Cardiovascular System Physiology PDF
Document Details
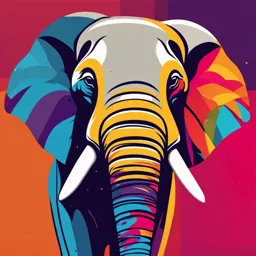
Uploaded by JawDroppingArtInformel
Lagos State University of Education
Tags
Related
- Anatomy And Physiology Of The Cardiovascular System Part 2 PDF
- Chapter 11 - Cardiovascular System PDF
- CVS Lec # 1 Physiology and Anatomy of Heart PDF
- Introduction to Cardiovascular Physiology PDF
- Basic Anatomy and Physiology 1 - Cardiovascular System - Topic 5 PDF
- Physiology of Cardiovascular System PDF
Summary
This document provides an overview of the physiology and anatomy of the cardiovascular system. It explains the functional organization of the system, including the heart's chambers, valves, and the roles of arteries, veins, and capillaries. The document also covers the anatomy of the heart, layers of the heart wall, and the microscopic anatomy of heart muscle.
Full Transcript
PHYSIOLOGY OF THE CARDIOVASCULAR SYSTEM FUNCTIONAL ORGANIZATION OF THE CVS The CVS consists of the heart and a closed system of vessels through which the heart pumps blood to all parts of the body. Arteries - arterioles- carry blood from the heart to...
PHYSIOLOGY OF THE CARDIOVASCULAR SYSTEM FUNCTIONAL ORGANIZATION OF THE CVS The CVS consists of the heart and a closed system of vessels through which the heart pumps blood to all parts of the body. Arteries - arterioles- carry blood from the heart to tissues Veins - venules - carry blood from the tissues to the heart. Capillaries connect the arterioles to venules in the tissues; The heart consists of two pumps; Right pump (right heart) receives blood from veins throughout the body into the right atrium and pumps it from the right ventricle to the lungs for oxygenation – the pulmonary circulation. The left pump receives oxygenated blood from the lungs into the left atrium and pumps it from the left ventricle to all parts of the body – systemic circulation. The pulmonary and systemic circulations are in series and form a closed circuit therefore the amount of blood pumped by each ventricle per minute is equal in a healthy individual The lymphatic system – is another system of vessels - begins from the tissues and collects small amounts of plasma proteins that escape into the interstitium and return them to the blood circulation through the thoracic duct and the right lymphatic duct into the subclavian veins. Figure 18.5 Chapter 18, Cardiovascular System 3 ANATOMY OF THE HEART The heart is enclosed in a doubled walled sac called the pericardium. The fibrous pericardium - the superficial part of the this sac – with tough dense connective tissue protects the heart anchors it to surrounding structures prevents over filling of the heart with blood the serous pericardium – deep to the fibrous pericardium - is a thin slippery two-layer serous membrane. Its parietal layer lines the internal surface of the fibrous pericardium and at the point of exit of the large heart vessels folds over to continue over the external heart surface as the visceral layer – also called the epicardium. The serous fluid between these two layers allows the heart to move without friction as it pumps. Pericarditis, Pericardial effusion, Cardiac tamponade LAYERS OF THE HEART-WALL The superficial epicardium The middle myocardium (heart muscle) The endocardium – the endothelial lining of the heart that is continuous with endothelium of blood vessels entering and leaving the heart. Layers of the Heart Wall 6 CHAMBERS OF THE HEART Each atrium empties its contents into the corresponding ventricle through a one-way valve - the atrio ventricular (AV) valves. Left AV valve has two cusps - mitral valve Right AV valve has 3 cusps - tricuspid valve The outflow from the left ventricle to the aorta and from the right ventricle to the pulmonary artery is through one-way semilunar valves with 3 cusps each namely: The aortic valve The pulmonary valves. The left ventricular walls are about four times as thick as the right. The right heart chambers are separated from the left by inter atrial and inter ventricular septa which are muscular. The atria are separated from the ventricles by an atrio ventricular fibro- tendinous ring to which the muscle fibers and AV valves are attached. This means that the only muscular connection between the atria and ventricles is the specialized bundle of muscle fibers known as the bundle of His. CHAMBERS OF THE HEART Right atrium