اساسيات الكيمياء العضوية 1 PDF
Document Details
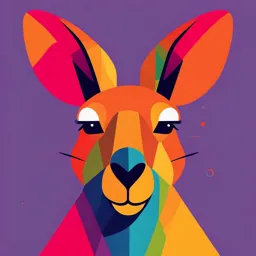
Uploaded by WellInformedEinstein5967
Suez Canal University
Tags
Summary
هذا الملخص يتناول أساسيات الكيمياء العضوية، و يركز بشكل خاص على التأثير الاستحثائي في المركبات العضوية. يوضح الكيفية التي تؤثر بها المجموعات المختلفة على خصائص المركبات العضوية، ويوضح كيفية توازن هذه التأثيرات.
Full Transcript
The Inductive Effect The inductive effect of substituent's was deduced from the study of the effect of different substituent's on the degree of ionization of aliphatic acids. Thus any changes in the structure that will facilitate the removal of H ion from the acid molecule (i.e. decrease the electr...
The Inductive Effect The inductive effect of substituent's was deduced from the study of the effect of different substituent's on the degree of ionization of aliphatic acids. Thus any changes in the structure that will facilitate the removal of H ion from the acid molecule (i.e. decrease the electron density on oxygen of OH group of the acid RCO-O-H ). Thus, when one of the hydrogen atoms of methyl group of acetic acid H—CH2COOH was replaced by a methyl group i.e. CH3-CH2COOH ( propionic acid ) the degree of ionization would be decreased (i.e. acetic acid is stronger than propionic acid ). Whereas when this hydrogen atom from the methyl group of acetic acid was replaced by a chlorine atom Cl-CH2COOH ( chloroacetic acid ) the degree of ionization increased (i.e. chloroacetic acid is stronger than acetic acid ) This means that, the degree of ionization of an organic acid depends on the electron density on oxygen of OH group in the carboxyl group -COOH and its appears that the methyl group ( CH3- ) and chlorine atom ( Cl ) are operating in a different manner. [ methyl group increase the electron density on oxygen atom and therefore decrease the removal of H atom as H+ , while chlorine atom ( Cl ) decrease the electron density on oxygen atom, therefore facilitate the removal of H atom as H+.This process can take place by assuming a displacement of the electron clouds in the sigma bond towards the oxygen atom or away from it, this displacement in the sigma bond is known as the inductive displacement. O O CH3 CH2 C Cl CH2 C O H O H The methyl group is considered to be an electron- repelling group ( compared to H ) , while chlorine atom an electron - attracting group. Transmission of the effect of a substituents to adjacent bonds in a molecule: 1-Transmission of electron - attracting: Suppose we have a substituent atom ( or group ) X attached to a chain of carbon atoms C—C—C—C—X ( X= electron- attracting ) X will exert a stronger attraction on the negative cloud of the C—X bond ; this result in the development of a fractional positive charge ( + ) on C.This electron deficiency of C atom results in an electron displacement in the C—C bond in the direction of C. This electron displacement is slighter than that in the C—X bond, and result in the development of smaller fractional positive charge ( + ) on C and similar a fractional positive charge (+ )on C will be created. We said that X group has -I effect. 2-Transmission of electron - repelling effect Suppose that the substituent atom or group is an electron - repelling. Y—C –--C ---C ( Y electron- repelling ) Y will induce a fractional negative charge ( - ý) on C. C will be slightly electron - repelling and hence C will be acquire smaller negative charge ( - ). Similarly C will acquire very smaller fractional negative charge ( - ). Such Y said to have +I effect. Examples of atoms and groups which possess Electron- attracting (i.e. have - I effect): A) Atoms which are more electronegative than carbon : i)-Halogens in alkyl halides R—X ( X= F,Cl, Br, I ) ii)A part of substituent group such as : O in alcohols ( R—OH ) and ethers ( R—O—R ) N in amines RNH2 , R 2NH , R3N Note that the order of -I effect for halogens is F Cl Br I. B ) Groups containing electronically deficient atoms : The carbonyl group is strongly polarized because the displacement towards O ( more electronegative than C ) C=O carbonyl C N cyanide -N+=O nitro Groups which possess electron- repelling ( i.e. have + I effect ) The most important atoms or groups in this class are those which have a negative charge on the atom attached to the carbon chain e.g. The ethoxide ion CH3 –CH2—O - Relative order of + I effect on negatively charged atoms C—NR - C—O - Inductive effect of alkyl groups: Alkyl groups have + I effect and the relative magnitude of their +I effect in the following order: CH3 CH3CH2 CH3CH2CH2 CH3CH CH3CH2CH2CH2 CH3 CH3 CH3 CH CH 2 CH3 C CH3 CH3 Effect of electron- attracting substituent's on acid strength : a- Effect of increasing the number of electron- attraction Cl3C—COOH Cl2HC—COOH ClCH2—COOH The combined effect of two or more electron- attracting substituent's will be greater than of one only substituent. b) Effect of changing the nature of the electron- attracting substituent: F-CH2—COOH Cl-CH2—COOH Br-CH2—COOH I-CH2—COOH fluroacetic chloroacetic bromoacetic iodoacetic acid acid acid acid c) Effect of changing the position of the substituent: CH3CHý2CH CO2H CH3CH CH2CO2H CH2CH2 CH2CO2H Cl Cl Cl -chlorobutyric acid -chlorobutyric acid -chlorobutyric acid The inductive effects of the different groups are summarized Groups with -I effect Groups with + I effect -O+R 2 -COOR -OR -N- R --COO - -N- H3 --COOH -SH -O- -N+R3 -F -SR -CH3 -NO2 -Cl -CH=CH2 -CH2R -CN -Br -CR=CR2 -CHR2 -COOH -I -CCH -CR3 ******************************************************** O+ R2 --NR3 --NO2 -F --OR --NR2 -F -Cl - Br - I HALOGEN DERIVATIVES OF ALKANES ( a ) Monohalogenated compounds: Alkyl halides ( R-X ) Monohalogenated compounds are named alkyl halides where they have a general molecular formula C2 H2n+1 X. They are classified as primary, secondary and tertiary alkyl halides depending on the type of the carbon atom in which the halogen is attached to it. e.g. : CH3Cl C H 3 CH 2Br C H 3 CH CH3 Cl methylchloride ethyl chloride isopropyl chloride (chloro methane) (bromo ethane) (2- bromopropane) CH3 CH3 CH3 CH CH2 Br CH3 C CH 3 Cl isopropyl bromide ter.butyl bromide Isomerism in alkyl halides: 1- Position isomerism: compounds having the same molecular formula but differ in the position of the halogen atom e.g. C4H9Br can be: CH3CH2CH2CH2Br CH3CH CH 2CH3 Br 1-bromobutane 2-bromobutane 2- Chain isomerism: depends on the type of the carbon chain, straight or branched e.g. : CH3CH CH 2Br CH3CH2CH2CH2Br CH3 iso-butylbromide n-butylbromide 3-Optical isomerism: present in alkyl halides of asymmetrical carbon atom( atom which is attached to four different atoms or groups ). Such compounds are optically active e.g. 2- chlorobutane which has two isomeric forms ( D) and L. CH3 CH3 H Cl Cl H CH2CH3 CH2CH3 (D) -form (L)-form D= Dextrorotatory L= Levorotatory Methods of preparation: 1- From alcohols: by the action of hydrogen halides (HX) ,thionyl chloride ( SOCl2 ) or phosphorous pentachloride ( PCl5): CH3CH2-OH + HCl CH3CH2Cl + H2O ethanol ethyl chloride CH3(OH)CHCH3 + SOCL2 CH3(Cl)CHCH3 + SO2 + HCl isopropanol thionyl chloride isopropyl chloride CH3-OH + PCl5 CH3Cl + POCl3 + HCl phosphorous oxychloride 2- From alkenes: by addition of hydrogen halides (HX),where the addition takes