Carbohydrate Metabolism PDF
Document Details
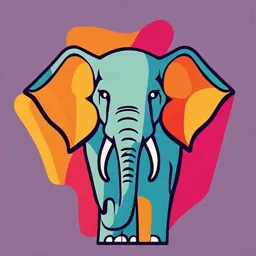
Uploaded by ProblemFreeBalalaika
Tags
Summary
This document provides an overview of carbohydrate metabolism, detailing various processes and pathways involved in carbohydrate digestion, transport, and utilization within the body. It discusses catabolism and anabolism, highlighting key enzymes and reactions. This document is not an exam paper.
Full Transcript
Metabolism Metabolism (from Greek: "metabolē", "change" or Greek : metabolismos, "outthrow") is the set of chemical reactions that happen in living organisms to sustain life. These processes allow organisms to grow and reproduce, maintain their structures, and respond to their environments. Metab...
Metabolism Metabolism (from Greek: "metabolē", "change" or Greek : metabolismos, "outthrow") is the set of chemical reactions that happen in living organisms to sustain life. These processes allow organisms to grow and reproduce, maintain their structures, and respond to their environments. Metabolism is usually divided into two categories. Catabolism: It is break down of organic matter, for example to harvest energy. Anabolism: It uses energy to construct components of cells such as proteins and nucleic acid The chemical reactions of metabolism are organized into metabolic pathways, in which one chemical is transformed through a series of steps into another chemical, by a sequence of enzymes. Carbohydrate metabolism Carbohydrate enter the body in complex forms, such as disaccharides and the polymers starch and glycogen. The polymer cellulose is also consumed but not digested. The first step in the metabolism of digestible carbohydrate is the conversion of the higher polymers to simpler, soluble forms that can be transported across the intestinal wall and delivered to the tissues. The breakdown of polymeric sugars begins in the mouth. Saliva has a slightly acidic pH of 6.8 and contains lingual amylase that begins the digestion of carbohydrates. The action of lingual amylase is limited to the area of the mouth and the esophagus; it is virtually inactivated by the much stronger acid pH of the stomach. Once the food has arrived in the stomach, acid hydrolysis contributes to its degradation; specific gastric proteases and lipases aid this process for proteins and fats, respectively. The mixture of gastric secretions, saliva, and food, known collectively as chyme, moves to the small intestine. The main polymeric-carbohydrate digesting enzyme of the small intestine is α-amylase. This enzyme is secreted by the pancreas and has the same activity as salivary amylase, producing disaccharides and trisaccharides. The latter are converted to monosaccharides by intestinal saccharidases, including maltases that hydrolyze di- and trisaccharides, and the more specific disaccharidases, sucrase, lactase, and trehalase. The net result is the almost complete conversion of digestible carbohydrate to its constituent monosaccharides. The resultant glucose and other simple carbohydrates are transported across the intestinal wall to the hepatic portal vein and then to liver parenchymal cells and other tissues. There they are converted to fatty acids, amino acids, and glycogen, or else oxidized by the various catabolic pathways of cells. Absorption of Glucose and Other Monosaccharides : Transport Across the Intestinal Epithelium Absorption of glucose is transport of glucose from the intestinal lumen, across the epithelium and into blood. The transporter that carries glucose and galactose into the enterocyte is the sodium- dependent hexose transporter, known more formally as SGLUT-1 As the name indicates, this molecule transports both glucose and sodium ion into the cell. 1. The transporter is initially oriented facing into the lumen - at this point it is capable of binding sodium, but not glucose 2. Sodium binds, inducing a conformational change that opens the glucose-binding pocket. 3. Glucose binds and the transporter reorients in the membrane such that the pockets holding sodium and glucose are moved inside the cell. 4. Sodium dissociates into the cytoplasm, causing glucose binding to destabilize. 5. Glucose dissociates into the cytoplasm and the unloaded transporter reorients back to its original, outward-facing position. Fructose is not co-transported with sodium. Rather it enters the enterocyte by another hexose transporter (GLUT5) Once inside the enterocyte, glucose and sodium must be exported from the cell into blood. Sodium is rapidly shuttled out in exchange for potassium by the battery of sodium pumps on the basolateral membrane. The energy stored in this gradient is actually what is driving glucose entry through the sodium-dependent hexose transporter described above. Glucose, galactose and fructose are tranported out of the enterocyte through another hexose transporter (GLUT-2) in the basolateral membrane. These monosaccharides then diffuse "down" a concentration gradient into capillary blood within the villus. Fate of the absorbed sugars Absorbed monosaccharides (glucose, galactose and fructose) are undergo following: A- Uptake by liver: After absorption liver uptakes sugars and converted galactose and fructose into glucose. B- Glucose utilization by tissues: glucose undergo one of the following: 1- Oxidation through: a) Major pathways (glycolysis and Kreps` cycle) for production of energy. b) Pentose phosphate pathway for production of pentose and NADPH+H. c) Uronic acid pathway for production of glucuronic acid used for detoxification. 2- Storage in the form of: a) Glycogen (glycogenesis). b) Fat (lipogenesis). 3- Conversion to substances of biological importance: a) Ribose and Deoxyribose for DNA and RNA structure. b) Lactose for milk c) Glucosamine and Galactosamine for production of mucopolysaccharides. d) Gulucoronic acid for production of glucosaminglucan and mucopolysaccharides. e) Fructose in semen. Glycolysis (embden Meyerhoph pathway) Glycolysis is Oxidation of glucose to either pyruvate under aerobic conditions in most and the pathway is known as aerobic glycolysis or lactate when oxygen is depleted, as for instance during prolonged vigorous exercise, and the process is known as anaerobic glycolysis. Site: cytosol of all tissue cells. It is of physiological importance in: 1- Tissues with no mitochondria like mature RBCS, cornea and lens. 2- Tissues with few mitochondria like testes, leucocytes, medulla of kidney, retina, skin and GIT. 3- Tissues under frequent lack of oxygen like skeletal muscle especially during exercise. Steps of glycolysis Stages of glycolysis 1- Stage 1 (energy requiring stage): One molecule of glucose give two molecule of glyceraldehyde-3 phosphate. These steps require 2 molecules of ATP (energy loss). 