tricuspid valve right ventricle Left atrium bicuspid valve (mitral) left ventricle Right ventricle pulmonary valve pulmonary arteries lungs Left ventricle aortic valve aorta The valves are one – way valves 8 Heart Valves Microscopic Anatomy of Heart Muscle Cardiac muscle is striated, short, fat, branched, and interconnected Intercalated discs anchor cardiac muscle cells together and allow free passage of ions Heart muscle behaves as a functional syncytium 10 Microscopic Anatomy of Heart Muscle INNERVATION OF THE HEART The heart is supplied by the parasympathetic (vagus) and sympathetic nerves Vagal supply - is only to the atria. The right vagus supplies the SA node The left vagus supplies the AV node. Stimulation of vagus slows the heart. Sympathetic supply - the sympathetics supply motor and sensory fibers to the ventricles and the atria. Stimulation of the sympathetics increases rate and force of contraction of the heart and dilates the coronary arteries. ELECTRICAL ACTIVITY OF THE HEART The ability of the cardiac muscle to depolarize and contract is intrinsic and does not depend on the nervous system. GENERATION AND SPREAD OF THE CARDIAC IMPULSE The heartbeat originates in a specialized cardiac conduction system and spreads through this system to all parts of the myocardium. This intrinsic conduction system consists of: The Sino Atrial Node (SA node) The Internodal Atrial pathways The Atrioventricular node (AV node) The Bundle of His and its branches The Purkinje system All parts of this system are capable of spontaneous discharge but the SA node discharges most rapidly (72times/min) thus spreading the impulse through the entire system and myocardium . Inherent rhythm of: AV node - 40times/min. Bundle of His – 20times/min The SA node is thus referred to as the cardiac pacemaker and its rate of discharge determines the heart rate. GENERATION AND SPREAD OF THE CARDIAC IMPULSE The SA node is located at the junction of the superior vena cava with the right atrium. Impulses it generates pass through the three bundles of atrial fibers that connect the SA node to the AV node namely:- The anterior internodal tract of Bachman The middle internodal tract of Wenckebach The posterior internodal tract of Thorel. The AV node is located at the right posterior portion of the interatrial septum. The impulses pass through the AV node to the Bundle of His. The Bundle of His gives off a left bundle branch at the top of the inter ventricular septum and continues as the right bundle branch. The branches run sub endocardially down both sides of the septum and come into contact with the Purkinje system, whose fibers spread the impulse to all parts of the ventricular myocardium GENERATION AND SPREAD OF THE CARDIAC IMPULSE Once action potentials are generated in the SA node they are conducted from cell to cell throughout the atrium and then through the conducting system to the ventricles. The atria and ventricles behave like a continuous mass of cells i.e. like a syncytium. This is made possible because of the presence of: Intercalated discs at points where the end of one myocardial (muscle fibers) cell abuts on another. They are modifications of the sarcolemma and contain gap junctions and desmosomes. They provide strong union between cells so that contraction of one unit is transmitted to the next. Gap junctions – are membrane channels that mediate the cell to cell movement of ions and small metabolites. the result is low resistance bridges for the spread of excitation from one cell to the other. ACTION POTENTIAL INITIATION BY THE AUTO RHYTHMIC CELLS The cells of the conduction system are non contractile. The autorhythmic cells of the conducting system have an unstable resting membrane potential that continuously depolarizes, drifting slowly toward threshold. These spontaneously changing membrane potentials are called pacemaker potentials or prepotentials. The action potentials in the SA and AV nodes are peculiar and different from those of other parts of the heart. The RMP is -60mV and is unstable The action potential has 3 phases, phase 4, 0 and 3. Phases 1 and 2(as seen in ventricular AP) are absent. ACTION POTENTIAL INITIATION BY THE AUTO RHYTHMIC CELLS The pacemaker potential is initiated by a group of channels called hyperpolarization-activated cyclic nucleotide-gated (HCN) channels. These channels open at very negative voltages (i.e. immediately after phase 3 of the previous AP) and allow slow influx of sodium ions. This is known as ‘funny’ current (If) At about -50mV transient (T) Ca2+ channels open and Ca2+ influx bring depolarization to the threshold (approximately - 40 mV) At threshold long lasting (L) Ca2+ channels open allowing an explosive entry of Ca2+from the extracellular space and produces the impulses (phase 0).. The repolarization phase occurs as a result of opening of K+channels and K+efflux from the cells (phase 3). Once repolarization is complete K+channels close, K+efflux declines ‘funny’ current and transient Ca channels open to begin the slow depolarization to threshold again. Action Potentials of auto rhythmic cells VENTRICULAR ACTION POTENTIALS Action potentials in the ventricle including Bundle of His and Purkinje fibers have 5 phases named 0 – 4. PHASE 0: RAPID DEPOLARIZATION WITH OVERSHOOT The RMP of contractile (myocardial) cell is -90mV. The RMP rapidly drops from -90mV to 0mV and then to +25mV relative to the outside. This is due to opening of voltage-gated Na channels with rapid influx of Na + + PHASE 1: INITIAL RAPID REPOLARIZATION From the positive potential of about +25 there is a rapid decline to 0mV due to closure of Na. Phases 0 and 1 correspondent with + the QRS wave of the ECG. PHASE 2: PLATEAU PHASE The membrane potential remains stable for about 150ms. This is because of opening of voltage gated Ca channels which allow a 2 + slow influx of Ca. This phase correspondents with ST segment of 2 + ECG. PHASE 3: RAPID REPOLARIZATION Rapid repolarization occurs due to closure of Ca 2+ channels and opening of both voltage and Ligand gated K channels allowing efflux of potassium + ions.This phase lasts 50ms, corresponds to T wave of ECG and returns membrane potential to resting level. This phase coincides with end of systole. PHASE 4: Without any further change in membrane potential the Na K pump restores Na and K to their original + + + + concentrations on either side of the cell membrane. Total duration of AP in ventricle is 250ms. Action 1 Depolarization is potential due to Na+ influx through Plateau fast voltage-gated Na+ channels. A positive feedback cycle rapidly 2 opens many Na+ Tension channels, reversing the Membrane potential (mV) development membrane potential. (contraction) Channel inactivation ends this phase. Tension (g) 1 3 2 Plateau phase is due to Ca2+ influx through slow Ca2+ channels. This keeps the cell depolarized because few K+ channels Absolute are open. refractory period 3 Repolarization is due to Ca2+ channels inactivating and K+ channels opening. This allows K+ efflux, which Time (ms) brings the membrane potential back to its resting voltage. Figure 18.12 ACTION POTENTIALS IN THE ATRIA Has same features as in ventricle (i.e. plateau phase and ionic mechanism) Is shorter in duration SPREAD OF CARDIAC EXCITATION Atrial depolarization is complete in about 0.1s. Because conduction in AV node is slow, a delay of about 0.1s (AV nodal delay) occurs before excitation spreads to ventricles. This delay is shortened by sympathetic stimulation to the heart and lengthened by vagal stimulation or digitalis. Depolarization spreads through the ventricle in 0.08 – 0.1s Rate of conduction of impulse is slowest in the SA and AV nodes – 0.05m/sec In the general atrial and ventricular muscles including Bundle of His – 1m/sec. In the Purkinje fibers – 4m/sec AV nodal delay ensures atria empty into ventricles before ventricles contract. Fast conduction of Purkinje fibers ensures impulse reaches all parts of the large ventricular mass immediately. Slow conduction in SA node prevents re-entry of impulses from atria into the pace maker. Heart Physiology: Sequence of Excitation Chapter 18, Cardiovascular System 25 Figure 18.14a REFRACTORY PERIODS OF THE HEART During the phases 0, 1, 2 and part of 3 before repolarization reaches threshold potential, the heart is absolutely refractory and no stimulus can evoke a response. Systole ends in phase 3 therefore the contractile response is over while the heart is still absolutely refractory. This means the heart cannot be tetanized like skeletal muscle. RELATIVE REFRACTORY PERIOD As repolarization progresses beyond the threshold potential to the end of phase 3, the heart enters a relative refractory period during which a strong stimulus can evoke a response. Cardiac Muscle Contraction