Genome 15 PDF
Document Details
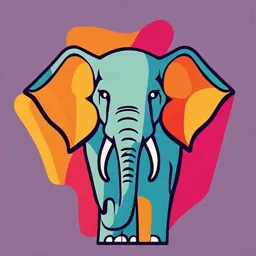
Uploaded by WorthString7417
Philadelphia University - Jordan
Song Tan
Tags
Summary
This document is an outline of the chapter on gene regulation in eukaryotes. It covers regulatory transcription factors, chromatin remodeling, histone variants, histone modifications, DNA methylation, and a comparison of transcriptional regulation in bacteria, archaea, and eukaryotes. The document aims to explain the processes of gene expression and regulation in eukaryotes.
Full Transcript
CHAPTER OUTLINE 15.1 Regulatory Transcription Factors and Enhancers 15.2 Chromatin Remodeling, Histone Binding of a regulatory Variants, and Histone Modifications transcription...
CHAPTER OUTLINE 15.1 Regulatory Transcription Factors and Enhancers 15.2 Chromatin Remodeling, Histone Binding of a regulatory Variants, and Histone Modifications transcription factor to 15.3 DNA Methylation DNA. Certain proteins, known as regulatory 15.4 Gene Activation and Gene transcription factors, Repression have the ability to bind into the major groove of 15.5 A Comparison of Transcriptional DNA and regulate gene Regulation in Bacteria, Archaea, transcription. and Eukaryotes Song Tan, Penn State University, 15 www.bmb.psu.edu/faculty/tan/lab GENE REGULATION IN EUKARYOTES I: GENERAL FEATURES OF TRANSCRIPTIONAL REGULATION Gene expression refers to the process by which the information Translation (discussed in Chapters 13 and 17) within a gene is accessed, first to synthesize RNA and polypeptides, 1. Proteins that bind to the 5′ of mRNA regulate translation and and eventually to affect the properties of cells and the phenotype of those that bind to the 3′ end may influence mRNA degradation. multicellular organisms. For cells and organisms to develop and func- 2. Small RNAs, called miRNAs and siRNAs, silence the tion properly, the level of expression of most genes must be carefully translation of mRNA, a process called RNA interference. controlled so it is not too high or not too low. Gene regulation refers Posttranslation (discussed in Chapters 14 and 23) to the phenomenon in which the level of gene expression can vary Feedback inhibition and covalent modifications regulate protein under different conditions—the level can be increased or decreased. function. From a molecular perspective, gene expression in eukaryotes can be The ability to regulate gene expression provides many ben- efits to eukaryotic organisms—a category that includes protists, regulated at many different steps in the process that gives rise to poly- fungi, plants, and animals. peptides and functional proteins. These steps include the following: ∙∙ Like their prokaryotic counterparts, eukaryotic cells need Transcription (discussed in this chapter and Chapter 16) to adapt to changes in their environment. For example, eu- 1. Regulatory transcription factors can activate or inhibit tran- karyotic cells respond to changes in nutrient availability scription. through enzyme adaptation, much as prokaryotic cells do. 2. The arrangements and composition of nucleosomes influ- ∙∙ Among plants and animals, multicellularity and a more ence transcription. complex cell structure demand a much greater level of gene 3. DNA methylation (usually) inhibits transcription. regulation than occurs in bacteria. Gene regulation is nec- essary to establish and maintain the differences in structure RNA modification (discussed in Chapter 12) and function among distinct cell types. It is amazing that 1. Alternative splicing alters exon choices. the various cells within a multicellular organism usually 2. RNA editing alters the base sequence of RNAs. contain the same genetic material, yet phenotypically may 387 bro50795_ch15_387-412.indd 387 17/06/23 11:13 AM 388 C H A P T E R 1 5 :: GENE REGULATION IN EUKARYOTES I: GENERAL FEATURES OF TRANSCRIPTIONAL REGULATION be quite different. For example, the appearance of a human The term transcription factor is broadly used to describe a cate- nerve cell seems about as similar to that of a muscle cell as gory of proteins that influence the ability of RNA polymerase to an amoeba is to a paramecium. In spite of these phenotypic transcribe DNA into RNA. We will focus much of our attention on differences, a human nerve cell and muscle cell actually transcription factors that affect the ability of RNA polymerase to contain the same complement of human chromosomes. begin the transcription process. Such transcription factors regulate Nerve and muscle cells look strikingly different because of the binding of the preinitiation complex to the core promoter and/ gene regulation rather than differences in DNA content. or control the switch from the initiation to the elongation stage of transcription. In this chapter, our focus is on the regulation of gene transcription. Two types of transcription factors play a key role in these Researchers have discovered that most eukaryotic genes, particu- processes. In Chapter 12, we considered general transcription larly those found in multicellular species, are transcriptionally reg- factors (GTFs), which are required for the binding of RNA poly- ulated by many factors. This phenomenon is called combinatorial merase to the core promoter and for progression to the elongation control because the combination of many factors determines the stage. General transcription factors are necessary for any tran- expression of any given gene. At the level of transcription, the fol- scription to occur. In addition, eukaryotic cells possess a diverse lowing factors commonly contribute to combinatorial control: array of regulatory transcription factors (RTFs) that serve to 1. One or more activator proteins may stimulate the ability of regulate the rate of transcription of target genes. RNA polymerase to initiate transcription. 