2- Stage 2 (energy producing stage): Two molecules of glyceraldehyde-3 phosphate are converted into 2 pyruvate molecules (aerobic glycolysis) or 2 molecules of lactate (anaerobic glycolysis). These steps produce ATP molecules (energy production). Energy (ATP) poduction of glycolysis ATP production = ATP produced- ATP utilized. ATP produced ATP utilized Net ATP In absence 4 ATP (substrate level 2 ATP 2 ATP of oxygen physphorylation) - From glucose to (Anaerobic) - 2ATP from 1,3 BPG. glucose-6-p. - 2ATP from phosphoenol pyruvate. - From fructose-1p to fructose 1,6 bisp. In presence 4 ATP (substrate level 2 ATP 6 ATP of oxygen physphorylation) - From glucose to (Aerobic) - 2ATP from 1,3 BPG. glucose-6-p Or - 2ATP from phosphoenol pyruvate. - From fructose-1p to 8 ATP 4 ATP or 6 ATP fructose 1,6 bisp. From oxidation of 2 NADH+H in mitochondria. Differences between aerobic and anaerobic glycolysis Aerobic Anaerobic End product Pyruvate Lactate Energy 6 or 8 ATP 2 ATP Regeneration of NAD Trough respiratory chain in Through lactate formation mitochondria Availability to TCA Available Not available Regulation of glyolysis The rate of glycolysis is regulated by controlling three irreversible enzymes (key enzymes), hexokinase (glucokinase), phosphofructokinase-1and pyruvate kinase. Glucose Insulin + Glucagon - Glucokinase - Glucose-6-p Hexokinase - ATP Insulin + - Citrate Glucagon - Phospho-fructokinase-1 + AMP + Fructose 2,6 biphosphate + - ATP Insulin Pyruvate kinase + Glucagon - Fructose 2,6 biphosphate Pyruvate Special feature of glycolysis in RBCs 1- Mature RBCs contain no mitochondria thus they depend only upon glycolysis for energy production (2 ATP) and the lactate is always the end product. 2- Glucose uptake by RBCs is independent of insulin hormone. 3- Reduction of met-Hb: Met-Hb binds oxygen irreversibly. Glycolysis produces NADH + H, which used for reduction of met-Hb in red cells into Hb. This reaction is catalyzed by cytochrome b5-met-Hb reductase system (cyt b5) Met-Hb + NADH + H Hb + NAD 4- 2,3 Bisphoglycerate: RBCs have the ability to form 2,3 Bisphoglycerate (2,3 BPG) through Rapoport-Luebering cycle or 2,3 BPG cycle. 2,3 BPG decrease affinity of Hb to O2 that gives good oxygenation of tissues. Rapoport-Luebering cycle Function of glycolysis Glucose GDH-3p DHAp Lipogenesis 1,3 BPG ATP Affinity of Energy 2,3 BPG Hb to O2 3 phosphoglycerate Serine Alanine Pyruvate Pyruvate Acetyl CoA Kerbs’ cycle Importance of anaerobic glycolysis In the absence of oxygen glucose gives lactate so lactate is the end product of anaerobic glycolysis. In absence of oxygen NADH +H is not oxidized by respiratory chain thus the conversion of pyruvate to lactate is the mechanism for regeneration of NAD. This help continuity of glycolysis as the generated NAD will be used once more for oxidation of another molecule of glucose. Source and fate of lactate: a) Source: from glycolyis especially in RBCs due to absence of mitochondria and muscle during exercise due to lack of oxygen. b) Fate: 1- Glucose formation through lactic acid cycle (Cori cycle): Lactate formed in muscles and RBCs may diffuse to the blood then to liver. In the liver, lactate is converted to glucose by gluconeogenesis. Glucose may diffuse back to the blood then to red cells or muscles to be used for energy production Cori cycle: It is the conversion of glucose into lactate in peripheral tissues followed by conversion of lactate into glucose in the liver 2- Conversion into pyruvate: if oxygen gets available lactate is converted into pyruvate which proceeds into Krebs’ cycle. 3- Lactate may accumulated in muscle causing muscle fatigue. 4- lactate is excreted in urine and sweat. Lactate dehydrogenase: It is enzyme which catalyzes the reaction Lactate pyruvate. This reation helps reoxidation of NADH + H into NAD. Fermentation: It is the conversion of glucose into ethanol by yeast enzymes and bacteria. Pyruvate is formed from glucose then pyruvate is converted into acetaldhyde then ethanol. O Pyruvate decarboxylase Alcoholic dehydrogenase CH3-C-COOH CH3-CHO CH3-CH2-OH Mg CO2 NADH +H NAD Thus the end product of fermentation is CO2 and ethanol. Mitochondrial pathway for glucose oxidation Complete oxidation of glucose occurs in both cytosol (glycolysis) and mitochondria (Kerbs’ cycle). In the presence of oxygen pyruvate pass by special pyruvate transporter into mitochondria which proceeds as follows: A- Oxidative decarboxylation of pyruvate to acetyl CoA. B- Acetyl CoA is then oxidized completely to CO2, H2O through Kerbs’ cycle. A- Oxidative decarboxylation of pyruvate to acetyl CoA O Pyruvate dehydrogenase O CH3-C-COOH CH3-C-SCoA TPP S FAD CO2 NAD L S CoASH NADH +H Enzyme: Pyruvate dehydrogenase (PDH) complex It composed of 3 subunits which catalyze the reaction Pyruvate decarboxylase, Dihydrolipoyl transacetylase and dihydrolipoyl dehydrogenase. These enzyme needs 5 co-enzymes all are vitamin B complex derivatives. 1- Thiamin pyrophosphate (TPP) = vitamin B1 2- Lipoic acid 3- Coenzyme A= CoA 4- Flavin adenine dinucleotide = FAD 5- Nicotinamide adenine dinucleotide = NAD Location of PDH: It is located within mitochondrial matrix. Energy production (3 ATP) from NADH H Regulation of PDH PDH present in two forms: Phosphorylated form (inactive) and dephosphorylated form (active). PDH kinase converts the active PDH into inactive PDH. PDH phosphatase converts the inactive PDH into active PDH. Factors stimulating PDH are Pyruvate, CoASH, NAD (inhibit PDH kinase) and insulin H (stinulate PDH phosphatse). Factors inhibiting PDH are NADH H, ATP, acetylCoA (stimulate PDH kinase). Krebs cycle (Citric acid cycle) (Tricarboxylic acid cycle, TCA) Also it called catabolism of acetyl CoA. Def: It is a series of reactions in which acetyl CoA is oxidized into CO2 and H2O and energy Location: Mitochondria. The enzymes of TCA cycle are present in the mitochondrial matrix either free or attached to the inner surface of the mitochondrial membrane. The cycle is started by acetyl CoA (2 carbon) an oxaloacetate (4 carbon) to form citrate (6 carbon). It ends by oxaloacetate (4 carbon). The differences between starting compound citrate (6 carbon) and the ending compound (oxaloacetate 4 carbon) is 2 carbon that are removed in the form of CO2. These 2 carbons derived from acetyl CoA. For this reason acetyl CoA is completely catabolized in TCA. Energy production of TCA Oxidation of one molecule of acetyle CoA in TCA produces 12 ATP molecules. 