place according to Markowni-kov's rule HBr CH3 CH=CH 2 CH3 CH CH 3 Br propene iso-propylbromide ( as mentioned before: see alkenes) 3- From alkanes: by the direct halogenation in presence of indirect sun light: hv CH4 + Cl 2 CH3Cl + HCl methane methyl chloride ( see reactions of alkanes ) Chemical reactions: The major reactions of alkyl halides proceed by substitution or by elimination ( ß- Elimination ). The nucleophilic substitution reactions can be generally represented as follow: - - - - L Nu + C X Nu C Nu C +X nucleophile leaving group The nucleopohile can be negatively charged as HO-, CN-,.. or neutral such as NH3, H2O,. … SN2 Mechanism: The hydrolysis of alkyl halides ( R-X ) using different concentrations of alkali [OH-] were found to give the following rate law: For primary halides ( RCH2X ) the rate law was found to be: Rate= K2[RX][OH-], i.e 2nd order reaction For tertiary halides: Rate= K1[RX], i.e 1st order reaction This means the reaction is independent on the concentration of alkali [OH-] as in case of ( CH3)3C-X. For secondary halides: Rate = K1[RX] + K2[RX][OH-]. ( mixed orders ). (a) Kinetic Measurements: The observed rate law for 1º-halides[ rate=k2[RX][OH-] suggest a single step process in which OH- attacked the positively polarized carbon C-X bond and passes through transition state (T.S ) in which O-C bond is partially formed and C-X bond is partially broken, the broken-down to the product. - - H O + R X H O R X HO R + X T.S The mechanism is single step mechanism where the two molecular species OH- and R-X undergo covalence charge in the rate determining step. This mechanism has been designed as SN2 mechanism. (b) Stereochemical evidence: R - - OH front side HO C X attack backside R' H attack The front side attack leads to the formation of a product with the same configuration ( i.e retention of configuration ) but if the attack from opposite direction, it will lead to inversion of configuration. R - R OH C X C OH front side R' R' H attack H retention of configuration R R - HO C X HO C R' backside R' H H attack inversion of configuration If the reaction is single step, the nucleophile will come from backside leading to inversion of configuration i.e SN2 proceeds with inversion of configuration. The steric course of SN2 reaction: The sterochemical studies of nucleophillic substitution reactions involving optically active secondary alkyl halide under experimental conditions of SN2 mechanism have shown that the product is optically active with opposite configuration to the starting materials. This means SN2 reaction is sterospecific accompanied by inversion of configuration. The inversion of configuration leads to the theory of backside attack, which is expected for single step reaction. The nucleophile attacks the side of reactant from the backside, and the reactants passes through transition state (T.S) is which Sp3 hybridized carbon become temporally Sp2 hybridized at T.S complete bond formation with nucleophile leads to optically active products having oppositely configuration. Sp3 R R Sp2 R * Nu: + C X Nu C* X Nu C R R R H H H optically active optically active with opposite conf. SN1 mechanism: Hydrolysis of tertiary alkyl halide (as tert. Butyl chloride) in aqueous alkali using different concentrations of alkali, the rate of this reaction was depend on the concentration of alkyl halide [RX] and independent on the concentration of alkali [OH]. R R R C X slow + X R C R R R R R H2O R C R C OH2 fast R C OH fast -H R R R The steric course of SN1 reaction can also explained on the bases of formation intermediate carbonium ion (C+) however the exact course of the reaction varies according to “life time of the carbonium ion”. If the carbonium ion is “long lived” so it will have the chance to become in planner Sp3 hybridization and will be surrounded by symmetrical solvent environment leading to the attack from each sides in the fast step. In such case if the starting material is optically active the resulting alcohol will be a racemic mixture. R front side C OH s R s R R H s s H2O retention 50% C X C+ - s R s R H H R optically active planar back side tertiary halides C+ in T.S HO C (sp3) R H inversion 50 % the above representation shows 100 % racemization which in fact very rare; the usual course of SN2 reaction is partial racemization and certain percentage of inversion this can be explained by the theory that the carbonium ion usually do not has an enough life time to become completely planner configuration with symmetrical solvent enviroment. When the carbonium ion is short-lived, it will react with nucleophile before the leaving group has departed far enough, this group “shields” the front side and hence preferential attacks by nucleophile will occurs from backside leading to partial racemization. R front side C OH R s R R s H H2O retention 50% C X slow s X - C+ R s s H R H R optically active C+ with unsymmetrical back side tertiary halides enviroment HO C R H inversion 50 % the inversion is larger than the retention and by increasing the life time the racemization inceases. Structure and Reactivity Relationship in both SN1, SN2 1- effect of alkyl substitution at the site of reaction (α C): a) upon SN2 reactivity: CH3 CH3 CH3 H3C X H2C X H3C C X H3C C X H CH3 rate 154 1 0.0077 0.0006 where the numbers are the relative rate of reaction of acetone SN2 reaction I + R Br R I + Br i.e the reactivity of CH3 CH3 CH3 H3C X > H2C X > H3C C X > H3C C X H i.e CH3 2 SN reaction dercease in this direction this can be explained in the term of +I effect for CH3 groups and as well as the steric hindernce to approach the nucleophile from the backside. R C X R H Nu: the inductive effect in this case (+I effect) will decrease the positively polarization (δ+) on αC and make it less susceptible by the nucleophile attack. The reason belived to be extremely important is the steric factor; as the groups at αC increased, the reactivity will decrease due to the increasing of steric hindernce to the approach of the nucleophile from backside of αC. b) Upon SN1 reactivity: the rate of formlysis (reaction with anhydrous formic acid) of some alkyl halides found as follow: O O R Br + O C H R O C H + HBr at 100C H CH3 CH3 CH3 H3C X H2C X H3C C X H3C C X H CH3 rate 0.28 1 261 = 108 This means the introducing of α-alkyl substitutent increases SN1 and decrease SN2 reactivity. This can be explained in the term of steric crowding at the back side at α carbon will increases and reaches maximum in the 3° halides. Upon ionization the bond angle changes from 109° (Sp3) to ~ 120° (Sp2) and this accompanied by relieve of steric crowding which leads to facilitate the ionization to carbocation (C+). Note: In tertiary butyl carbonium ion, the hyber conjugative stabilization would be with 9 αC-H (i.e max.) 2- effect of alkyl substitution at the position further the reaction site: a) upon SN2 reactivity: The reaction of ethoxide C2H5O- with some alkyl halides (R-X) in ethanol at 55 °C, can be represented as follow:- EtO + R X R O Et + Br the relative rate of reaction for some derivatives was illustrated … CH3 CH3CH2 X CH2CH2 X CH3(CH2)3 X CH3(CH2)4 X rate 1 0.31 0.23 0.21 this means the introducing of alkyl groups at position away from αC has only little effect upon