2. One or more repressor proteins may inhibit the ability of RNA polymerase to initiate transcription. Structural Features of Regulatory Transcription 3. The function of activators and repressors may be modulated in Factors Allow Them to Bind to DNA a variety of ways, including the binding of small effector mol- Genes that code general and regulatory transcription factors have ecules, protein-protein interactions, and covalent modifications. been identified and sequenced from a wide variety of eukaryotic 4. Regulatory proteins may alter the composition or arrange- species, including yeast, plants, and animals. Several different ment of nucleosomes in the vicinity of a promoter, thereby families of evolutionarily related transcription factors have been affecting transcription. Also, histone proteins may be discovered. In recent years, the molecular structures of transcrip- covalently modified. tion factor proteins have become an area of intense research. These 5. DNA methylation may inhibit transcription, either by pre- proteins contain regions, called domains, that have specific func- venting the binding of an activator protein or by recruiting tions. For example, one domain of a transcription factor may have proteins that change the structure of chromatin in a way a DNA-binding function, and another may provide a binding site that inhibits transcription. for a small effector molecule. When a domain or a portion of a 6. As discussed in Chapter 16, the formation of heterochro- domain has a very similar structure in many different proteins, the matin may inhibit gene expression in localized regions of a structurally similar region is called a motif. chromosome. Figure 15.1 depicts several different motifs found in tran- All six of these factors can contribute to the regulation of a single scription factor proteins. The protein secondary structure known gene, or possibly only three or four will play a role. In most cases, as an α helix occurs frequently in transcription factors. Why is the transcriptional regulation is aimed at controlling the initiation of α helix common in such proteins? The explanation is that the α transcription at the promoter or at an early point in the elongation helix is the proper width to bind into the major groove of the phase. In Section 15.1, we will survey the first three factors that DNA double helix. In helix-turn-helix and helix-loop-helix mo- may contribute to combinatorial control of transcription. In the tifs, an α helix called the recognition helix makes contact with next two sections, we will consider the fourth and fifth factors; the and recognizes a base sequence along the major groove of the sixth factor is considered in Chapter 16. The process of gene acti- DNA (Figure 15.1a, b). Such motifs are able to recognize a par- vation as it occurs in living cells is described in Section 15.4, which ticular base sequence because they have a surface that is bio- also includes specific examples. Finally, the last section compares chemically complementary to that of the DNA, allowing for a transcriptional regulation in bacteria, archaea, and eukaryotes. series of favorable electrostatic and van der Waals interactions between the protein and the base pairs. Also, such proteins make a large number of contacts with the DNA backbone, including 15.1 REGULATORY salt bridges between negatively charged phosphates and posi- TRANSCRIPTION FACTORS tively charged amino acid side chains and hydrogen bonds with uncharged amino acids. AND ENHANCERS Recall that the major groove is a region of the DNA double Learning Outcomes: helix where the nucleotide bases are in contact with the water in cellular fluid. Hydrogen bonding between the amino acid side 1. Distinguish between general and regulatory transcription factors. chains in an α helix and the nucleotide bases in the DNA is one 2. Describe how a regulatory transcription factor binds to an way that a transcription factor binds to a specific DNA sequence. enhancer. In addition, the recognition helix often contains many positively 3. Describe three ways that the function of a regulatory tran- charged amino acids (e.g., arginine and lysine) that favorably in- scription factor can be modulated. teract with the DNA backbone, which is negatively charged. bro50795_ch15_387-412.indd 388 17/06/23 11:13 AM 15.1 Regulatory Transcription Factors and Enhancers 389 Loop Recognition helix Turn Recognition helix (a) Helix-turn-helix motif (b) Helix-loop-helix motif Zn2+ 1 Zinc finger Recognition Leu Leu Leu Leucine helix side chains Leu Leu Leu (zipper) Zn2+ 2 β sheet Coiled coil formed from two different proteins Zn2+ 3 Zinc Zn2+ ion 4 Recognition helix (c) Zinc finger motif (d) Leucine zipper motif FI GURE 15.1 Structural motifs found in transcription factor proteins. Certain types of protein secondary structures are found in many dif- ferent transcription factors. In this figure, α helices are shown as cylinders and β sheets as flattened arrows. (a) Helix-turn-helix motif: Two α helices are connected by a turn. The α helices bind to the DNA within the major groove. (b) Helix-loop-helix motif: A short α helix is connected to a longer α helix by a loop. In this illustration, a dimer is formed from the interactions of two helix-loop-helix motifs, and the longer helices are binding to the DNA. (c) Zinc finger motif: Each zinc finger is composed of one α helix and two antiparallel β sheets. A zinc ion (Zn2+), shaded red, holds the zinc fin- ger together. This illustration shows four zinc fingers in a row. (d) Leucine zipper motif: The leucine zipper promotes the dimerization of two transcrip- tion factor proteins. Two α helices (termed a coiled coil) are intertwined due to interactions between their leucines (see inset). CONCEPT CHECK: Explain how