11 ATP molecules by respiratory chain phosphorylation and 1 ATP by substrate level phosphorylation. Energy production of complete oxidation of one molecule of glucose Glucose oxidation 36 or 38 ATP Pyruvate oxidation 15 ATP Acetyl CoA 12 ATP Function of TCA cycle 1- Energy production 12 ATP 2- Catabolic functions: TCA is the final common pathway for oxidation of carbohydrate, lipids and protein. 3- Anabolic function formation of: 1- Amino acids: A-Ketoglutrate Transamination Glutamate Oxaloacetate Transamination Aspartate 2-Glucose: Α-Ketoglutrate Gluconeogenesis Glucose 3- Heme synthesis: Succinyl CoA Heme 4- Fatty acid and cholesterol: from acetyl CoA (synthesized in cytosol). N.B. Acetyl CoA is produced in mitochondria however, the inner mitochondrial membrane is impermeable to acetyl CoA to overcome this impermeability acetyl CoA combines with oxaloacetate to form citrate which, diffuses to cytosol and dissociated into acetyl CoA and oxaloacetate. 5- CO2 produced in TCA is used in the following reactions (CO2 fixation reactions) Pyruvate + CO2 Oxaloacetate Gluconeogenesis Glucose Acetyl CoA + Co2 Malonyl CoA Fatty acids Ammonia + ATP + CO2 carbamoyl phosphate urea and pyrimidine Propionyl CoA + CO2 Methyl malonyl CO succinyl CoA Formation of C6 of purine. Synthesis of H2Co3/ HCO3 buffer. Regulation of citric acid cycle TCA is regulated through the key enzymes (Citrate synthase, isocitrate dehydrogenase and A-ketoglutarate dehydrogenase) and the avilability of O2. a) Citrate synthase: 1- stimulated by acetly CoA, oxaloacstate, ADP and NAD. 2- Inhibited by long chain fatty acid, citrate, succinyl CoA, ATP and NADH + H. b) Isocitrate dehydrogenase and A-ketoglutarate dehydrogenase: 1- Stimulated by ADP and NAD. 2- Inhibited by ATP and NADH +H. c) Availability of oxygen: TCA needs oxygen to proceed. In absence of O2 respiratory chain is inhibited leading to increase of NADH +H which Inhibit TCA cycle. Oxidation of extramitochondrial NADH+H Cytosolic NADH+H can not penetrate mitochondrial membrane, however they can be used to produce energy by respiratory chain of phosphorylation in mitochondria. This can be done by using special carriers for hydrogen of NADH+H. These carriers are either 1- Dihydroxyacetone phosphate (glycerophosphate shuttle) 2- Or oxaloacetate (aspartate- malate shuttle). Substrate level phosphorylation This means phosphorylation of ADP to ATP occur at the reaction itself. Examples: 1,3 biphosphoglycerate + ADP 3 phosphoglycerate + ATP Phospho-enolpyruvate + ADP Pyruvate + ATP Glycerophosphate shuttle It is important in certain muscles and nerve. Cytoplasmic NADH reduces dihydroxyacetone phosphate to glycerol 3-phosphate which then diffuses across the outer mitochondrial membrane. Glycerol 3-phosphate is then oxidised back to dihydroxyacetone phosphate by FAD which becomes redueced to FADH2. The enzyme responsible (glycerol 3-phosphate dehydrogenase is associated with the outer surface of the inner mitochondrial membrane. The FADH 2 is oxidised back to FAD by the electron transport chain. Dihydroxyacetone phosphate then returns to the cytoplasm. The final energy produced is 2ATP because oxidation of FAD2 in respiratory chain gives 2 ATP. Aspartate- Malate shuttle It is important for certain tissues like liver and heart. For oxidising cytoplasmic NADH back Cytoplasmic NADH reduces oxaloacetate to malate which then shuttles the the reducing equivalent across the outer and inner mitochondrial membranes. Malate is then oxidised back to oxaloacetate by mitochondrial NAD which becomes redueced to NADH. The mitochondrial NADH is oxidised back to NAD by the electron transport chain. The oxaloacetate must now be returned to the cytoplasm but it cannot cross the inner mitochondrial membrane. To get round this problem an amino group is transfered from glutamate to oxaloacetate to form alpha-ketoglutarate and aspartate (this is a transaminase reaction). Aspartate can cross into the cytoplasm where it is converted back to oxaloacetate by a reversal of the transaminase reaction. Note that 2 transport proteins are required to transport the molecules involved across the inner mitochondrial membrane: Transporter 1 rof etaratulgotek-ahpla segnahcxe )retropsnart etaratulgotek -ahpla(.etalam Transporter 2 rof notorp a dna etamatulg segnahcxe )retropsnart etatrapsa/etamatulg(.etatrapsa The final energy produced is 3ATP because oxidation of NADH+H in respiratory chain give 3 ATP. Biological oxidation Energy is required to maintain the structure and function of the body. Energy derived from oxidation of CHO, lipids and proteins of diet. The energy liberated from food oxidation is partly converted into ATP. Each gram of CHO and protein gives about 4 Kcal on oxidation, while oxidation of each gram of fat gives about 9 Kcal. Oxidation means: the addition of oxygen to a compound, removal of hydrogen from a compound or removal of electron from an ion or atom. Reduction means: the removal of oxygen from a compound, addition of hydrogen to a compound or addition of electron to an ion or atom. The oxidation reactions are accompanied by reduction reactions and they called Redox reactions. Redox reactions are accompanied by energy liberation. N.B. Hydrogen atom is formed of one electron (e) and one proton (H). Redox potential = electron affinity: Oxygen has the highest electron affinity (highest redox potential). Hydrogen has the lowest electron affinity (lowest redox potential). Redox chain: It is a chain of different compounds of increasing redox potential between hydrogen and oxygen. Respiratory chain (Electron transport chain)(ETC( Electron transport chains are the cellular mechanisms used for extracting energy from redox reactions, such as the oxidation of sugars. The electron transport chain consists of a spatially separated series of redox reactions in which electrons are transferred from a donor molecule to an acceptor molecule. It couples electron transfer between an electron donor such as NADH )and an electron acceptor( such as O2 )with the transfer of H+ protons) across a membrane. The function of the electron transport chain is to produce a transmembrane proton electrochemical gradient as a result of the redox reactions which, used to generate chemical energy in the form of (ATP(. 