Depolarization (AP) wave travels along T tubules membrane to the SR causing release of Ca into the 2+ sarcoplasm from SR In addition the Ca influx (responsible for the plateau) 2+ triggers opening of Ca -sensitive channels in the SR, 2+ which liberates bursts of Ca into the sarcoplasm 2+ E-C coupling occurs as Ca binds to troponin and sliding 2+ of the filaments begins The strength of cardiac muscle contraction depends greatly on concentration of Ca ions in ECF unlike skeletal muscle Duration of the AP and the contractile phase is much greater in cardiac muscle than in skeletal muscle EFFECTS OF CALCIUM AND POTASSIUM IONS ON HEART FUNCTION IONS Hyperkalemia (high blood K ) lowers the resting potential of the + cardiac cells i.e. it partially depolarizes the cell so the membrane potential is less negative. As membrane potential decreases the intensity of action potential decreases and contraction of the heart becomes progressively weaker. Heart slows and the heart becomes dilated and flaccid. Severe hyperkalemia could cause a block in conduction of impulse through the A- V bundle, severe weakness of the heart and death. Hypokalemia causes life threatening arrythmias Hypocalcemia (low blood Ca )depresses the heart because + calcium helps initiate heart contraction Hypercalcemia produces a tight coupling of the excitation – contraction mechanism thus prolonging the plateau phase of the action potential. This increases heart irritability and spastic heart contractions that allow the heart little rest. THE CARDIAC CYCLE CARDIAC CYCLE – mechanical events The depolarization in the electrical events of the cardiac cycle triggers a wave of contraction that spreads through the myocardium. This contraction results in changes in pressures, flows and blood volumes in the heart chambers. Blood circulates endlessly through the heart therefore we choose an arbitrary starting point to describe one turn of the cardiac cycle. Each point of the cycle could be called a phase. Systolic pressure – peak pressure reached during systole. Diastolic pressure – lowest pressure reached in diastole not mean pressure. VENTRICULAR FILLING: MID-TO-LATE DIASTOLE In this phase pressure in the heart is low; blood returning from the circulation is flowing passively into the atria and through the open AV valves into the ventricles. About 70 – 80% of ventricular filling occurs during this period and the cusps of the AV valves drift toward the closed position. ATRIAL SYSTOLE Atrial systole starts after the P wave of the ECG – the atria contract. This causes a sudden rise in atrial pressure which propels additional blood into the ventricles (20 – 30% of ventricular filling). At this point the ventricles are in last part of their diastole and contain the maximum amount of blood they will contain in the cycle this is known as – the end diastolic volume (EDV). VENTRICULAR SYSTOLE: Starts near the end of the R wave and ends just after the T wave. As the atria relax the ventricles begin contracting. Pressure rises in the ventricles as the myocardium presses against blood in the chambers and AV valves close causing the 1 heart st sound which is louder, longer and lower pitched than the second and best heard with the bell of the stethoscope. This initial stage when there is no appreciable shortening of ventricular muscles and the ventricles are completely closed chambers is known as period of isovolumetric (isovolumic, isometric) ventricular contraction which lasts about 0.05s until the pressures in the left and right ventricles exceed pressures in the aorta (80mm Hg) and pulmonary artery (10mmHg) causing aortic and VENTRICULAR SYSTOLE CONTD. During isovolumetric contraction the AV valves bulge into atria causing a small sharp rise in atrial pressure. When the aortic and pulmonary valves open the phase of ventricular ejection begins. Ejection is rapid at first, slowing down as systole progresses. The left ventricular pressure rises to a peak of about 120mmHg and the right to about 25mmHg or less. Ventricular contractions cause the AV valves to be pulled down and atrial pressure drops. Amount of blood ejected by each ventricle per stroke at rest is about 70 – 90ml, EDV is 140ml … about 50ml remains in each ventricle at the end of systole. – End Systolic (ventricular) volume (ESV). The ejection fraction is the proportion (expressed as a percentage) of the EDV that is