the rate of SN2 because the free rotation around C-C σ bond which make the molecule take a configuration with less steric hindernce. H H C H X C H H If the branching in β-position as in case of (CH3)3C-CH2-X it will leads to inhibition in SN2 reactivity in spite of it is a primary halides, this is due to the large bulk of alkyl groups and consequently increasing the steric hindrance to the back side attack by nucleophilies. Therefore the compound (CH3)3C-CH2-X which is primary halide it does not react by SN2 mechanism, it is thus characterized by diminished reactivity of both SN1, SN2 reactions. Upon SN1 reactivity: Alkyl substitution away from the reaction side is also expected to bring about small variation in the rate of SN1 reaction. Excessive branching in β-C leads however, to large changes in the rate of SN1 reaction. CH3 Me CH3 H3C C CH3 Me C Me Me H3C C Cl CH3CH2 C Cl Me C C Cl Me CH3 CH3 Me C Me Me rate 1 7 40 The relative rate which is stated below for the solvolysis reaction with 80% ethanol at 25 °C, the increased rate of solvolysis in spite of decreasing the hyberconjugation (αC-H) in the carbocation can be attributed to the increasing of back strain as we go from left to right which leads to steric acceleration of ionization. Effect of nucleophiles on the reactivity of SN1 and SN2 mechanisms. The nucleophils can be classified into: a- strong nucleophiles such as species with negatively charge as; -halides nucleophiles; Br-, I-, Cl- -alkoxide and hydroxides; RO-, HO- - cyanides and azides ; CN-, N3- -mercaptants ; RS- b- moderate nucleophiles such as: -negatively charged anion; RCOO- -neutral molecules with atoms having unpaired electron; RSH, R2S, NH3, RNH2 c- weak nucleophiles as water and alcohol; H2O, ROH Effect of the polarity and solvation ability of medium of the reactivity of SN1 and SN2 mechanisms. The solvents can be classified into:- a- protic solvents: which are H-bond donors and they usually contain hydroxyl group as in case of water, alcohols and acids; H2O, ROH, RCOOH. b- Aprotic solvents: They are not H-bond donors and they divided into: i-polar: such as: H2O, ROH ii-non –polar: such as: ROR, CH2Cl2 and C6H12. Increasing of solvent polarity will:- a- enhance the rate in which the transition state (T.S) require more solvation than the reactants. b-it will retard reactions in which T.S requires less solvation than the reactants. For example: in SN1 mechanism: R X R X R + X R= t.butyl T.S This reaction is strongly accelerated as the medium is rendered more polar (strongly polar) because there is changes in the magnitude of charges on going from reactants to T.S. in SN2 mechanism: HO + R X HO R X HO R + X reactants require T.S more solvationm dispersal of charges requires less solvation this reaction is slightly retarded as the polarity of the medium increased slightly because there is no change in the magnitude of the charge. Nucleophilicity in polar aprotic solvents: These solvents are effective in solvating the cations but not effective in solvating the anions. So for the halides ions, their relative nucleophilicity is parallel with their relative basicities as follow: F- > Cl- > Br- > I- (the nucleophilicity order) Nucleophilicity in polar protic solvents: In this type of solvents, the H-bonding of solvent molecules will play a part in the solvation of the anions. So far the halides ions, the relative nucleophilicity will be in contrast with the basicity and the order of their nucleophilicity will be as follow: F- < Cl- < Br- < I- (the nucleophilicity order) generally the nucleophilicity of atoms in the periodic table will decrease from left to right: CH-3 > NH-2 > OH- > F- period 2 Ph-2 > SH- > Cl- period 3 Effect of leaving groups: The leaving groups will have in both T.S of SN1 and SN2 mechanism a partial negative charge and at the end of reaction they leave the reactant molecule as an anion, so the best leaving are those the stable one (usually the conjugate bases of strong acids). For example, the halide ions, the best leaving group is iodide ion I-. Elimination Reactions Elimination of (HX) from alkyl halide leads to the formation of the corresponding olefins. The overall the reaction can be represented as follow: H R C C X R C CH2 + HX H2 H H B-elimination Investigation of these types of reaction has shown that, the alkyl halides can undergo elimination of HX by two mechanisms E1 and E2. a) Bimolecular mechanism E1: When a primary alkyl halide is treated with an alkali such as aq. NaOH, alc. NaOH or alcoholic solution of RONa the corresponding alcohol or ether (due to substitution reaction ) will be produced in addition to the corresponding olefin (due to to elimination reaction). The percentage of either reaction depends upon the structure of primary halide as well as the experimental condition (nature of reagent, nature of solvent, temperature and pressure). OH H R C C X R CH2CH2 OH + X SN2 H2 H H R C C X R C CH2 + X + H2O E2 H2 H H OH the rate of olefin formation in such case depends on: R α [R-X][base] i.e 2nd order reaction This kinetic observation means that the reaction proceeds via a single step mechanism. When β-phenyl ethyl bromide was treated with sodium ethoxide C2H5ONa in ethyl alcohol which rich in deuterium (C2H5OD, the unchanged bromide which isolated at the end of the reaction was found to contain no C β -D bond. H2 H C C Br C CH2 H2 EtONa EtOD The lack of deuteration at Cβ indicate that no carbanion center has been created at this carbon: Ph C C X H2 H2 this experiment support the single step mechanism which can picture as-follow:- B B H H R C C X R C C X R C CH2 + X + BH H2 H2 H H T. S E2 The reactants come together to form single T.S in which B-Hβ and Л-bond are partially formed and H-Cβ , Cα-X is partially broken [i.e concerted mechanism]. This mechanism called E2 mechanism. b)Unimolecular (E1) mechanism: In the solvolysis of tert. Butyl halides; eg. ter- butyl halides in water a certain percentage of isobutene is formed beside ter-butyl alcohol. The rate of olefin formation R α [R-X] only. This lead to the suggestion that elimination and substitution have a common first step namely the slow rate determining step of ionization to carbon cation C+. once formed this carboncation will have two possibilities:- 1-attack the C+ center which lead to the substitution (SN1). 1- Elimination of β-H as proton which lead to the formation of olefin (E1). CH3 H2O at SN1 H3C C OH C+ CH3 CH3 CH3 slow H3C C X H3C C R.D.S CH3 CH3 CH3 H2O at H2C C CH3 E1 B H This mechanism of elimination has been called unimolecular (E1 mechanism). The mechanism of substitution and elimination for different classes of alkyl halides. Type of halide SN E SN2 E2 Primary halides Secondary halide SN1 , SN2 E2 , E1 Tertiary halides SN1 E1 QUESTION: 1- if we want to obtain isobutene from 3º-butyl chloride, which reaction condition we must use: a) 3º--halides + H2O (boil) or conc. NaOH. b) 3º--halides + alc. EtONa in boiling EtOH. Competition between substitution and elimination reaction (E / SN ratio). E2 / SN2 ratio: E2/SN2 ratio is depending on the following factors : 1- Basicity of the added reagent. 2- Polarity of the medium. 3- Temperature. 