an α helix in a transcription factor protein is able to function as a recognition helix. A zinc finger motif is composed of one α helix and two dimerization and DNA binding of two proteins that have β sheets that are held together by a zinc (Zn2+) metal ion (Fig- several leucine amino acids (a zipper). Leucines in both ure 15.1c). The zinc finger can also recognize DNA sequences proteins interact (“zip up”), resulting in protein dimerization. within the major groove. Two identical transcription factors may come together to form A second interesting feature of certain motifs is that a homodimer, or two different transcription factors can form a they promote protein dimerization. The leucine zipper (Fig- heterodimer. As discussed later, the dimerization of transcrip- ure 15.1d) and helix-loop-helix motif (see Figure 15.1b) medi- tion factors can be an important way to modulate their ate protein dimerization. For example, Figure 15.1d depicts the function. bro50795_ch15_387-412.indd 389 17/06/23 11:13 AM 390 C H A P T E R 1 5 :: GENE REGULATION IN EUKARYOTES I: GENERAL FEATURES OF TRANSCRIPTIONAL REGULATION Regulatory Transcription Factors Recognize Enhancer Regulatory Elements Within Enhancers Some RTFs exert their effects by influencing the ability of RNA Regulatory element polymerase to begin transcription of a particular gene. They typi- cally recognize cis-acting regulatory sequences that are located in the same chromosome as the gene they regulate; such elements Activator may be close to or far way from the core promoter. These DNA protein sequences are analogous to the operator sites found near bacterial Preinitiation complex promoters. Such sequences are generally known as regulatory elements (also called regulatory sequences or response elements). As described in Sections 15.2 and 15.4, changes in chromatin struc- DNA- ture are often needed for RTFs to bind to regulatory elements. bending proteins An enhancer is a DNA region, usually 50–1000 bp in length, that contains one or more regulatory elements. If the binding of an RTF to an enhancer increases the rate of transcription, such a regu- Core latory transcription factor is termed an activator. In Figure 15.2a, promoter RNA transcription the enhancer is not adjacent to the core promoter so a bend in the is increased. DNA must occur to bring the enhancer and core promoter close to each other. DNA-bending proteins facilitate this process. (a) Gene activation Alternatively, regulatory transcription factors may act as re- pressors by binding to enhancers and preventing transcription Repressor from occurring (Figure 15.2b). Repressors also bind to enhancers protein but they do not promote the binding of GTFs, mediator, and RNA polymerase to the core promoter or they do not allow RNA poly- merase to proceed to the elongation phase of transcription. The mechanisms by which an activator stimulates transcription or a Enhancer Core repressor inhibits transcription are discussed in Section 15.4. promoter RNA transcription is inhibited. When an activator binds to an enhancer, such binding can stimulate transcription 10- to 1000-fold, a phenomenon known as (b) Gene repression up regulation. Alternatively, the binding of repressors that inhibit transcription result in down regulation. F I G URE 1 5. 2 Overview of transcriptional regulation by Regulatory elements and the enhancers that contain them regulatory transcription factors. A regulatory transcription factor are orientation-independent, or bidirectional. This means that a can act as either an activator to increase the rate of transcription or a regulatory element can function in either the forward or the re- repressor to decrease the rate of transcription. (a) The preinitiation verse direction. For example, let’s consider an element with a for- complex is bound to the core promoter; the DNA of the core pro- moter is shown in tan. The activator binds to a regulatory element ward orientation as shown below: within a distant enhancer. A loop in the DNA allows the activator 5′–GATA–3′ to be close to the core promoter and preinitiation complex. Some 3′–CTAT–5′ activators, such as the one shown here, may interact with the preini- tiation complex in a way that increases the rate of transcription. This element is also bound by a regulatory transcription factor and (b) When a repressor binds to an enhancer, it may inhibit the forma- enhances transcription even when it is rotated 180o and oriented in tion of the preinitiation complex, as shown here, or it may prevent the reverse direction: the preinitiation complex from proceeding to the elongation phase of transcription. 5′–TATC–3′ 3′–ATAG–5′ Striking variation is also observed in the locations of enhanc- role in immunity, these researchers identified a region that is ers relative to a gene’s promoter. Enhancers are sometimes far away from the core promoter, but is needed for high levels located in a region within 200 bp upstream from the core pro- of transcription to take place. In some cases, enhancers are lo- moter. More commonly, they are fairly distant from the core cated downstream from the promoter site and may even be promoter, even 100,000 bp away, yet exert strong effects on found within introns, the noncoding parts of genes. As you may the ability of RNA polymerase to initiate transcription at the imagine, the variation in the orientation and location of en- core promoter! hancers profoundly complicates the efforts of geneticists to Enhancers were first discovered by Susumu Tonegawa identify those regulatory elements that affect the expression of and coworkers in the 1980s. While studying genes that play a any given gene. bro50795_ch15_387-412.indd 390 17/06/23 11:13 AM 15.1 Regulatory Transcription Factors and Enhancers 391 The Functions of RTFs Can Be Modulated in be affected by covalent modifications, such as the attachment Three Ways of a phosphate group (Figure 15.3c). As discussed in Sec- tion 15.4, the phosphorylation of activators can control their Thus far in this section, we have considered the structures of ability to stimulate transcription. RTFs and their abilities to bind to DNA and influence tran- scription. The functions of the RTFs themselves must also be modulated. Why is this necessary? The answer is that the genes they control must be turned on at the proper time, in the correct 15.1 COMPREHENSION QUESTIONS cell type, and under the appropriate environmental conditions. 