400px-Mitochondrial_electron_transport_chain%E2%80%94Etc4 Electron transport chainna sesirpmoc enzymatic series of electron donors and acceptors. Each electron donor passes electrons to a more electronegative acceptor, which in turn donates these electrons to another acceptor, a process that continues down the series until electrons are passed to oxygen, the most electronegative and terminal electron acceptor in the chain. The Electron Transport consists of 3 complexes of integral membrane proteins NADH dehydrogenase (Complex I) cytochrome c reductase (Complex III ) cytochrome c oxidase (complex IV) and two freely-diffusible molecules Ubiquinone and cytochrome c that shuttle electrons from one complex to the next. The electron transport chain accomplishes the transfer of electrons from NADH dna FADH to oxygen ot selucelom form water molecules H2Osnotorp fo dia ht( with The entire process is called oxidative phosphorylation ,since ADP is phosphorylated to ATP using the energy of hydrogen oxidation. A small amount of ATP is available from substrate level phosphorylation While the majority of ATP is generated in electron transport chains. File:ETC.PNG Complex I In Complex I (NADH dehydrogenase) ,also called NADH:ubiquinone oxidoreductase; two electrons are removed from NADH and transferred to a lipid-soluble carrier, ubiquinone,tcudorp decuder ehT.)Q( ubiquinol ( QH2) freely diffuses within the membrane, and Complex I translocates four protons (H+) across the membrane, thus producing a proton gradient. The pathway of electrons occurs as follows:NADH is oxidized to NAD+, by reducing Flavin mononucleotide (FMN) to FMNH2. Each electron thus transfers from the FMNH2 to ubiquinone (Q) form, QH2.FMNH2 is then oxidized. Complex II: In Complex II (Succinate dehydrogenase )additional electrons are delivered into the quinone pool (Q) originating from succinate, fatty acids and glycerol 3-phosphate and transferred via FAD to Q. Complex III: In Complex III ) Cytochrome bc complex) the Q-cycle contributes to the proton gradient by an asymmetric absorption/release of protons. Two electrons are removed from QH2 and sequentially transferred to two molecules of Cytochrom c a water- soluble electron carrier located within the intermembrane space. Complex IV: In Complex IV (Cytochrom c oxidase )four electrons are removed from four molecules of cytochrom c and transferred to molecular oxygen (O2) ,producing two molecules of water. At the same time, four protons are translocated across the membrane, contributing to the proton gradient. Complex V (ATP synthase): Through which protons outside the inner mitochondrial membrane enter the mitochondrial matrix. This result in synthesis of ATP from ADP and Pi. So it converts this mechanical into chemical energy by producing ATP ,which powers most cellular reactions. Summary Complex I (NADH coenzyme Q reductase )accepts electrons from the Krebs cycle electron carrier nicotinamide adenine dinucleotide (NADH), and passes them to coenzyme Q( ubiquinone, UQ), which also receives electrons from complex II (Succinate dehydrogenase.) UQ passes electrons to complex III (Cytochrom bc1 ) which passes them to cytochrom c ( cyt c). Cyt c passes electrons to (Complex IV( cytochrom c oxidase ,which uses the electrons and hydrogen ions to reduce molecular oxygen to water. NADH → Complex I →Q → Complex III →cytochrome c → Complex IV → O2 ↑ Complex II ↑ FADH2 Oxidative phosphorylation Energy obtained through the transfer of electrons down the ETC is used to pump protons from the mitochondrial matrix into the intermembrane space, creating an electrochemical proton gradient across the mitochondrial inner membrane (IMM). This electrochemical proton gradient allows ATP synthase (ATP-ase) to use the flow of H+ through the enzyme back into the matrix to generate ATP from (ADP) and inorganic phosphate. So the flow of electrons from NADH to oxygen (oxidation ) results in ATP synthesis by phosphorylation of ADP by inorganic phosphate (phosphorylation). Therefore there is a coupling between oxidation and phosphorylation. Uncouplers Coupling the oxidation and phosphorylation is a key step for ATP production. However, in specific cases, uncoupling the two processes may be biologically useful. The uncoupling protein ,thermogenin, presents in the inner mitochondrial membrane of brown adipose tissue provides for an alternative flow of protons back to the inner mitochondrial matrix. This alternative flow results in thermognesis rather than ATP production and generates heat. Uncouplers are substances that allow oxidation to proceed but prevent phosphorylation. So energy released by electron transport will be lost in form of heat A) Oligomycin: it binds the stalk of the ATP synthase, closes the H channel and prevent re-entry of protons to the mitochondrial matrix. B) 2,4 Dinitrophenol: It increases the permeability of the inner mitochonderial membrane to proton causing decrease proton gradient. C) Calcium and high doses of aspirin. D) Ionophores such as antibiotic they inhibit phosphorylation because they decrease both electrical and pH gradient. So intake of these substances cause hotness. Pentose phosphate pathway( Phosphogluconate pathway( Hexose monophosphate shunt) Def:.noitadixo esoculg rof yawhtap evitanretla si tI It generates NADPH and pentoses, while ATP is neither produced nor utelized Location is cytosol Enzymes that function primarily in the reductive direction utilize the NADP+/NADPH cofactor pair as co-factors. The reactions of fatty acid biosynthesis and steroid biosynthesis utilize large amounts of NADPH so PPP are occur in the following tissues:. 1-Those need NADPH+H for fatty acids biosynthesis such as adipose tissue and liver. 2 -Those need NADPH+H for steroids biosynthesis such as adrenal cortex, ovaries and testes and lactating mammary glands. 3 -RBCs need NADPH+H for production of reduced glutathione. 4-Retina needs NADPH+H for reduction of retinal into retinol. 