4- Structure of the substrate R-X. 1- Basicity of the added reagent. B E2 2 H SN C X Nu By increasing the basicity the greater of E2 / SN2 ratio. Since the E2 reaction involve the added nucleophile acting as the base, the greater the basicity of this nucleophile, the greater of E2 / SN2. For a given R-X, the E2 / SN2 ratio will be expected to be greater with alc. EtONa than with alc. KOH (EtONa more basic than KOH). In some cases it is desired to increase the ratio of olefin, this can be done by increasing the basicity of nucleophile or by retarding the competing of SN2 reaction through using of tertiary base such as:- H3C CH3 N H3C N CH3 In both cases SN2 attack will be greatly retarded by steric crowding involving the alkyl groups at the front side of nucleophile center which called [ F-Strain]. 2- Effect of medium. The use of alcoholic KOH leads to higher ratio of olefin than in case of using aqueous KOH, two reason can be suggested:- a) the first in the equilibrium between OH- and C2H5OH to form C2H5O- OH + C2H5OH H2O + C2H5O i.e alc. KOH contain beside OH- some C2H5O- ions and hence gives a greater E2 / SN2 ratio. b) the second reason is the distribution of the charges. C X Nu T.S for SN2 C X Nu dispersal of charged reactants B H B H T.S for E2 C C X C C X greater dispersal If a reaction passes through a T. S. in which the charges on reactant is dispersed, such reaction will be retarded by increasing the polarity of the medium. (i.e the greater of dispersal the greater of retardation), so the increasing of medium polarity, the retardation of E2 reaction. In order to increase E2 / SN2 ratio we should use less polar solvent (i.e alc. KOH is better reagent for preparation of olefin than aq. KOH). 3- Temperature. Increasing the temperature increases E2 / SN2 ratio. 4- Structure of alkyl groups (R). The greater the branching at α-carbon, the greater of E2 / SN2 ratio. CH3 H3C CH2X CH3CH2CH2X CH3CH X H3C C X CH3 CH3 E2 / SN2 ratio increases a- as we go from left to right, SN2 is retarded by the bulk of groups attached to α - C. b- E2 reaction is enhanced by hyberconjugation of the α-alkyl groups. E1 / SN1 ratio. The most important factor in determining of E1 / SN1ratio is the structure of the alkyl groups in R-X and it has been observed that the ratio increases with increasing the branching in Cβ. CH3 CH3 80 % EtOH H3C C X SN1 + CH2 C CH3 25 C CH3 84 % CH3 CH2CH3 CH3 CHCH3 1 + H3C C C X SN H3C C C CH3 CH2CH3 CH3 CH2CH3 10 % 90 % The increasing of the branching at β-carbon leads to steric crowding between the three alkyl groups attached to β-carbon (β-strain). Upon ionization to C+ this back strain is relieved, however, if SN reaction occurs, this back strain is reintroduced, while if elimination reaction occurs, the back strain remain relieved. R Nu C Nu SN1 R R R CH2R X B-strain is reintroduced C X C R R CH2R CH2R R there is B-strain B-strain is relieved H E1 C C R H R 120 Orientation in Elimination Reactions: Saytzeff’ rule; State that “ in dehydrohalogenation of alkyl halides, the predominant product is the more highly substituted olefin.” alc. KOH H3C CH CH CH3 + H2C C CH2 CH3 H3C CH CH2 CH3 H Cl 80 % 20 % Where 1-butene consider monosubstituted olefin, while 2-butene is disubstituted olefin. This can be interpreted on term of greater hyberconjugation, which lower the energy of intermediate and so facilitate the formation of the corresponding olefin. Some examples for the reactions of alkyl halides with some reagents: 1-Hydrolysis: Action of aqueous alkali give the corresponding alcohol: R-X + NaOH R-OH + NaX CH3Br + NaOH CH3OH + NaCl methyl bromide methanol 2-Action of alcoholic ammonia give amines: R-X + NH3 R-NH2 + HX CH3CH2Cl + NH3 CH3CH2NH2 + HCl ethyl chloride ethyl amine 3-Action of alcoholic pot. cyanide: give alkyl cyanide: R-X + KCN R-CN + KX R-CN + 2H2O R-CO2H + NH3 CH3Cl + KCN CH3CN + KCl methyl chloride methyl cyanide CH3CN + 2H2O CH3CO2H + NH3 methyl cyanide acetic acid CH3CH2Cl + KCN CH3CH2CN +H2O CH3CH2CO2H + NH3 ethyl chloride ethyl cyanide propionic acid 4-Action of silver salts give esters: R-CO2Ag + R'X RCO2R' + AgX CH3CO2Ag + CH3Cl CH3CO2CH3 + AgCl silver acetate methyl chloride methyl acetate 5-Action of sodium alkoxide: give ethers: R-ONa + R'X R-O-R' + NaX CH3CH2ONa + CH3CH2Cl CH3CH2OCH2CH3 sod.ethoxide ethyl chloride diethyl ether 6-Action of sodium sulfide: give thio ether 2R-X + Na2S R-S-R + NaX CH3Cl + Na2S CH3-S-CH3 + NaCl dimethyl thio ether 7-Formation of Grignard's reagent: Alkyl halides react with magnesium in dry ether as a solvent to give Grignard's reagent. R-X + Mg / ether R-Mg X CH3CH2I + Mg / ether CH3CH2MgI ethyl iodide ethyl magnesium iodide 8-Reduction: With H2 /Pt give alkanes: R-X + H2/ Pt R-H + HX CH3Br H2/Pt CH4 + HBr 9-Wurz reaction: reaction of alkyl halides with sodium metal to give alkanes 2R-X + 2Na R-R + 2 NaX 2 CH3Cl + 2 Na CH3-CH3 + NaCl methyl chloride ethane 10-Reaction with Grignard's reagent: give alkanes: R-X + R'MgX R-R' + MgX2 11-Action of alcoholic pot.hydroxide give alkenes: where the dehydrohalogenation proceeds according to Saytzeff's rule: alc. KOH CH3 CH CH 2CH3 CH3 CH=CH CH 3 - HCl Cl 2-chloro butane 2-butene Dihalogen derivatives of alkanes Dihalogen derivatives of alkanes have a general molecular formula CnH2nX2 and they are divided into two classes: 1- Vicinal dihalides ( vic-dihalides ) in which the two halogen atoms are attached to two adjacent carbon atoms 2- Geminal dihalides ( gem-dihalides) in which the two halogen atoms are attached to the same carbon atom. CH3 CHCl2 CH3CH2CHBr2 CH3Cl2CH3 1,1-dichloroethane 1,1-dibromopropane 2.2-dichloropropane Methods of preparation a-Vicinal-dihalides: 1-Action of halogens (X2) on alkenes: CH2=CH2 + Br2 CH2 CH2 ethylene bromide Br Br 1,2-dibromoethane 2- Action of PCl5 0n glycols: CH2 CH2 + PCl5 CH2 CH2 + POCl3 + HCl OH OH Cl Cl ethylene glycol ethylene chloride b) Geminal-dihalides: 1-Action of HX on acetylene's: HCl CH3 C C H + HCl CH3 CH=CH 2 CH3 C CH3 Cl Cl Cl 2,2-dichloropropane 2-Action of PCl5 on aldehydes and ketones: CH3CHO + PCl 5 CH3CHCl 2 + POCl 3 + HCl acetaldehyde ethylidene chloride CH3 C CH3 CH3 CO CH 3 + PCl5 Cl Cl Chemical reactions 1- Action of alcoholic KOH: both vic- and gem-dihalides give acetylene's(see preparation of acetylene's): CH3CH CH Cl Cl 1,2-dichloropropane alc. KOH CH3 C C H CH3 C CH3 - 2 HCl Cl Cl methyl acetylene 2,2-dichloropropane 2-Action of aqueous alkali: a-vic-dihalides give glycols: CH2 CH2 NaOH CH2 CH2 Cl Cl OH OH b- gem-dihalides give aldehydes or ketones depending on the position of the halogen atoms: NaOH OH -H2O CH3 CH2 CHCl2 CH3 CH2CH CH3CH2CHO OH NaOH - H2O CH3 C CH3 CH3 C CH3 CH3 CO CH 3 Cl Cl OH OH acetone (c) TRIHALOGENATED COMPOUNDS e.g. CHCl3 CHBr3 CHI3 chloroform bromoform iodoform Methods of preparation: These compounds are prepared by Haloform reaction, where alcohols which contain CH3CHOH group or aldehydes and ketones which have CH3CO group in presence of halogens(X2) and sodium hydroxide give Haloform compounds. Cl2 3 Cl2 CH3CH2OH CH3 CHO CCl3 CHO oxidation sustitution NaOH H COONa + CHCl 3 sod.formate iodoform NaOH 3 CH3 CO CH 3 CCl3 CO CH 3 CH3CO2Na + CHCl3 sod.acetate Cl2 3 Cl2 CH3CH2OH CH3 CHO CCl3 CHO oxidation sustitution NaOH H COONa + CHCl 3 sod.formate iodoform NaOH 3 CH3 CO CH 3 CCl3 CO CH 3 CH3CO2Na + CHCl3 sod.acetate Chemical reactions: We will take chloroform as an example for this type of compounds. 