1. As described in the chapter introduction, combinatorial control Therefore, eukaryotes have evolved different ways to modulate refers to the phenomenon that the functions of these proteins. a. transcription factors always combine with each other when The functions of RTFs are controlled in three common regulating genes. ways: b. the combination of many factors determines the expression ∙∙ The binding of a small effector molecule of any given gene. ∙∙ Protein-protein interactions c. small effector molecules and regulatory transcription factors ∙∙ Covalent modifications are found in many different combinations. Figure 15.3 depicts these three mechanisms for modulating the d. genes and regulatory transcription factors must combine functions of RTFs. Usually, one or more of these modulating with each other during gene regulation. effects are important in determining whether a transcription 2. An RTF that binds to a regulatory element typically contains factor binds to the DNA or influences transcription by RNA _________ that binds to the ________ of the DNA. polymerase. For example, a small effector molecule may bind a. an α helix, backbone to an RTF and promote its binding to DNA (Figure 15.3a). In b. an α helix, major groove Section 15.4, we will see that steroid hormones function in this c. a β sheet, backbone manner. Another important mechanism of modulation is via d. a β sheet, major groove protein-protein interactions (Figure 15.3b). The formation of homodimers and heterodimers is a fairly common means of 3. A bidirectional regulatory element has the following sequence: controlling transcription. Finally, the function of an RTF can 5′–GTCA–3′ 3′–CAGT–5′ Which of the following sequences would also be a functional regulatory element? Hormone a. 5′–ACTG–3′ c. 3′–GTCA–5′ Transcription 3′–TGAC–5′ 5′–CAGT–3′ factor b. 5′–TGAC–3′ d. 3′–TGAC–5′ Enhancer 3′–ACTG–5′ 5′–ACTG–3′ 4. RTFs can be modulated by a. the binding of small effector molecules. b. protein-protein interactions. (a) Binding of a small effector molecule such as a hormone c. covalent modifications. Transcription d. any of the above. factor Transcription factor Homodimer (b) Protein-protein interaction F I G URE 15. 3 Common ways to modulate Transcription factors PO42– PO42– the function of regulatory transcription factors. (a) The binding of an effector molecule such as a hor- mone may influence the ability of an RTF to bind to the DNA. (b) Protein-protein interactions among tran- scription factor proteins may influence their functions. (c) Covalent modifications, such as phosphorylation, (c) Covalent modification by phosphorylation may alter transcription factor function. bro50795_ch15_387-412.indd 391 17/06/23 11:13 AM 392 C H A P T E R 1 5 :: GENE REGULATION IN EUKARYOTES I: GENERAL FEATURES OF TRANSCRIPTIONAL REGULATION orchestrate a change in chromatin from closed to open conforma- 15.2 CHROMATIN REMODELING, tion by altering nucleosomes. HISTONE VARIANTS, AND One way to change chromatin structure is through ATP- HISTONE MODIFICATIONS dependent chromatin remodeling. In this process, the energy of ATP hydrolysis is used to drive changes in the positions and/or Learning Outcomes: compositions of nucleosomes, thereby making the DNA more or 1. Describe how chromatin-remodeling complexes alter less amenable to transcription. Therefore, chromatin remodeling nucleosomes. is important for both the activation and repression of 2. Define histone variant, and explain why histone variants are transcription. functionally important. The remodeling process is carried out by a protein complex 3. Explain how histone modifications affect transcription. that recognizes nucleosomes and uses ATP to alter their configu- ration. All chromatin-remodeling complexes have a catalytic ATPase subunit called DNA translocase; this ATPase subunit, In Section 15.1, we considered how RTFs can bind to regulatory similar to what is found in motor proteins, moves along the DNA. elements within enhancers and thereby influence transcription. Eukaryotes have multiple families of chromatin remodeling com- In eukaryotes, certain features of chromatin structure may act as plexes. Though their names may differ depending on the species, obstacles for the binding of RTFs to enhancers. If chromatin is common families of chromatin-remodeling complexes include the in a closed conformation, transcription may be difficult or SWI/SNF-family, the ISWI-family, the INO80-family, and the Mi- impossible. By comparison, chromatin that is in an open con- 2-family. The names of these complexes sometimes refer to the formation is more easily accessed by transcription factors and effects of mutations in genes that code them. For example, the RNA polymerase, allowing transcription to occur. As discussed abbreviations SWI and SNF refer to the effects that occur in yeast in Chapter 16, a closed conformation may occur via the when these remodeling complexes are defective. SWI mutants are conversion of euchromatin, which is more loosely compacted, defective in mating-type switching, and SNF mutations create a into tightly compacted heterochromatin. In addition, researchers sucrose nonfermenting