5 -In many tissues as it supplies pentoses for synthesis of nucleotides. N.B. tcaf eht nI 30 %of the oxidation of glucose in the liver occurs via the PPP. PPP occurs in two distinct phases. A- The first is the oxidative phase (irreversible( in which NADPH+H and CO 2 are generated. B- The second is the non-oxidative (reversible).esotnep fo sisehtnys rof The primary functions of this pathway are: 1. To generate NADPH which essential for: A- Synthesis of many substrates ,senomroh diorets ,loretselohc ,sdica yttaf sa hcus yb etavuryp morf etalam ,sdica onima laitnesse non ,sdipilocylg ,enisognihps.msilobatem dica cinoroculg rof laitnesse dna emyzne cilam B- Reductive biosynthesis reactions within RBCs:enoihtatulg fo noitcuder : G-S-S-G Glutathione reductase 2 G-SH Oxidized glutathione NADPH+H NADP Reduced glutathione Reduced glutathione is essential for: 1 -Normal integrity of RBCs. 2 -Maintenance of SH group of RBCs enzymes. 3 -Removal of H2O2 which is toxic and cause cell membrane fragility. 4 -Inactivation of insulin. 5 -Detoxivication of many drugs and carciogens. C- Hydroxylation of aromatic and aliphatic compounds in the liver H+HPDAN : lamosorcim revil rof emyzneoC saaP-450 mono-oxygenase enzyme which hydroxylate the toxic compounds such as alcohol, steroids and many drugs to non toxic compounds. d- Phagocytosis in white blood cwlls (respiratory burst): Respiratory burst (Oxygen burst) is rapid consumption of molecular O2 that accompanies the formation of superoxide. White blood cells contain an enzyme NADPH+H oxidase (present in cell mem). After phagocytosis NADPH+H oxidase converts O2 into super oxide (O2.- Suber oxide is then converted into H2O2 by superoxide dismutase. H2O2 by myeloperoxidase in the presence of HCl is converted into hypochlorite (HOCl) which kill bacteria. Deficiency of NADPH+H oxidase leads to chronic bacterial infection. 2 -Provide the cell with ribose-5-phosphate )R5P( eht fo sisehtnys eht rof -oc dna )PTG ,PTA( seditoelcun ,)ANR ,AND( sdica cielcun dna seditoelcun.)DAF dna PDAN ,DAN( emyzne 3 -Although not a significant function of the PPP, it can operate to metabolize dietary pentose sugars derived from the digestion of nucleic acids. Regulation of PPP Glucose-6-phosphate dehydrogenase is the key enzyme of PPP. It stimulated by insulin and NADP. It inhibited by actyl CoA and NADPH+H. Differences between PPP and glycolysis PPP Glycolysis Location In certain cells In all cells Oxidation Oxidation occur in first reaction Phosporylation occur in first of glucose reaction coenzyme NADP NAD Energy No energy production 2 or 8 ATP CO2 Produced Not produced Pentose Produced Not produced PPP in skeletal muscle Skeletal muscles is poor in glucose-6-phosphate dehydrogenase but they contain transketolase and transaldolase. Skeletal muscles obtain their pentose by reversible reactions of PPP using fructose-6-phosphate and glyceraldhyde-3-phosphate and the enzymes transketolase and transaldolase. Xylulose-5-phosphate Ribose-5-phosphate Transketolase Glyceraldhyde-3-phosphate Sedoheptolase-7-phosphate Transaldolase Fructose-6-phosphate Erythrose-4-phosphate Xylulose-5-phosphate Transketolase Fructose-6-phosphate Glyceraldhyde-3-phosphate Defects of PPP Favism (Deficiency of glucose-6-phosphate dehydrogenase) It is type of hemolytic anemia (excessive destruction of RBCs). Cause ingestion of some oxidizing agents such as Fava beans, primmaquine (antimalarial drug), stryptomycin and sulfa. These compounds alter the structure of enzyme which decrease the activity of G-6-p dehydrogenase activity. Mechanism Deficiency of G-6-p dehydrogenase decreased NADPH+H production which reduce Glutathione in RBCs. G-S-S-G Glutathione reductase 2 G-SH Oxidized glutathione NADPH+H NADP Reduced glutathione Reduced glutathione is needed to remove H2O2 which is toxic to cells. 2 G-SH + H2O2 Glutathione Peroxidase + G-S-S-G 2 H2O So Deficiency of G-6-p dehydrogenase NADPH+H reduced glutathione Accumulation of H2O2 RBCs hemolysis Effects of H2O2 on RBCs 1- H2O2 causes peroxidation to the fatty acids in the cell membrane leading to hemolysis. 2- H2O2 causes conversion of Hb to Met-Hb. These toxic compounds increase the fragility of RBCs. Symptoms and signs of favism Hemolytic anemia, Jundice and decreased the concentration of Hb. Uronic acid pathway It is an alternative oxidative pathway for glucose (minor pathway) in which glucose is converted into glucuronic acid. It does not lead to generation of ATP Location It occurs mainly in liver cytoplasm , Importance of uronic acid pathway This pathway produces glucuronic acid which important for: 1 -Synthesis of glycosaminoglycans and vitamin C, L-ascorbic acid (not in human). 2-UDP-glucuronic acid is used for conjucation with many body compounds such as steroids and bilirubin to make them more soulable before excretion. 3 -UDP-glucuronic acid is used for conjucation with many toxic compounds such as phenol to make them less toxic. Fate of glucuronic acid UDP-glucuronic is converted to glucuronate then to L-xylulose which converted to D- xylitol and finally to D-xylulose which join PPP to be completely oxidized. Glycogen Glycogen is hemopolysccharide formed of branchedα D glucose units linked togther by α (1-4) glycosidic bonds linked with α (1-6) at branches. Each branch is made of 12-14 glucose units. Location eb osla nac tub ,selcsum eht dna revil eht yb yliramirp edam si negocylG :.hcamots dna niarb eht nihtiw sisenegocylg yb edam Glycogen is found in the form of granules in the cytoplasm in many cell types, and plays an important role in the glucose cycle. Function: ygrene mret-gnol yradnoces eht sa sevres taht elucelom a si negocylG.lamina ni egarots Glycogen forms an energy reserve that can be quickly mobilized to meet a sudden need for glucose, but less than the energy reserves of T.G. (lipids.