1-Oxidation: where chloroform when is exposed to air in presence of light it will oxidized to phosgene ( toxic material). for use chloroform as an anesthetic, it must be stored in a dark bottles which are well stoppered. Addition of about 1% ethanol converts any phosgene( poisonous) which might be formed to diethyl carbonate( harmless). Cl CHCl3 HO CCl 3 O=C + HCl Cl Cl O C2H5 O=C + 2 C2H5 OH O= C Cl O C2H5 diethyl carbonate 2-Nitration: when chloroform is treated with conc. HNO3 it gave chloropicrin. CHCl 3 + HNO 3 Cl3C NO 2 + H 2O chloropicrin 3- Hydrolysis: if chloroform is heated with conc. aqueous solution of alkali( NaOH) it will produce formic acid. HCl CHCl 3 + NaOH HCO 2Na HCO2H formic acid 4-Hofmann's carbylamine reaction: when chloroform is warmed with aniline and alcoholic KOH ,it gives phenylisocyanide. CHCl 3 + C6H5-NH2 + 3 KOH C6H5-N C + 3 KCl aniline phenylisocyanide Aromatic halogen compounds Halogen compounds can be obtained from three types of reactions: a- addition reactions b-substitution reactions c-side-chain substitution reactions [A] addition reactions: These compounds can be obtained by the addition of halogens ( Cl2, Br2) to benzene in presence of indirect sun light and absence of the catalyst. Cl Cl Cl 3 Cl2 gamexan hv Cl Cl ( hexachloro benzene) Cl [B] Substitution reactions products: 1-Halogens ( Cl2, Br2) react with benzene or alkyl benzene in presence of a catalyst such as ( low FeCl3 and in absence of sun light to give substitution products in benzene ring. temperature) Cl2 Cl + HCl FeCl3 chloro benzene CH3 Cl2 CH3 CH3 + FeCl3 Cl Cl o-chloro toluene p-chloro toluene The extend of halogenation depends on the amount of halogen used Iodine compounds: Iodination can be carried out in the presence of an oxidizing agent e.g., nitric acid mercuric oxide, ect.. the yield of the iodo-compound is usually very good. 2 C6H6 + I2 (HNO3) 2 C6H5-I +H2O 2-Sandmayer reaction: Replacement of diazonium group by -Cl or -Br is carried out by mixing the solution of freshly prepared diazonium salt with cuprous chloride or cuprous bromide ArN2X + CuX ArX + N2 ( X= Cl or Br). Gattermann reaction: Sandmayer reaction can be carried out by modification, in which the copper powder and hydrogen halide are used in place of cuprous halide. Replacement of the diazonium group by iodide does not require the use of a cuprous halide or copper, the diazonium salt and potassium iodide are simply mixed together and allowed to react. Cu / HCl Ar-Cl chloro benzene Cu /HBr ArN2 X Ar-Br bromo benzene KI Ar-I if Ar = C6 H5 - iodo benzene Replacement of the diazonium salt by fluoride -F can be carried out by using fluoroboric acid ( HBF4). C6H5N2Cl + BF4- C6H5-F fluoro benzene. Properties of Aryl halides: The aryl halides differ from alkyl halides, this is due to the resonance in aryl halides which leads to give the C-X bond some of the double bond character ( i.e. this bond become difficult to be broken ). X X X X X hybrid structure This also explain why the halogen atom in aryl halides can not be replaced by nucleophiles except under very drastic reaction conditions.- However, the presence of certain groups at certain positions of the ring markedly activates the halogen of aryl halides towards displacement. 1- with aqueous NaOH: Chlorobenzene is converted into phenol by aqueous NaOH only at temp. over 300 C. The presence of a nitro group ortho or para to the chlorine increases its reactivity so, p-chloro benzene is converted into p-chloro phenol by treatment with aqueous NaOH at 160 C.Phenol is obtained from 2,4-dichlorobenzene by treatment with hot aq. NaOH and from 2,4,6-trichlorobenzene by simple treatment with water. Cl OH aq. NaOH, 300C Cl OH Cl OH aq. NaOH, 160 C 2,4-dichlorobenzene o-dihydroxybenzene ( catechol) Cl OH H2 O, warm Cl Cl HO OH 2,4,6-trichlorobenzene 2,4,6-trihydroxybenzene 2-with aqueous NH3: Chlorobenzene reacts with ammonia in presence of a catalyst such as CuO at 200C and under pressure to give aniline. 2 NH3, CuO, 200C 2 Cl 2 NH2 + CuCl + H 2O Bromobenzene reacts with KNH2 in liquid ammonia to produce aniline. Br KNH2 NH2 liq. NH3 The mechanism of this reaction involves elemination-addition reaction through the formation of an intermediate called benzyne as follow: Br + NH3 + Br H NH2 benzyne NH2 NH2 NH2 NH2 + NH3 The benzyne intermediate can be trapped through its reaction with diene under Diels- Alder reaction, for example it reacts with furan to form Diels-Alder adduct as follow: + O benzyne O 3-with cuprous cyanide: They react with CuCN to form aryl cyanides which on hydrolysis give the corresponding acids. Cl CN CO 2H CuCN hydrolysis Chloro benzene phenyl cyanide benzoic acid 4-Wurtz- Wittig reaction: Heating of aryl halides with alkyl halides in presence of metallic sodium give alkyl benzenes [ see the preparation of alkyl benzene ]. C6H5-Br + CH3Br + 2Na C6H5-CH3 + 2NaBr bromo benzene methyl bromide toluene 5-formation of Grignard’s reagent: Aryl halides react with Mg in presence of water free ether as a solvent to give aryl magnesium halides where the reactivity of halogen in this reaction are in the following order I2 Br2 Cl2. C6H5-I + Mg/ether C6H5-MgI iodo benzene phenyl magnesium iodide Preparation of DDT. Cl Cl H2 SO 4 2 Cl + CCl 3 CHO Cl C CH conc. Cl chlorobenzene chloral Cl [ c ] Side- chain substitution compounds: This type of halogenated compounds can be prepared by 1-Halogenation: This type of halogenation ( chlorination and bromination ) favour the high temperature and light. Chlorination of toluene is carried out stepwise to give mono, di, and trihalo derivatives. CH3 CH2Cl CHCl 2 CCl 3 Cl2 Cl2 Cl2 hv hv hv benzylchloride benzalchloride benzotrichloride Halogenation of alkyl benzene proceeds in this order 3 2 1carbon atom. 2-Chloromethylation: Benzene reacts with formaldehyde and HCl in the presence of ZnCl2 as Lewis acid catalyst and in methylene chloride as a solvent to give benzyl chloride. C6H6 + CH2O /HCl C6H5CH2Cl benzyl chloride Mechanism: the mechanism of the reaction is similar to Fridel-Craft alkylation reaction. ( suggest the mechanism ). Chemical properties: The halogen in the side-chain undergoes the same reactions as those of aliphatic alkyl halides. C6H5CH2Cl + aq. NaOH C6H5CH2OH benzyl alcohol C6H5CHCl2 + aq. NaOH C6H5CHO benzal chloride benzaldehyde C6H5CCl3 + aq. NaOH C6H5CO2H benzotrichloride benzoic acid C6H5CH2Cl + alc. NH3 C6H5CH2NH2 benzyl amine C6H5CH2Cl + KCN C6H5CH2CN C6H5CH2CO2H Phenylacetic acid ALIPHATIC AMINES Aliphatic amines can be considered as derivatives from ammonia by replacing hydrogen atoms by the alkyl groups and they classified into primary, secondary and tertiary amines. Aliphatic amines can be considered as derivatives of ammonia (NH3), R-NH2 R2NH R3N primary amines secondary amines tertiary amines Nomenclature: Aliphatic amines are named according to the alkyl groups attached to the nitrogen by adding the word amine CH3-NH2 CH3NHCH3 ( CH3)3N methyl amine dimethyl amine trimethyl amine Methods of preparations: 1- Ammonolysis of alkyl NH3 NH3 a)- R X R NH3X R NH2 + NH4X NH3 NH3 CH3Cl CH3NH3Cl CH3NH2 + NH4Cl methyl chloride methyl amine RX + HX b)- R NH2 R2NH CH3Cl CH3 NH2 ( CH3 )2NH + HCl c)- R2NH