ejected with each stroke. The ejection fraction is a useful index of ventricular function. It can be measured by equilibrium radionuclide angiocardiography where radionuclide labeled red blood cells are injected to image the cardiac blood pool at the end of diastole and end of systole. EARLY DIASTOLE: early diastole has 2 components. The initial part – protodiastole begins towards the end of T wave. During this period the ventricles are fully contracted but are not ejecting blood, instead intra ventricular pressures are falling because of continued outflow of blood from the arteries. The ventricles then relax suddenly and the ventricular pressure falls rapidly below the pressures in aorta and pulmonary trunk resulting in closure of the semilunar valves – which is responsible for the 2 heart sound and a notch in nd the aortic pressure recording (dicrotic notch). The continued relaxation of the ventricles while the semilunar and A-V valves are closed is called isovolumetric relaxation. All through ventricular systole and early diastole atria were in diastole, filling with blood and intra atrial pressure was rising. When intra atrial pressure exceeds pressure in ventricles the AV valves opens and ventricular filling (phase 1) begins again. Duration of the various phases of the cardiac cycle depends on heart rate. Increase in heart rate shortens systole and diastole but diastolic shortening is more. DURATION OF CARDIAC CYCLE At a heart rate of about 75 beats/min duration of cardiac cycle is 0.8s; systole – 0.27s, diastole 0.53s. At heart rate of 200/min duration of cardiac cycle is only 0.3s. Systole – 0.16s Diastole – 0.14s This is because cardiac muscle has the unique property of contracting and repolarizing faster when heart rates increase. Duration of the cardiac action potential, absolute and relative refractory periods are shortened. The physiologic and clinical implications of this are: Heart muscle rests during diastole Blood flow to subendocardial portions of the left ventricle occurs only during diastole. Most of ventricular filling occurs during diastole But at very high heart rates filling may be compromised to such a degree that cardiac output per minute falls and symptoms of heart failure develop. HEART SOUNDS First one is a low-slightly prolonged lub caused by closure of the A-V valves at the start of ventricular systole. The second is a shorter high pitched dup caused by closure of the aortic and pulmonary valves just after end of ventricular systole. Right atrial systole precedes left atrial systole. Left ventricular systole precedes right ventricular systole. During expiration aortic and pulmonary valves close at the same time. During inspiration the aortic valves closes before the pulmonary valve - physiologic splitting of the 2 heart sound. If there is left bundle branch block nd it delays closure of aortic valve so it closes after pulmonary valve - paradoxical splitting of second sound. Atrioventricular Valve Function Semilunar Valve Function Mitral Valve Prolapse HEART SOUNDS Third heard sound- occurs in earlyu diastole as a result of rapid ventricular filling – ventricular gallop or protodiastolic gallop. it is not audible by stethoscope. Fourth heart sound – is sound produced by contraction of atrial musculature. It is inaudible. Only becomes audible when ventricle are stiff from hypertrophy, aortic stenosis causing the atria to contract more forcefully. ABNORMAL HEART SOUNDS These are called murmurs. Murmurs occur when normal streamline flow of blood in the heart changes to turbulent flow. This could happen in Valvular diseases Septal defects Vascular defects Valvular diseases could cause either stenosis or incompetence TYPES OF MURMURS Systolic murmurs can be caused by: AV valve incompetence Semilunar valve stenosis Anemia Septal defects Coarctation of aorta Diastolic murmurs: AV valve stenosis SL valve incompetence Continuous murmurs: Patent ductus arteriosus HEART RATE AND ITS REGULATION Definition: heart rate is the number of times the heart beats per minute. Basic heart rate is set by the intrinsic conducting system -SA node. This rate is modified by the autonomic nervous system. Stimulation of the sympathetic increases the rate (chronotropy) and force (inotropy) of contraction parasympathetic stimulation slows the heart. The heart is under tonic inhibition from the vagus nerve which predominates