( In the liver hepatocytes, glycogen can compose up to 6 -8 % of the fresh weight( 100– 120 g in adult) soon after a meal. Only the glycogen stored in the liver can be made accessible to other organs in the form of glucose as it maintain blood glucose concentration especially during the early stage of fast After 12-18h fasting, liver glycogen is depleted. In the muscles, glycogen is found in a low concentration 350 g about 1 to 2 % of the muscle mass. It acts as a source of energy within muscle itself especially during muscle contractions. Small amounts of glycogen are found in the kidnyes and even smaller amounts in certain glial cells in the brain and WBCs. The uterus also stores glycogen during pregnancy to nourish the embryo. The amount of glycogen stored in the body especially within muscles, liver, and RBCs mostly depends on physical training,basal metabolic rate, and eating habits such as fasting. Glycogenesis It is the synthesis of glycogen in liver and muscle. Glycogen synthesis is endrogenic. This means that glycogen synthesis requires the input of energy. Energy for glycogen synthesis comes from UTP, which reacts with glucose-1phosphate, forming UDP-glucose, in a reaction catalysed by UDP-glucose pyrophosphorylase. The starting point for synthesis of a molecule of glycogen is the protein glycogenin. This is a self-glucosylating enzyme that can create short chains of glucose attached to itself. Once the chains contain more than 10 glucose residues, then they can act as a primer for glycogen synthase to elongate them. Uridine diphosphate glucose (UDP-glucose( is the immediate precursor for glycogen synthesis UDP-glucose is the substrate and UDP is released as a reaction product. UDP-glucose is formed from glucose-1-phosphate and uridine triphosphate (UTP( Glucose Hexokinase in muscle or Glucokinase in liver glucose-6- phosphate glucose-6-phosphate Phosphoglucomutase glucose-1- phosphate Glucose-1-phosphate + UTP UDP-glucose Pyrophosporyl UDP-glucose + Ppi PPi + H2O 2 Pi Spontaneous hydrolysis of the ~P( iPP ni dnob ~P) drives the overall reaction. Glycogenin initiates glycogen synthesis. Glycogenin is an enzyme that catalyzes attachment of a glucose molecule to one of its own tyrosine residue. Glycosidic bond is formed between the anomeric C 1 of the glucose moiety derived from UDP-glucose and the hydroxyl oxygen of a tyrosine. ninegocylg fo niahc-edis UDP is released as a product. Chain elongation Once molecules of proglycogen are established in a cell, further synthesis is controlled by glycogen synthase (key enzyme of glycogeneis. This enzyme elongates existing glucose chains by transferring a new glucose residue onto the free reducing end of the chain. UDP-glucose + glycogen primer glycogen synthase Elongated glycogen primer + UDP Branching enzyme fo tnemgarf lanimret a fo refsnart eht sezylatac : 6-7 glucose residues from elongated chain to next chain forming a new α1-6 glyosidic bond. The branching enzyme can act upon only a branch having at least 11 residues. The new branches are elongated by the glycogen synthase and the process repeated. Glycogenlysis Glycogenolysis “ sa nwonk osla( Glycogenlysis si )” ni esoculg ot negocylg fo noisrevnoc ro nwodkaerb eht.elcsum eht ni dica citcal ro revil eht Glycogen is catabolized by removal of a glucose monomer by phosphorylae ezyme (key enzyme of glycogenlysis) -esoculg ecudorp ot 1-phosphate. Phosphorylae ezyme acts on 1-4 bonds breaking it down by phosphorlysis so it produces glucose-1- phosphate. Phosphorylae ezyme acts on branches containig more than 4 glucose units. When branch contain 4 glucose units ,3 of them are transferred to next branch by transferase enzyme eno tsal gnivael Last glucose unit is attached to the original branch by 1-6 bond which removed by debranching enzyme. This derivative of glucose is then converted to glucose-6-phosphate by mutase enzyme. Fate of glucose-6-phosphate: a) In liver glucose-6-phosphate is converted into glucose by glucose-6-phosphatase. b) In muscle there is no glucose-6-phosphatase so glucose-6-phosphate enter glycolysis to give lactate. So glycogen store is destined for internal use and is not shared with other cells. Regulation of glycogenesis and glycogenlysis: There is a cooredenated regulation of glycogenesis and glycogenlysis as the conditions stimulate glycogenesis inhibit glycogenlysis and vise versa. A) During fasting : Glycogenlysis is stimulated while glycogenesis is inhibited. This provides blood glucose from liver glycogen. B) After meal: Part of absorbed glucose (40 % (goes to circulation to be utilized. The remaiing (60 )%is converted into glycogen in liver So after meals Glycogenesis is stimulated while glycogenlysis is inhibited The principle enzymes controlling glycogen metabolism are glycogen synthase dna phosphorylase. After meal Blood glucose Insulin Glycogen Glycogen - + Phosphorylase Glycogen synthase + - Glucose Glucose Glucagon Epinephrine During fasting Blood glucose Glycogen storage diseases Def: They are group of inherited disorders characterized by deposition of abnormal type and quantity of glycogen in the tissues. Causes: Deficiency of one of enzymes of glycogen metabolism such as glucose-6-phosphatase, debranching enzymes. Types: 1- Type I (Von Gierk’s disease): It is due to deficiency of glucose-6-phosphatase. It is the commonest type and characterized by: 1- Accumulation of large amount of glycogen in liver which leads to disturbance of liver functions. 2- Enlargment of liver (hepatomegally). 3- Fasting hypoglycemia. 4- Ketosis and hyperlipidemia. 5- Hyperuricemia (gout) Glucose-6-phosphatase Glucose-6-phosphate PPP Ribose Uric acid Gout. 2- Type II (Pompe’s disease): Due to absence of lyosomessglucosidase (acid malatase). Acid malatase hydrolyses 1-3% of cellular glycogen. So Glycogen accumulates in lysosomes of all tissue cells including heart cells. Death occurs during first year due to heart failure. 3- Type III (Limit dextrosis) (Cori’s disease): Due to deficiency of debranching enzymes in liver, muscle and heart. 4- Typte IV (Amylopectinosis): Due to absence of branching enzyme. Death occurs during first year due to liver or heart failure. 5- Type V (McArdle’s syndrome): Due to deficiency of muscle phosphorylase. So glycogen is accumulated in muscle during exercise. This leads to decreased energy and muscle cramp after exercise. 6- Type VI (Her’s disease): Due to deficiency of liver phosphorylase. So glycogen is accumulated in liver. 7- Type VII (Tarui’S disease): Due to dificiency of phosphofructokinase in muscle and RBCs. So it leads tohemolytic anemia and muscle cramp. 