R-X R3N + HX CH3Cl ( CH3 )3N + HCl (CH3)2NH trimethyl amine 2-Catalytic reduction of alkyl cyanides: H2/Ni R-C N RCH 2NH2 alkyl cyanide H2 / Ni CH3CN CH3CH2NH2 methyl cyanide ethyl amine 3-Hofmann degradation reaction: Action of bromine and sodium hydroxide on amides ( -CONH2 ) O C NH2 + Br2 + NaOH R NH2 +Na2CO3 + NaBr + H2O R CH3CONH 2 + Br2 +NaOH CH3-NH2 + Na2CO3 + NaBr +H2O acetamide methyl amine 4- Gabriel phthalimide synthesis : O O O KOH R-X NH NK N-R O O O phthalimide pot.phthalimide HCl/H2O NaOH/H2O CO2H CO2Na + RNH 3Cl R-NH2 + CO2H CO2Na phthalic acid sod.phthalate 5-Reductive amination: Aldehydes and ketones can be reduced by H2/Ni in presence of ammonia to give primary amines: H H H2/Ni C O + NH3 R C NH R CH2NH2 R aldehyde imine 1o amine H2/Ni CH3CHO + NH3 CH3CH=NH CH3CH2NH2 ethyl amine R` R` R` H2/Ni R C O + NH3 R C NH R C NH2 CH3 CH3 CH3 H2/Ni CH3 C O + NH3 CH3 C NH CH3 C NH2 acetone isopropyl amine Physical properties of amines: Like ammonia , amines are polar compounds and except ter.amines they can form intramolecular hydrogen bonding. H H H R N H N H N R R H H-bonding Therefore amines have higher boiling points than non-polar compounds of the same molecular weight, but they have lower boiling points than alcohols. Chemical reactions of amines: 1- Basicity: Aliphatic amines more basic than ammonia due to the presence of alkyl groups which have donating properties i.e increase the electron density on nitrogen atom and hence increase the basicity, therefore we expect than tertiary amines more basic than secondary amines and the later more basic than primary amines. They react rapidly with mineral acids to give the corresponding salts. CH3CH2NH2 + HCl → CH3CH2NH3+Cl- ethyl amine ethyl amine hydrochloride 2- Alkylation: Amines can be alkylated in presence of alkyl halides to give the quaternary ammonium salts as the final stage of alkylation. H R R RX RX RX R NH2 R-N-R R-N-R R-N-R X R ( see preparation of amines ). 4-Acylation: Action of acetyl chloride or acetic anhydride on amines. CH3NH2 + CH3COCl CH3NHCOCH3 + HCl methyl amine acetyl chloride N-acetyl methyl amine 3-Reaction with nitrous acid: This reaction used for differentiation between primary, secondary and tertiary amines: a-Primary amines react with nitrous acid with evolution of nitrogen gas to give alcohols. R-NH2 + HNO2 R-OH + N2 + H2O CH3CH2-NH2 + HNO2 CH3CH2OH + N2 + H2O ethyl amine ethanol b- Secondary amines give N-nitroso dialkyl amine: H N O HONO R-N-R` R R` + H2O N N O ( NaNO2/ HCl) CH3NHCH 3 CH3 N CH3 + H2O N-nitrosodimethyl amine c-Tertiary amines does not react with nitrous acid. Aromatic amines Aromatic amines contain an amino group ( NH2 ) which can be attached directly to benzene nucleus as in case of aniline C6H5-NH2 or attached to the side chain as in case of benzyl amine C6H5CH2NH2.The latter amine is very similar to those of aliphatic amines in their preparations and reactions. Benzyl amine C6H5CH2NH2 Preparation: 1-Reduction of phenyl cyanide. C6H5-CN + C2H5-OH / Na C6H5-CH2-NH2 2-Heating of benzyl chloride with ammonia under pressure. C6H5-CH2-Cl + NH3 C6H5-CH2-NH2 3-Hofmann degradation of phenyl acetamide C6H5-CH2CO-NH2 + Br2 / KOH C6H5-CH2-NH2 4-Reaction with nitrous acid forms benzyl alcohol. C6H5-CH2-NH2 + HNO2 C6H5-CH2-OH+N2+H2O Chemical properties: Benzyl amine in its reactions is similar to the reactions of aliphatic amines. Aryl amines Ar-NH2 Methods of preparation: 1-Reduction of nitro compounds C6H5-NO2 + H2/Ni C6H5-NH2 nitro benzene 2-potassium hydroxide on benzamide (Hofmann degradation): C6H5-CONH2 + Br2/ KOH C6H5-NH2 Mechanism of Hofmann degradation(Hofmann rearrangement) O O Br / KOH Ar Ar C NHBr C NH2 (HOBr ) O O OH Ar C NHBr Ar C N Br -H + O Ar C N Br Ar N C O isocyanate H2O OH Ar NH C Ar-NH2 Ar N C O -CO2 O isocyanate Also aryl amines can be obtained from carboxylic acids or their derivatives through Curtius rearrangement: PCl5 NaN3 CO2H COCl CON3 O H2O C N N N N C O NH2 phenylisocyanate Lossen Rearrangement: Action of a base on the ester of hydroxamic acid which gives amines through the formation of isocyanate as an intermediate: B Ph CONHOCOR Ph C N OCOR Ph N C O -H + O H2O Ph NH2 + CO2 3-Ammonolysis of halo benzene. C6H5-Cl + 2NH3/ Cu2O / P C6H5-NH2 chloro benzene 4-Action of NH3 on phenol. C6H5-OH + NH3/ ZnCl2 / P , C6H5-NH2 5-From sulphonic acid C6H5-SO3Na + NaNH2, fusion C6H5-NH2 + Na2SO3 sod.benzenesulphonate sodamide Properties of Aromatic amines: Primary aromatic amines are liquid compounds and have characteristic odour and they become darkens when they expose to air due to the oxidation. 1-Basicity: The basicity of aniline is less than of aliphatic amines due to the fact that the unshared electron pair on nitrogen atom are less available owing to their introducing with - cloud of the benzene ring. NH2 NH2 NH2 NH2 NH2 hybrid structure The basicity increases by introducing an electron releasing group And decreases by introducing an electron withdrawing group. NH2 NH2 NH2 OCH3 H NO2 p-anisidine aniline p-nitroaniline The methoxyl group OCH3 has +M effect so it increases the electron density on the amino group NH2, therefore the basicity increases while the nitro group NO2 has -I and -M effect so it decreases the electron density on the amino group therefore the basicity decreases. Aniline reacts with mineral acids such as HCl to give aniline hydrochloride C6H5-NH2 + HCl C6H5-NH3+Cl- 2-Alkylation: Aniline reacts with alkyl halides giving secondary and tertiary amines. CH3 CH3Cl CH3Cl NH2 NH Cl N(CH3)2Cl NaOH NaOH N(CH3)2 NHCH3 N-methyl aniline N,N-dimethyl aniline Hofmann- Martius rearrangement: Alkyl aniline also its quaternary salts in their hydrochloride salts under high temperature, the alkyl groups migrate to the para position and when the p-position is occupied they migrate to the ortho position. + - N(CH3)3 Cl N(CH3)2 HCl NHCH3HCl NH2.HCl CH3 H3C CH3 CH3 CH3 CH3 3-Acylation: Aniline reacts with acetyl chloride or acetic anhydride in presence of a base such as sodium hydroxide to give acetanilide and this reaction known as Schotten-Baumann reaction C6H5-NH2 + CH3COCl / NaOH C6H5-NHCOCH3 + HCl acetanilide C6H5-NH2 + (CH3CO)2O / NaOH C6H5-NHCOCH3 +CH3CO2H p-Toluidine can be also acylated by acetic anhydride to give p-aceto-toluidine. NH2 NHCOCH3 (CH3CO)2O + CH3CO2H CH3 CH3 p-toluidine p- aceto-toluidine Secondary aromatic amines such as N-methylaniline can be also acylated to gine N-acetyl methylaniline but tertiary amines can not be acylated. NHCH3 H3C NCOCH3 (CH3CO)2O + CH3CO2H N-methylaniline N-acetyl methylaniline ( CH3CO)2O C6H5 N (CH3 )2 no reaction dimethylaniline Also it reacts with benzoyl chloride under the same reaction conditions to give benzanilide. C6H5-NH2 + C6H5COCl / NaOH C6H5-NHCOC5H5 + HCl Benzanilide 4- Reaction with arylsulphonyl chlorides: NH2 H NSO2 C6H5 C6H5SO2Cl +HCl benzene sulphonylchloride aniline N-phenylbenzenesulphonamide base C6H5NH2 C6H5NH SO2 CH3 H3C SO2Cl N-phenyl-p-tolunesulphonamide 5-Reaction with nitrous acid: ( diff. between 1, 2, 3 aromatic amines). A-ِprimary amines: Primary aromatic amines react with nitrous acid to give diazonium salts which are stable in contrast with aliphatic amines which decompose in solution to give alcohols. The stability of these salts attributed to