phenotype. have determined that the following factors can affect gene How do chromatin remodeling complexes change chromatin transcription: structure? Three effects are possible: ∙∙ Precise positioning of nucleosomes at or near promoters ∙∙ One result of ATP-dependent chromatin remodeling is a ∙∙ Presence of histone variants change in the positions of nucleosomes (Figure 15.4a). ∙∙ Covalent modification of histones This may involve shifts in nucleosomes to new locations or In this section, we will examine molecular mechanisms that changes in the relative spacing of nucleosomes over a long explain how changes in these three factors can occur. stretch of DNA. ∙∙ A second possible effect of remodeling is that histone oc- tamers are evicted from the DNA, thereby creating gaps Chromatin-Remodeling Complexes Alter the where nucleosomes are not found (Figure 15.4b). Positions and Compositions of Nucleosomes ∙∙ A third possibility is that remodeling may change the com- position of nucleosomes by removing standard histones The term ATP-dependent chromatin remodeling, or simply and replacing them with histone variants (Figure 15.4c). chromatin remodeling, refers to dynamic changes in the struc- The functions of histone variants are described next. ture of chromatin that occur during the life of a cell. These changes range from local alterations in the positioning of one or a few nu- cleosomes to larger changes that affect chromatin structure over a Histone Variants Play Specialized Roles in longer distance. Chromatin remodeling is carried out by ATP- dependent chromatin-remodeling complexes, which are a set of Chromatin Structure and Function diverse multiprotein machines that reposition and restructure The genes that code histones H1, H2A, H2B, H3, and H4 are mod- nucleosomes. erately repetitive. The total number of histone genes varies from In recent years, geneticists have been trying to identify the species to species. As an example, the human genome contains steps that promote the interconversion between the closed and over 70 histone genes that have been produced by gene duplication open conformations of chromatin. Nucleosomes have been events during evolution. Most of these genes code standard his- shown to have different positions in cells that normally express a tone proteins. However, a few have accumulated mutations that particular gene compared with cells in which the gene is inac- change the amino acid sequences of histone proteins. These al- tive. For example, in reticulocytes that express the β-globin gene, tered histones are called histone variants. Among eukaryotic an alteration in nucleosome positioning occurs in the promoter species, histone variants have been identified for H1, H2A, H2B, region from nucleotide −500 to nucleotide +200. This alteration and H3, but not for H4. is thought to be an important step in expressing the β-globin What are the consequences of histone variation? Certain gene. Based on the analysis of many genes, researchers have histone variants play specialized roles in chromatin structure and discovered that a key role of some transcriptional activators is to function. In all eukaryotes, histone variants are incorporated bro50795_ch15_387-412.indd 392 17/06/23 11:13 AM 15.2 Chromatin Remodeling, Histone Variants, and Histone Modifications 393 TA B L E 15.1 Standard Human Histones and Examples of Histone Variants ATP-dependent Number of chromatin-remodeling Histone Type Genes in Function complex (not shown) Humans or H1 Standard 11 Standard linker histone H10 Variant 1 Linker histone associated with chromatin compaction and gene repression H2A Standard 15 Standard core histone Change in the relative positions Change in the spacing of a few nucleosomes of nucleosomes over a MacroH2A Variant 1 Core histone that is abundant on long distance the inactivated X chromosome in female mammals. Plays a role in (a) Change in nucleosome location chromatin compaction. H2A.Z Variant 1 Core histone that is usually found in nucleosomes that flank the transcriptional start site of promoters. Plays a role in gene transcription. ATP-dependent chromatin-remodeling H2A.Bbd Variant 1 Core histone that promotes open complex chromatin. Plays a role in gene activation. H2A.X Variant 1 Plays a role in DNA repair H2B Standard 17 Standard core histone Histone octamers are removed. spH2B Variant 1 Core histone found in the telomeres of sperm cells (b) Eviction of histone octamers H3 Standard 10 Standard core histone cenH3 Variant 1 Core histone found at centromeres. Involved with the binding of kinetochore proteins. H3.3 Variant 2 Core histone that promotes open chromatin. Plays a role in gene ATP-dependent activation. chromatin-remodeling H4 Standard 14 Standard core histone complex Histone variants Table 15.1 describes the standard histones and a few his- tone variants that are found in humans. A key role of many his- tone variants is to regulate the structure of chromatin, thereby (c) Replacement with histone variants influencing gene transcription. Such variants can have opposing effects. The incorporation of histone H2A.Bbd into a chromo- FI GURE 15.4 ATP-dependent chromatin remodeling. The top somal region where a particular gene is found favors gene activa- part of each illustration shows five nucleosomes. Chromatin-remodeling tion. In contrast, the incorporation of histone H10 represses gene complexes may (a) change the locations of nucleosomes, (b) remove his- expression. tone octamers from the DNA, or (c) replace core histones with histone Although our focus in this chapter is on gene regulation, it is variants. The chromatin-remodeling complex is not shown in this figure; worth noting that histone variants play other important roles. For only its effects are shown. example, histone cenH3 (also called