over the excitation from the sympathetic nerve. Both systems work in a reciprocal relationship; an increase in sympathetic stimulation is accompanied by a decrease in vagal inhibition and vice versa. Sympathetic and parasympathetic discharge to the heart is however under the control of groups of neurons in the medulla called the cardiac center. Extrinsic Innervation of the Heart Heart is stimulated by the sympathetic cardioacceleratory center Heart is inhibited by the parasympathetic cardioinhibitory center 45 Figure 18.15 Cardiac Center Consists of : The cardio inhibitory area located in the dorsal nucleus of the vagus and the nucleus ambiguous sends parasympathetic nerves via the vagus to the heart, The cardio acceleratory area projects to sympathetic neurons in the T – T levels of the 1 5 spinal cord. These preganglionic neurons synapse with ganglionic neurons in the upper thoracic sympathetic trunk. Sensory area located in the nucleus tractus solitarius The heart is exhibits a vagal tone. Cutting the vagus increases the HR from 70 – 75/min to 100 – REGULATION OF HEART RATE HIGHER CENTERS: Centers in the cerebral cortex (limbic cortex) via the hypothalamus stimulate the cardiac center and affect heart rate. Specific factors include; excitement, anger fear and sexual arousal increase HR. Depression and grief can decrease HR. RESPIRATORY CENTERS: During inspiration respiratory centers in medulla radiate impulses to cardiac center causing an increase in HR. During expiration HR falls. This phenomenon is known as sinus arrhythmia. CHEMORECEPTORS: Stimulation of chemoreceptors(aortic and carotid bodies) by rise in pCO 2 and hydrogen ion concentration not only feeds back impulses to respiratory centers in medulla but also exerts influence on vasomotor and cardiac center. This results in peripheral vasoconstriction and bradycardia. Hypoxia however causes hyperpnea and increased secretion of catecholamines from adrenal glands resulting in tachycardia. BARORECEPTORS: When stimulated the baroreceptors send their impulses via the vagus and glossopharyngeal nerves to the nucleus tractus solitarius which sends inhibitory impulses to the cardio accelerator area and vaso constrictor nerves but excites vagal innervation to the heart. This results in a slowing of heart rate, vasodilation and fall in blood pressure. ATRIAL RECEPTORS: Stimulation of type B atrial stretch receptors leads to an increase in heart rate. Type B atrial stretch receptors discharge in late diastole at peak of atrial filling. The type A receptors discharge during atrial systole. Rapid infusion of blood or saline sometimes increases heart rate if it was initially low. This is known as the Bainbridge reflex. It is mediated by atrial stretch receptors via the vagus (vagal inhibition and sympathetic facilitation). It is abolished by vagotomy. The reflex competes with baroreceptor mediated decrease in heart rate produced by volume expansion. HYPOXIA AND HYPERCAPNIA: These have a direct effect on cardiac center to increase heart rate. CORONARY CHEMOREFLEX: (Bezold Jarisch Reflex) In experimental animals injection of some drugs e.g. serotonin, capsaicin and phenyl diguanides into coronary artery supplying left ventricle causes apnea followed by rapid breathing, hypotension and bradycardia – this is known as the coronary chemoreflex. Such a response is not observed with drug infusion into atria or right ventricle. EXERCISE: During anticipatory phase of exercise vagal inhibition causes increased heart rate. As exercise progresses sympathetic stimulation contributes to increased heart rate. SLEEP: During sleep HR is slowest. PHYSICAL TRAINING: increases vagal tone lowering the heart rate. Heart rate is lower in those who are physically fit. TEMPERATURE: Rate of SA node discharge increases with temperature increase. HORMONES: Thyroxine increases heart rate by increasing number of and affinity of β – adrenergic receptors in the heart thereby increasing its sensitivity to catecholamines. Hyperthyroidism cause tachycardia that persists during sleep while hypothyroidism results in bradycardia. SINUS DISORDER: (SICK SINUS SYNDROME) This occurs in the elderly and maybe due to SA node fibrosis or degeneration. It presents as bradycardia, sinus arrest with pauses, paroxysmal tachycardia and other forms of arrhythmias. JAUNDICE AND RAISED INTRACRANIAL PRESSURE: cause bradycardia.