presence of benzene ring which stabilized these salts by resonance. C6H5-NH2 + NaNO2 / HCl C6H5-N2Cl + 2H2O benzene diazonium chloride + + - + - + - N N N N N N N N + + + B- secondary amines: give N- nitroso amines. NO NaNO2 / HCl NHCH3 NCH3 + H2O N-methyl aniline N-methyl -N-nitroso aniline C-tertiary amines: give p- nitroso derivatives. NaNO2 / HCl N(CH3)2 ON N(CH3)2 N,N-dimethyl aniline p-nitroso-N,N-dimethyl aniline 5-Condensation with aldehydes and ketones: aniline condenses with aldehydes and ketones giving Schiff bases. C6H5-NH2 + C6H5-CHO C6H5-N=CHC6H5 + H2O benzaldehyde benzal aniline [B] Reactions involving the aromatic nucleus: 7-Oxidation: Aniline can be oxidized by sodium hybochlrite to give p-amino phenol. NaOCl NH2 HO NH2 p-hydroxy aniline or p- amino phenol 8-Bromination: Treatment of aniline with bromine solution gives 2,4,6-tribromo aniline. NH2 NH2 3 Br2 Br Br Br The formation of tribromide is due to the amino group ( NH2 ) which activates the o- and p- positions. In order to introduce one bromine atom, the activating effect of the amino group must be lowered by acylation. NH2 NHCOCH 3 NHCOCH 3 NHCOCH 3 CH3COCl Br2 Br + acetanilide Br NaOH NaOH NH2 NH2 Br Br 9-Nitration: Nitration of aniline can not be carried out using nitric acid alone due to its ease of oxidation, therefore the nitration of aniline can be carried out in concentrated sulfuric acid giving m- nitro aniline due to the formation of NH3+ which is m- directing group. NH2 NH3.HSO 4 NH3.HSO 4 NH2 H.HSO 4 HNO3 NaOH NO2 NO2 In order to prepare o- and p- nitroaniline, the acylation of aniline must be carried out firstly. NH2 NHCOCH 3 NHCOCH 3 NHCOCH 3 CH3COCl HNO 3 NO2 + acetanilide NO2 70%H2SO4 70 % H2SO 4 NH2 NH2 NO2 NO2 10-Sulphonation: Heating of aniline with concentrated sulfuric acid at 180 C gives sulphanilic acid. NH2 NH2 H2 SO 4 + H2 O 180 C SO 3 H Hinsberg method for differentiation between primary, secondary and tertiary aromatic amines: Aniline, methylaniline and dimethyaniline react with p-toluene sulphonylchloride (Tos-Cl); aniline forms sulphonamide which soluble in KOH solution while methylaniline forms sulphonamine insoluble in KOH solution but dimethylaniline does not react with Tos-Cl. - + NH2 NHSO2 Tos N SO2Tos K Tos-Cl KOH soluble NO2 NHCH3 H3C NSO2 Tos Tos-Cl KOH insoluble (no NH ) N(CH3)2 Tos-Cl no reaction Diazonium Salts Diazonium salts are important materials in preparation of various organic compounds. They are difficult to be isolated in solid form therefore they are prepared in solution. Preparations: Treatment of an aromatic amine with sodium nitrite/ HCl ( nitrous acid ) on cold. Ar-NH2 + NaNO2/ HCl Ar-N2+ Cl- for example NaNO2 / HCl NH2 N N Cl benzene diazonium chloride Chemical reactions: ( a ): Replacement reactions: 1-Replacement by hydroxyl group: when benzene diazonium chloride is boiled in water it gives phenol. C6H5-N2Cl + H2O boil C6H5-OH + N2 + HCl 2-Replacement by hydrogen: heating of the diazonium salt with methanol or with H3PO2 afforded benzene. C6H5-N2Cl + CH3OH boil C6H6 + N2 + HCl + CH2O 3-Replacement by halogen: i- Sandmayer’s reaction: Replacement of diazonium group by -Cl or -Br is carried out by mixing the solution of freshly prepared diazonium salt with cuprous chloride or cuprous bromide ArN2X + CuX ArX + N2 ( X= Cl or Br). ii-Gattermann reaction: Sandmeyer reaction can be carried out by modification, in which the copper powder and hydrogen halide are used in place of cuprous halide. Replacement of the diazonium group by iodide does not require the use of a cuprous halide or copper, the diazonium salt and potassium iodide are simply mixed together and allowed to react. Cu / HCl Ar-Cl chloro benzene Cu /HBr ArN2 X Ar-Br bromo benzene KI Ar-I if Ar = C6 H5 - iodo benzene Replacement of the diazonium salt by fluoride F- can be carried out by using fluoroboric acid ( HBF4). C6H5N2Cl + BF4- C6H5-F fluoro benzene 4-Replacing by nitro group: They react with sodium nitrite inpresence of copper to give nitrobenzene. C6H5-N2Cl + NaNO2/Cu C6H5-NO2 nitrobenzene 5- Replacing by cyano group: This reaction is carried out using cuprous cyanide in presence of potassium cyanide and powder cupper. C6H5-N2Cl + K3Cu(CN)6 C6H5-CN phenyl cyanide This phenyl cyanide on hydrolysis gives corresponding acid. C6H5-CN + 2H2O/ base C6H5-CO2H + NH3 Benzoic acid 6- Replacing by an aryl group ( Gomberg reaction): N2Cl NaOH biphenyl This reaction proceeds through free radical mechanism: C6H5-N2Cl + OH- C6H5 -N= N-OH C6H5 -N= N-OH C6H5. + N2 + OH. C6H5. + C6H6 C6H5¯ C6H5 + H2O ( b ) Coupling reactions: The diazonium salt ion C6H5N2- is a weak electrophile, therefore it attacks the activated benzene ring gives rise to highly colored compounds known as Azo-dye. Coupling takes place at the p- position , when this p- position is blocked, the coupling takes place at the o- position. NaOH N N Cl + OH N=N ONa benzene diazonium chloride p- hydroxy azo benzene NaOH N N Cl + NH2 N=N NH2 benzene diazonium chloride p- amino azo benzene N=N OH ONa N N Cl + NaOH benzene diazonium chloride ALCOHOLS Alcohols are compounds which contain one hydroxyl group called monohydric alcohols, while those of two hydroxyl groups called dihydric alcohold. Alcohols of three hydroxyl groups called trihydric alcohols. Monohydric alcohols : The monohydric alcohols have a general formula R-OH where R is an alkyl group , these alcohols have a general molecular formula Cn H2n+2 O and the are divided into three classes: a- Primary alcohols R-CH2-OH b- Secondary alcohols (R)2-CH-OH c- Tertiary alcohols (R)3-C-OH Nomenclature of alcohols 1-IUPAC system: alcohols have a name derived from the corresponding alkane by changing " e " of " ane " to " ol" and alcohols of more than two carbon atoms must be numbered starting from carbon atom which is nearest to the hydroxyl group, where the alcohol take the number of carbon atom in which the hydroxyl group is attached to it. 2-Alkyl system: in which the word alcohol is coupled with the name of alkyl group. 3-Carbinol system: where the simplest alcohol ( CH3OH ) called carbinol and the other alcohols can be considered as a derivatives from carbinol CH3OH CH 3CH2OH CH 3CH2CH2OH methnaol ethanol n-propanol( 1-propanol) methyl alc. ethyl alcohol n-propyl alcohol carbinol methyl carbinol ethyl carbinol CH3 CH3 CHCH 3 CH3 C CH3 CH3CH2CH2CH2OH OH OH n-butanol(1-butanol) isopropanol(2-propanol) ter.butanol isopropyl alcohol ter.butyl alcohol n-butyl alcohol dimethyl carbinol n-propyl carbinol trimethyl carbinol CH3-CH-CH2-CH3 CH3-CH-CH2-OH OH CH3 sec.butanol( 2-butanol) iso-butanol sec.butyl alcohol iso-butyl alcohol ethyl methyl carbinol isopropyl carninol Methods of preparation 1-Hydrolysis of alkyl halides with aqueous alkali ( NaOH, KOH ) : R-X + NaOH R-OH + NaX e.g.: CH3CH2Cl + NaOH CH3CH2OH + NaCl ethyl chloride ethanol 2-Indirect hydration of olefins: H.OH CH3CH CH2 + H.HSO 4 CH3 CH CH3 propene HSO4 CH3 CH CH3 + H2SO4 OH isopropanol 3-Reduction of carbonyl compounds with lithium aluminum hydride (LiAlH 4) or H2 in presence of a catalyst; where aldehydes give primary alcohols , ketones give secondary alcohols while the reduction of the fatty acids ,esters, acid anhydride and acid chlorides give primary alcohols. a-reduction of aldehydes: LiAlH4 