CENP-A), which is a variant CONCEPT CHECK: How might eviction of histone octamers affect transcription? of histone H3, is found at the centromere of each chromosome and functions in the binding of kinetochore proteins. Histone cenH3 is into a subset of nucleosomes to create functionally specialized required for the proper segregation of eukaryotic chromosomes. regions of chromatin. In most cases, the standard histones are in- Other histone variants are primarily found at specialized sites in corporated into the nucleosomes while new DNA is synthesized certain cells. Histone macroH2A is found on the inactivated X during S phase of the cell cycle. Later, some of the standard his- chromosome in female mammals, whereas spH2B is found in the tones are replaced by histone variants via chromatin-remodeling telomeres in sperm cells. Finally, certain histone variants appear complexes. to play a role in DNA repair. For example, histone H2A.X becomes bro50795_ch15_387-412.indd 393 17/06/23 11:13 AM 394 C H A P T E R 1 5 :: GENE REGULATION IN EUKARYOTES I: GENERAL FEATURES OF TRANSCRIPTIONAL REGULATION phosphorylated where a double-stranded DNA break occurs. This ac phosphorylation is thought to be important for the proper repair of Lys ac 5 p that break. p Amino-terminal tail ac SerLys 15 ac Lys Ser 20 Lys The Histone Code Also Controls Gene ac ac 10 Lys Lys 15 Transcription 5 10 20 H2B As described in Chapter 10, each of the core histone proteins con- H2A sists of a globular domain and a flexible, charged amino-terminus Globular domain called an amino-terminal tail (refer back to Figure 10.17a). The DNA wraps around the globular domains, and the amino-terminal tails protrude from the chromatin. Particular amino acids in the ac amino-terminal tails of both standard histones and histone variants p ac Lys 10 Lys are subject to several types of covalent modifications, including Ser m ac acetylation, methylation, and phosphorylation. Over 50 different m Arg Lys 15 ac m Arg 5 Lys enzymes have been identified in mammals that selectively modify m ac m ac Lys Lys 20 the amino-terminal tails of histones. Figure 15.5a shows exam- Arg Lys H4 5 m Lys ples of sites in the tails of H2A, H2B, H3, and H4 that can be ac p 15 20 modified. Lys Ser H3 How do histone modifications affect the level of transcrip- 10 tion? First, they may directly influence interactions within nucleo- somes. For example, positively charged lysines within the core histone proteins can be acetylated by histone acetyltransferases (HATs). The attachment of the acetyl group (—COCH3) elimi- (a) Examples of possible histone modifications nates the positive charge on the lysine side chain, thereby disrupt- ing the electrostatic attraction between the histone protein and the Core histone protein negatively charged DNA backbone and favoring the open confor- DNA is less tightly bound mation (Figure 15.5b). However, histone acetylation is highly re- to the histone proteins. COCH3 versible. Histone deacetylases (HDACs) remove acetyl groups from acetylated histones and thereby favor a tighter contact Histone COCH3 acetyltransferase between histones and the DNA. In addition, histone modifications occur in patterns that are recognized by proteins. According to the histone code hypothe- Histone sis, proposed by Brian Strahl, C. David Allis, and Bryan Turner deacetylase in 2000, the pattern of histone modification acts much like a lan- COCH3 guage or code in specifying alterations in chromatin structure. Acetyl For example, one pattern might specify phosphorylation of the group serine at the first position in H2A and acetylation of the fifth and eighth amino acids in H4, which are lysines. A different pattern (b) Effect of acetylation could invoke acetylation of the fifth amino acid, a lysine, in H2B and methylation of the third amino acid in H4, which is an F I G URE 1 5. 5 Histone modifications and their effects on nucleosome structure. (a) Examples of histone modifications that may arginine. occur on the amino-terminal tail of each of the four core histone proteins. The pattern of covalent modifications to the amino-terminal The abbreviations are: p, phosphate; ac, acetyl group; and m, methyl group. tails provides binding sites for proteins that subsequently affect the (b) Effect of acetylation. When the core histones are acetylated via histone degree of transcription. One pattern of histone modification may acetyltransferase, the DNA becomes less tightly bound to the histones. attract proteins that inhibit transcription, which would silence the Histone deacetylase removes the acetyl groups. Note: The structures of the transcription of genes in the region. A different combination of histone proteins in part (a) are meant to be schematic. H2A and H2B as histone modifications may attract proteins, such as chromatin- well as H3 and H4 actually form intertwined dimers. remodeling complexes, that would alter the positions of nucleo- CONCEPT CHECK: Describe two different ways that histone modifications somes in a way that promotes gene transcription. For example, the may alter chromatin structure. acetylation of histones attracts certain chromatin-remodeling complexes that shift nucleosomes or evict histone octamers, thereby aiding in the transcription of genes. the effects of the covalent modifications that make up the histone Overall, the histone code is known to play an important role code. In Chapter 16, we will consider specific histone modifica- in determining whether the information within the genomes of tions that promote heterochromatin formation and thereby inhibit eukaryotic species is accessed. Researchers are trying to decipher gene transcription. bro50795_ch15_387-412.indd 394 17/06/23 11:13 AM 15.3 DNA Methylation 395 NH2 15.2 COMPREHENSION QUESTIONS H 4 5 3N 1. A chromatin-remodeling complex may 2 Cytosine 6 a. change the locations of nucleosomes. H 1 N O b. evict histones from DNA. H c. replace standard histones with histone variants. d. do any of the above. DNA methyltransferase 2. According to the histone code hypothesis, the pattern of histone modifications acts like a language that NH2 a. influences chromatin structure. CH3 4 3N b. promotes transcriptional termination. 