8- Type VIII: Due to dificiency of liver phosphorylase kinase. Gluconeogenesis Gluconeogenesis is the biosynthesis of glucose, from non carbohydrate sources. The Sources of the carbone skeleton for gluconeogenesis are pyruvate, lactate, glycerol, amino acids alanine and glutamine and propionate in ruminant only. Synthesis of glucose from its precursors is essentially a reversal of glycolysis. Functions of gluconeogenesis: 1. Glucose is fuel source for brain, testes, erythrocytes and kidney medulla since glucose is the sole energy source for these organs. 2. Glucose is the precursor of milk sugar (lactose) in mammary gland. 3 -Glucose is important during low carbohydrate diet or when liver glycogen is depleted. 4 -Gluconeogenesis clears blood from waste products of other tissues as lactate )produced from muscle and RBCs). Location of Gluconeogenesis: fo etis rojam eht ,si revil fo airdnohcotim dna losotyC ,sisenegoenoculg 90 )and kidney( 10.)% 1.Gluconeogenesis from pyruvate gluconeogenesis gluconeogfnesis2 The three irreversable reactions of glycolysis are by passed during gluconeogenesis by using different enzymes. These three are the pyruvate kinase, phosphofructokinase- (PFK1) and hexokinase/glucokinase. In the liver or kidney cortex and in some cases skeletal muscle, the glucose-6-phosphate )G6P) produced by gluconeogenesis can be incorporated into glycogen. Since skeletal muscle lacks glucose-6-phosphatase it cannot deliver free glucose to the blood and undergoes gluconeogenesis exclusively as a mechanism to generate glucose for storage as glycogen. 1 -Pyruvate to Phosphoenolpyruvate (PEP( Conversion of pyruvate to PEP requires the action of two mitochondrial enzymes. The first is an ATP-requiring reaction catalyzed by pyruvate carboxylase, (PC). As the name of the enzyme implies, pyruvate is carboxylated to form oxaloacetate (OAA). The CO2 in this reaction is in the form of bicarbonate (HCO3. )- The second enzyme in the conversion of pyruvate to PEP is PEP carboxykinase (PEPCK). PEPCK requires GTP in the decarboxylation of OAA to yield PEP. Since PC incorporated CO2 into pyruvate and it is subsequently released in the PEPCK reaction, no net fixation of carbon occurs. For gluconeogenesis to proceed, the OAA produced by PC needs to be transported to the cytosol. However, no transport mechanism exist for its ‘direct transfer and OAA will not freely diffuse. Mitochondrial OAA can become cytosolic via three pathways, conversion to PEP, transamination to aspartate or reduction to malate, all of which are transported to the cytosol. If OAA is converted to PEP by mitochondrial PEPCK, it is transported to the cytosol where it is a direct substrate for gluconeogenesis. Transamination of OAA to aspartate allows the aspartate to be transported to the cytosol where the reverse transamination occurs yielding cytosolic OAA. This transamination reaction requires continuous transport of glutamate into, and αketoglutarate out of, the mitochondrion. Therefore, this process is limited by the availability of these other substrates. Mitochondrial OAA can also be reduced to malate by malate dehydrogenase (MDH). The reduction of OAA to malate requires NADH, which will be accumulating in the mitochondrion. The resultant malate is transported to the cytosol where it is oxidized to OAA by cytosolic MDH which requires NAD+ and yields NADH. The NADH produced during the cytosolic oxidation of malate to OAA is utilized during the glyceraldehyde-3-phosphate dehydrogenase reaction of gluconeogenesis. The coupling of these two oxidation-reduction reactions is required to keep gluconeogenesis functional when pyruvate is the principal source of carbon atoms. The conversion of OAA to malate predominates when pyruvate (derived from glycolysis or amino acid catabolism) is the source of carbon atoms for gluconeogenesis. In the cytoplasm, OAA is converted to PEP by the cytosolic version of PEPCK. Pyruvate + ATP + GTP + H2O + iP + PDG + PDA + PEP >——2H+ 2 -Fructose-1-,6bisphosphate to Fructose-6-phosphate: Fructose-1-,6bisphosphate )F1,6BP) is converted to fructose-6-phosphate )F6P) by fructose-1-,6bisphosphatase )F1,6BPase.) N.B. Like the regulation of glycolysis occurring at the PFK-1reaction, the F1,6BPase reaction is a major point of control of gluconeogenesis. 3 -Glucose-6-phosphate )G6P) to Glucose (or Glycogen :) G6P is converted to glucose through the action of glucose-6-phosphatase )G6Pase.) Since the brain and skeletal muscle, as well as most non-hepatic tissues, lack G6Pase activity, any gluconeogenesis that occurs in these tissues is not utilized for blood glucose supply. In the kidney, muscle and especially the liver, G6P be shunted toward glycogen if blood glucose levels are adequate. The G6P produced from gluconeogenesis can be converted to glucose-1-phosphate )G1P) by phosphoglucose mutase (PGM). G1P is then converted to UDP-glucose (the substrate for glycogen synthase) by UDP-glucose pyrophosphorylase. 2 -Gluconeogenesis from Lactate The Cori cycle invloves the utilization of lactate, produced by glycolysis in non-hepatic tissues, (such as muscle and erythrocytes) as a carbon source for hepatic gluconeogenesis. In this way the liver can convert the anaerobic by product of glycolysis, lactate, back into more glucose for reuse by non-hepatic tissues. 3 -Gluconeogenesis from Amino acids All 20 of the amino acids, excepting leucine and lysine, can be degraded to TCA cycle intermediates. This allows the carbon skeletons of the amino acids to be converted to those in oxaloacetate and subsequently into pyruvate. The pyruvate thus formed can be utilized by the gluconeogenic pathway. When glycogen stores are depleted, in muscle during exertion and liver during fasting, catabolism of muscle proteins to amino acids contributes the major source of carbon for maintenance of blood glucose levels. Gluconeogenesis from Amino acid Alanine The glucose-alanine cycle is used primarily as a mechanism for skeletal muscle to eliminate nitrogen while replenishing its energy supply. Glucose oxidation produces pyruvate which can undergo transamination to alanine. This reaction is catalyzed by alanine transaminase, ALT (ALT used to be referred to a serum glutamate-pyruvate transaminase, SGPT). The alanine then enters the blood stream and is transported to the liver. Within the liver alanine is converted back to pyruvate which is then a source of carbon atoms for gluconeogenesis. The newly formed glucose can then enter the blood for delivery back to the muscle. The amino group transported from the muscle to the liver in the form of alanine is converted to urea in the urea cycle and excreted. 