R C O R CH2OH H e.g.: CH3 LiAlH4 C O CH3CH2OH H acetaldehyde ethanol b-reduction of ketones LiAlH4 R C O R C R` R` OH LiAlH4 e.g.: CH3 C O CH3C CH3 CH3 OH acetone isopropyl alcohol c- reduction of fatty acids: LiAlH4 R C O R CH2OH + H2O OH LiAlH4 e.g.: CH3 C O CH3CH2OH + H2O OH acetic acide d-reduction of acid chloride: LiAlH4 R C O R CH2OH + HCl Cl LiAlH4 e.g.: CH3 C O CH3CH2OH + HCl Cl acetyl chloride e- reduction of acid anhydride: R-CO O = LiAlH4 2 RCH2OH R-CO acid anhydride CH3-CO O + LiAlH4 2CH3CH2OH CH3CO acetic acid 4- Action of Grignard'reagenrs on: a- formaldehyde gives primary alcohols: H.OH OH RMgX + C O R-CH2OMgX R-CH2OH +Mg X H H.OH CH CH OH +Mg OH CH3MgI + CH2O CH3CH2OMgI 3 2 I methyl mag.iodide ethanol aldehydes rather than formaldehyde give secondary alcohols -c H H OH H.OH RMgX + R` C O R-COMgX R-C-R` + Mg H R` OH X H H H.OH CH3MgI + CH3CH2CHO CH3 CH2COMgI CH3 CH2CCH3 propionaldehyde CH3 OH sec.butanol c- ketones give tertiary alcohols: R OH OH H.OH RMgX + R` C O R-COMgX R-C-R` +Mg X R R` R CH3 CH3 H.OH CH3MgI + CH3CCH3 CH3 COMgI CH3CCH3 acetone O CH3 OH ter.butanol e-ethylene oxide gives primary alcohol : Ag2O RMgX H.OH CH2 CH2 CH2 CH2 RCH 2CH2OMgX O R-CH2CH2OH + Mg X OH H2O CH3MgBr + CH2 CH2 CH3CH2CH2OMgBr O ethylene oxide CH3CH2CH2OH + Mg Br OH n-propanol 5-Alkaline hydrolysis of esters give alcohols and fatty acids ( their salts): H2O RCOOR' R'OH + RCOONa NaOH NaOH CH3CO2C2H5 C2H5OH + CH3CO2Na H2O ethylacetate ethanol sod. acetate 6-Action of nitrous acid on primary amines: R-CH2NH2 + HONO R-CH2OH + N2 + H2O 10 amine nitrous acid CH3CH2NH2 + HNO2 CH3CH2OH +N 2 +H2O ethanol ethyl amine Properties of alcohols Isomerism in alcohols: 1- Position isomerism which depends on the position of the functional group in the molecule. e.g.: CH3CH2CH2CH2OH CH3CH2CHCH 3 OH 1-butanol 2-butanol 2- Chain isomerism, which depends on the shape of carbon chain( straight or branched ). e.g.: CH3CH2CH2CH2OH CH3CH-CH 2OH CH3 n-butanol iso-butanol 3-Optical isomerism, present in alcohols which contain asymmetrical carbon atom, where the molecule in this case has two isomers called " enantiomers" which they are optical active ( i.e. rotate the plane of polarized light in spectrometer).e.g.: sec-butanol can has this configuration in the space: CH3 CH3CH2CH2CH2OH CH3 CH CH2OH n-butanol iso-butanol Hydrogen Bonding: The relative low volatility of alcohol is due to the association of the molecules in the liquid state, through the hydrogen bond, these hydrogen bonds could be broken by the action of heat and this explain the high boiling points of alcohols. R H etc. R R O O H O R O H H hydrogen bonding Chemical properties of alcohols: 1-Reaction with alkali metals such as Na, and K; form salts which are called alkoxides with liberation of H2: R--OH + Na R-ONa + 1/2 H2 CH3CH2OH + Na CH3CH2ONa + 1/2 H2 ethanol sodium ethoxide 2-Action of hydrogen halides ( HX) give alkyl halides (R-X) : ROH + HX RX + H2O HBr CH3CHCH3 CH3CHCH3 + H2O OH Br iso-propanol iso-propyl bromide 3-Action of phosphorouspentachloride (PCl5) or thionyl chloride ( SOCl2) give alkyl chlorides: CH3CH2OH + PCl5 CH3CH2Cl + POCl3 + HCl ethanol ethyl chloride CH3OH + SOCl2 CH3Cl + SO2 + HCl methanol methyl chloride 4-Dehydration by the action of heated alumna( Al2O3) or concentrated sulfuric acid give olefins -H2O CH3CH2OH + H.HSO 4 CH3CH2.HSO4 ethyl hydrogen sulfate o 140 C 170oC C2H5OH CH3CH2OCH 2CH3 CH2 CH2 + H2SO 4 diethyl ether ethylene The formation of ether proceeds according to Williamson continuos etherification method, where the heating of ethylhydrogen sulfate at 140C in presence of an excess of alcohol led to the formation of ether according to the following mechanism. H -H2O HOCH 2CH3 CH3CH2OH CH3CH2OH2 CH3CH2 -H CH3CH2-O-CH2CH3 CH3CH2-O-CH2CH3 H HSO4 dietyl ether if there is no adjacent -C-H , the dehydration takes place with rearrangement CH3 CH3 CH3 H -H2O CH3-C-CH2OH CH3-C-CH2OH2 CH3-C-CH2 CH3 CH3 CH3 2,2-dimethyl-1-propanol rearrangement -H CH3-C-CH2 CH3 CH3-C CHCH 3 CH3 CH3 2-methyl-2-butene Also dehydration of 2,3-dimethyl-butanol can be represented as follow: CH3 H CH3 H -H2O CH3 C C CH3 CH3 C C CH3 H+ CH3 OH CH3 secondary carbonium ion ( less stable ) CH3 H E1 CH3 C C CH3 CH2 C CH CH3 + B-elimination CH3 CH3 CH3 ter- carbonium ion ( minor ) CH3 CH3 ( more stable ) CH3 C C CH3 ( major ) 5-Esterification: Fisher method: by the reaction of alcohol with organic acid in presence of )a dehydrating agent such as hydrochloric acid in gaseous state or conc. sulfuric acid: H2SO4 R-CO2H + R`OH RCO2R` + H2O H2SO4 CH3CO2H + CH3OH CH3CO2CH3 + H2O methanol conc. methyl acetate Mechanism: OH R`OH OH H R-C OH R-C OH R-C OH O O-R` OH O H H -H2O -H R-C OH2 R-C R-C -OR` OR` OR` O b-reaction with acid chloride: - O R-C-Cl + HOR` R-C-OR` + HCl O e.g.: CH3COCl + C2H5OH CH3CO2C2H5 +HCl acetyl chloride ethyl acetate c- Reaction of alcohol with acid anhydrides: (RCO)2O + R'OH RCOOR' + RCOOH acid anhydride alcohol ester acid (CH3CO)2O + C2H5OH CH3COOC2H5 + CH3COOH acetic anhydride ethanol ethyl acetate acetic acid 6- Dehyrogenation: Dehydrogenation is carried out by passing the vapor of alcohol over heated copper ( 350C) : a- Primary alcohols give aldehydes: Cu /350o e.g.: CH3CH2OH CH3CHO +H2 acetaldehyde b-Secondary alcohols give ketones: OH O Cu /350o CH3-CH-CH3 CH3C-CH3 + H2 acetone isopropanol c-Tertiary alcohols give olefins: OH Cu /350o CH3-C-CH3 CH3-C CH2 ter.butanolCH3 isobutene CH3 7- Oxidation: Oxidation with potassium permanganate or potassium dichromate: a) 10 alc. ( O) ( O) aldehyde acid (O) (O) R CH2 OH R CHO R CO2H KMnO 4 KMnO 4 (O) b) sec. alc. ketones (O) R CH R' R C R' OH O c) ter. alcohols resist the oxidation under this conditions 8- Haloform reaction: Alcohols which have CH3 CH OH group in the presence of halogen ( X2 ) and NaOH give Haloform: 3 I2 NaOH I2 CH 3CH 2OH CH 3CHO CI 3 CHO oxidation substitution iodal ethanol acetaldehyde CHI 3 + HCOONa chloroform sod.formate Differentiation between primary, secondary and tertiary alcohols: Oxidation ( as mentioned before ) Dehyrogenation ( as mentioned before ) Victor- Mayer's method: a- Primary alcohol: AgNO3 R CH2OH + PC l 5 R CH2Cl HONO NaOH R C NO 2 red colour (NaNO2/HCl) N OH b) Secondary alcohols: AgNO 3 R CH R' + PCl5 R CH R' R CHNO 2 OH Cl R' R' HONO NaOH R C NO 2 blue colour (NaNO2/HCl) N O c) Tertiary alcohols: R" R" R" PCl5 AgNO3 HNO2 R C R' R C R' R C R' OH Cl NO2 HNO2 no reaction Dihydric Alcohol e.g. Ethylene glycol CH2(OH) - CH2(OH) Methods of preparation: 1- Hydrolysis of vic-dihalides CH2 CH2 + NaOH CH2 CH2 Cl Cl OH OH ethylene chloride 2-By passing ethylene into cold dilute alkaline permanganate (O) CH2 CH2 CH2 CH2 KMnO 4 OH OH Chemical reactions: 1- Glycols condense with aldehydes or ketones to give cyclic acetals or cyclic ketals O CH3 CH2 OH CH3 CH2 + O C C CH2 OH CH3 CH2 CH3 O 2-With hydrochloric acid (HCl): The products depends on the reaction's temperature. CH2 Cl HCl CH2 OH HCl CH2 Cl CH2 OH 160o CH2 OH 200o CH2 Cl 2-Oxidation with nitric acid gives glycollic and oxalic acids: with nitric acid, ethylene glycol can be oxidized finally to oxalic acid through different oxidative steps: CHO ( O ) C O2H CH2 OH (O) CH2 OH glycollic CH2 OH CH2 OH acid (O) (O) CHO (O) C O2H (O) C O2H CHO C O2H CHO glyoxal oxalic