5 5-methylcytosine 6 2 c. inhibits the elongation of RNA polymerase. H 1 O N d. does all of the above. H (a) The methylation of cytosine 15.3 DNA METHYLATION 5′ 3′ C Learning Outcomes: G G C 1. Define DNA methylation, and explain how it affects tran- 3′ 5′ scription. (b) Unmethylated 2. Explain how DNA methylation is heritable. 5′ 3′ We now turn our attention to a regulatory mechanism that involves a CH3 C G direct change in DNA structure. A methyl group (CH3) can be attached G C to the cytosine base in DNA, a process called DNA methylation. The 3′ 5′ methylation of cytosines is common in some, but not all, eukaryotic species. For example, yeast and Drosophila have little or no detectable (c) Hemimethylated methylation of their DNA, whereas DNA methylation in vertebrates and plants is relatively abundant. In mammals, approximately 2–7% of 5′ 3′ the DNA is methylated. In this section, we will examine how DNA CH3 C G methylation occurs and how it controls gene expression. G C CH3 3′ 5′ DNA Methylation Occurs on the Cytosine Base and (d) Fully methylated Usually Inhibits Gene Transcription F I G URE 1 5. 6 DNA methylation on cytosine bases. As shown in Figure 15.6, eukaryotic DNA methylation occurs via (a) Methylation occurs via an enzyme known as DNA methyltransferase, an enzyme called DNA methyltransferase, which attaches a which attaches a methyl group to the number 5 carbon of cytosine. The methyl group to the carbon at the number 5 position of the cyto- CG sequence can be (b) unmethylated, (c) hemimethylated, or (d) fully sine base, forming 5-methylcytosine. The sequence that is usually methylated. In (b) and (c), the C in CH3 refers to carbon, whereas the methylated is shown here: C in the DNA refers to cytosine. CH3 connected by a phosphodiester linkage.) These CpG islands are 5′ CG 3′ commonly 1000–2000 bp in length and contain a large number of 3′ GC 5′ CpG sites. In the case of housekeeping genes—genes that code CH3 proteins required in most cells of a multicellular organism—the cytosine bases in the CpG islands are unmethylated. Therefore, house- Note that this sequence contains cytosines in both strands. Meth- keeping genes tend to be expressed in most cell types. ylation of the cytosine in both strands is termed full methylation, By comparison, other genes are highly regulated and may be whereas methylation of the cytosine in only one strand is called expressed only in a particular cell type. These are tissue-specific hemimethylation. genes. In some cases, it has been found that the expression of such DNA methylation typically inhibits the transcription of eukary- genes may be silenced by the methylation of CpG islands. In otic genes, particularly when it occurs in the vicinity of the promoter. general, unmethylated CpG islands are correlated with active In vertebrates and plants, CpG islands occur near many promoters of genes, whereas suppressed genes contain methylated CpG islands. genes. (Note: CpG refers to a dinucleotide of C and G in DNA that is Thus, DNA methylation can play an important role in the silencing bro50795_ch15_387-412.indd 395 17/06/23 11:13 AM CH3 CpG island CH3 Core promoter CH3 CH3 CH3 CH3 CH3 Enhancer CH3 CpG island Core promoter CH3 Chromatin A methyl-CpG-binding protein in an open binds to the methylated Coding sequence conformation CpG island. Activator protein Methylation CH3 CH3 CH3 CH3 CH3 CH3 CH3 CH3 CH3 CH3 CH3 CH3 CH3 CH3 CH3 Methyl groups block the binding of an activator protein Methyl-CpG- to an enhancer. binding protein The methyl-CpG-binding protein recruits other proteins, such as histone deacetylase, that convert Chromatin the chromatin to a closed (a) Methylation inhibits the binding of an activator protein. conformation. in a closed CH3 CH3 conformation CH3 CH3 CH3 CH3 FI GURE 15.7 Transcriptional silencing via methylation. CH3 (a) The methylation of a CpG island may inhibit the binding of a tran- CH3 scriptional activator protein to the promoter region. (b) The binding of a CH3 Histone methyl-CpG-binding protein to a CpG island may lead to the recruitment deacetylase of other proteins, such as histone deacetylase, that convert chromatin to a closed conformation and thus suppress transcription. (b) Methyl-CpG-binding protein recruits other proteins that change the CONCEPT CHECK: Explain why the events shown in part (a) inhibit transcription. chromatin to a closed conformation. of tissue-specific genes and thereby prevents them from being ex- CpG island. Once bound to the DNA, the methyl-CpG-binding pro- pressed in the wrong tissue. Methylation can affect transcription in tein recruits to that region other proteins that inhibit transcription. two general ways, as described next. For example, methyl-CpG-binding proteins may recruit histone deacetylase to a methylated CpG island near a promoter. Histone Alteration in the Binding of Regulatory Transcription deacetylation removes acetyl groups from the histone proteins, Factors. Because the methyl group on cytosine protrudes into the which makes it more difficult for nucleosomes to be removed from major groove of the DNA, methylation can affect the binding of pro- the DNA. In this way, deacetylation tends to inhibit transcription. teins into that groove, and thereby affect transcription. For example, methylation of CpG islands may prevent or enhance the binding of DNA Methylation Is Heritable regulatory transcription