel CAP lacZYA Expression Die lac operon regulation ohne Lactose mit Lactose mit Lactose ohne Lactose ohne Glucose ohne Glucose mit Glucose mit Glucose Bildung von nein ja nein nein Allolactose LacI Repressor aktiv inaktiv aktiv (da IA) aktiv EIIGlcA-P / EIIGlcA hoch hoch niedrig niedrig Induktor- nein nein ja nein ausschluss (IA) AC Aktivität hoch hoch niedrig niedrig cAMP Spiegel hoch hoch niedrig niedrig CAP aktiv aktiv inaktiv inaktiv lacZYA sehr niedrig hoch sehr niedrig sehr niedrig Expression (Basalniveau) Repression und Repression und Repression Aktivierung fehlende Aktivierung fehlende Aktivierung Summary: regulation of bacterial gene expression Regulation of bacterial RNAP activity by transcription factors promoter, RNA polymerase, transcription cycle Transcriptional regulators: positive regulation Transcriptional regulators: negative regulation, DNA looping dual function regulators (AraC) Global control of the lac operon LacI (direct negative regulation) CRP-cAMP (class-I activator) inducer exclusion/PTS for carbon-catabolite repression Applications of the lac-operon regulatory circuit in molecular biology inducible expression systems (LacI/IPTG) LacZ-assays and synthetic ß-galactosids