factors to the promoter region. In some Methylated DNA sequences are inherited during cell division. Ex- cases, methylated CpG islands prevent the binding of an activator perimentally, if fully methylated DNA is introduced into a plant or protein to an enhancer (Figure 15.7a). The inability of an activator vertebrate cell, the DNA will remain fully methylated even in sub- protein to bind to the DNA inhibits the initiation of transcription. sequently produced daughter cells. However, if the same sequence However, CG methylation does not slow down the movement of of nonmethylated DNA is introduced into a cell, it will remain RNA polymerase along a gene. In vertebrates and plants, coding re- nonmethylated in the daughter cells. These observations indicate gions downstream from the core promoter usually contain methyl- that the pattern of methylation is retained following DNA replica- ated cytosines, but these do not hinder the elongation phase of tion and, therefore, is inherited in future daughter cells. transcription. This observation suggests that methylation must occur How can methylation be inherited from cell to cell? in the vicinity of the promoter to have an effect on transcription. Figure 15.8 illustrates a molecular model that explains this pro- cess, which was originally proposed by Arthur Riggs, Robin Binding of Methyl-CpG-Binding Proteins. A second way Holliday, and J. E. Pugh. that methylation affects transcription is via proteins known as methyl-CpG-binding proteins, which bind to methylated CpG is- 1. The DNA in a particular cell may become methylated by lands (Figure 15.7b). These proteins contain a domain called the de novo methylation—the methylation of DNA that was methyl-binding domain that specifically recognizes a methylated previously unmethylated. 396 bro50795_ch15_387-412.indd 396 17/06/23 11:13 AM 15.4 Gene Activation and Gene Repression 397 5′ 3′ Once methylation has occurred, it can then be transmitted from C G mother to daughter cells via maintenance methylation. G C The methylation mechanism shown in Figure 15.8 can ex- 3′ 5′ plain the phenomenon of genomic imprinting, which is described in Chapters 5 and 16. In this case, specific genes are methylated de novo methylation during oogenesis or spermatogenesis, but not during both of these processes. Following fertilization, the pattern of methylation is maintained in the offspring. For example, if a gene is methylated 5′ 3′ only during spermatogenesis, the allele that is inherited from the CH3 C G father will be methylated in the somatic cells of the offspring, but G the maternal allele will remain unmethylated. Along these lines, C CH3 3′ 5′ geneticists are eager to determine how variations in DNA methyla- tion patterns may be important for cell differentiation. Methylation DNA replication may be a key way to silence genes in different cell types. However, additional research is necessary to fully understand how specific Hemimethylated genes may be targeted for de novo methylation or demethylation in DNA specific cell types or during different stages of development. 5′ 3′ 5′ 3′ CH3 C C G G G G 15.3 COMPREHENSION QUESTIONS C C CH3 3′ 5′ 3′ 5′ 1. How can methylation affect transcription? a. It may prevent the binding of regulatory transcription Maintenance factors. Fully methylated methylation DNA b. It may enhance the binding of regulatory transcription factors. 5′ 3′ 5′ 3′ c. It may promote the binding of methyl-CpG-binding proteins, CH3 C CH3 C G G G G which inhibit transcription, to a methylated sequence. C CH3 C CH3 d. All of the above are possible ways for methylation to affect 3′ 5′ 3′ 5′ transcription. FI GURE 15.8 A molecular model for the inheritance of DNA 2. The process in which completely unmethylated DNA becomes methylation. The DNA initially undergoes de novo methylation, which is methylated is called a rare, highly regulated event. Once this occurs, DNA replication produces a. maintenance methylation. hemimethylated DNA molecules, which are then fully methylated by b. de novo methylation. DNA methyltransferase. This process, called maintenance methylation, is c. primary methylation. a routine event that is expected to occur for all hemimethylated DNA. d. demethylation. CONCEPT CHECK: What is the difference between de novo methylation and maintenance methylation? 2. When a fully methylated segment of DNA replicates in 15.4 GENE ACTIVATION AND preparation for cell division, the newly made daughter strands contain unmethylated cytosines. Such DNA is said GENE REPRESSION to be hemimethylated. Learning Outcomes: 3. This hemimethylated DNA is efficiently recognized by DNA methyltransferase, which makes it fully methylated. 1. Describe the organization of nucleosome-free regions for an This process is called maintenance methylation, because active gene. it preserves the methylated condition in future cells. 2. Explain how activators bind to enhancers and facilitate the However, maintenance methylation does not act on un- binding of the preinitiation complex. methylated DNA. 3. Describe the key events that occur during the elongation phase of transcription. Overall, maintenance methylation appears to be an efficient pro- 4. Outline the steps of gene regulation via glucocorticoid cess that routinely occurs within vertebrate and plant cells. By receptors and CREB proteins. comparison, de novo methylation and demethylation are infrequent 5. Distinguish between short- and long-term repression. and highly regulated events. According to this view, the initial 6. Describe the method of chromatin immunoprecipitation methylation or demethylation of a given gene can be regulated sequencing, and explain what it is used for. so that it occurs in a specific cell type or stage of development. bro50795_ch15_387-412.indd 397 17/06/23 11:13 AM