4 -Gluconeogenesis from Glycerol: Oxidation of fatty acids yields enormous amounts of energy on a molar basis, however, the carbons of the fatty acids cannot be utilized for net synthesis of glucose. The two carbon unit of acetyl-CoA derived from β oxidation of fatty acids can be incorporated into the TCA cycle, however, during the TCA cycle two carbons are lost as CO2.Thus, explaining why fatty acids do not undergo net conversion to carbohydrate. The glycerol backbone of lipids can be used for gluconeogenesis. This requires phosphorylation to glycerol-3-phosphate by glycerol kinase and dehydrogenation to dihydroxyacetone phosphate (DHAP) by glyceraldehyde-3- phosphate dehydrogenase (G3PDH.) The G3PDH reaction is the same as that used in the transport of cytosolic reducing equivalents into the mitochondrion for use in oxidative phosphorylation. This transport pathway is called the glycerol-phosphate shuttle. The glycerol backbone of adipose tissue stored triacylgycerols is used as a gluconeogenic substrate. 5 -Gluconeogenesis from Propionate (in ruminante only): Oxidation of fatty acids with an odd number of carbon atoms and the oxidation of some amino acids generates as the terminal oxidation product, propionyl-CoA. Propionyl-CoA is converted to the TCA intermediate, succinyl-CoA. This conversion is carried out by propionyl-CoA carboxylase then methylmalonyl-CoA epimerase and finally the vitamin B12 requiring enzyme, methylmalonyl-CoA mutase. The utilization of propionate in gluconeogenesis only has quantitative significance in ruminants. Total energy cost to synthesise glucose from pyruvate Gluconeogenesis converts 2 pyruvates to 1 glucose. The overall reaction is: 2Pyruvate + 4ATP + 2GTP + 2NADH + 6H2O Glucose + 4ADP + 2GDP + 6Pi +2 NAD + +2 H+ Control of gluconeogenesis Hormonal Control Glucagon hormone is released during starvation. This inactivates glycolysis by inhibiting pyruvate kinase by cAMP dependent phosphorylation. Glucagon also increases rate of transcription of phosphoenolpyruvate carboxylase and inhibits transcription of pyruvate kinase. These measures conserves glucose by preventing its metabolism via glycolysis and increase gluconeogeneosis by making the key enzyme phosphoenolpyruvate carboxylase more readily available. Allosteric activation by acetyl CoA: During fasting acetyl CoA concentrations increase due to increased beta-oxidation of fatty acids. Acetyl CoA allosterically activates the gluconeogenic enzyme phosphoenolpyruvate carboxylase and inhibits the enzyme pyruvate dehydrogenase (the enzyme responsible for converting pyruvate into acetyl CoA ready for entry into the citric acid cycle). Therefore, pyruvate will be channelled into gluconeogenesis rather than citric acid cycle. Substrate availability: The presence of glucogenic amino acids promote gluconeogenesis. Alanine inhibits the glycolytic enzyme pyruvate kinase. Citrate inhibits glycolytic enzyme phosphofructikinase and activates the gluconeogenic enzyme fructose 1- ,6bisphosphatase. High levels of citrate signal high levels of citric acid cycle intermediates. Under these conditions converting more glucose to pyruvate ready for entry into the citric acid cycle is unnecessary. Citrate activates the gluconeogenic enzyme fructose 1-,6bisphosphatase so that glucose synthesis is favoured when citric acid cycle intermediates are plentiful. ATP/ADP ratio: High concentrations of ADP and AMP signal that the cell is low on energy. ADP inhibits the gluconeogenic enzymes pyruvate carboxylase and phosphoenolpyruvate carboxylase while AMP inhibits fructose 1-,6bisphosphatase. AMP activates glycolysis through phosphofructokinase. In other words when the energy state is low gluconeogenesis is inhibited while glycolysis is activated. High concentrations of ATP signal that the cell is energy rich. ATP inhibits glycolysis through pyruvate kinase and phosphofructokinase. In other words when ATP is plentiful glucose need not be metabolised. Gluconeogenesis in kidney Although the liver has the critical role of maintaining blood glucose homeostasis and therefore, is the major site of gluconeogenesis, the kidney plays an important role. During periods of severe hypoglycemia that occur under conditions of hepatic failure, the kidney can provide glucose to the blood via renal gluconeogenesis. In the renal cortex, glutamine is the preferred substance for gluconeogenesis. Glutamine is produced in high amounts by skeletal muscle during periods of fasting as a means to export the waste nitrogen resulting from amino acid catabolism. Through the actions of transaminases, a mole of waste ammonia is transferred to αketoglutarate via the glutamate dehydrogenase catalyzed reaction yielding glutamate. Glutamate is then a substrate for glutamine synthetase which incorporates another mole of waste ammonia generating glutamine. The glutamine is then transported to the kidneys where the reverse reactions occur liberating the ammonia and producing αketoglutarate which can enter the TCA cycle and the carbon atoms diverted to gluconeogenesis via oxaloacetate. This process serves two important functions. The ammonia NH3 that is liberated spontaneously ionizes to ammonium ion NH4 +and is excreted in the urine effectively buffering the acids in the urine. In addition, the glucose that is produced via gluconeogenesis can provide the brain with critically needed energy. Galactose metabolism Importance of galactose: A- Synthesis of lactose (milk sugar). B- Synthesis of glycolipids (cerebrosides). C- Synthesis of glycoprotein and proteoglycans. D- Synthesis of glycosaminglycans. Conversion of galactose into glucose occurs in liver. Conversion of glucose into galactose in mammary gland and lactose synthesis: Lactose (milk sugar) is a disscharide formed of B-Dgalactose attached to A-Dglucose by B1-4 bond Galactosemia Def. It is the increase of blood galactose concentration due to inability to metabolize galactose. Cause: Inherited enzyme deficiency of galactokinase, Galactose-1-P uridyl transferase or Epimerase. Effect: Cataract (Opacity of eye lens): Galactose in the eye is reduced by an enzyme, aldose reductase into galacticol which accumulates causing cataract. b) Galactose Aldose reductase Galacticol Cataract b) Liver failure. c) Mental retardation. Galactosuria (excretion of galactose in urine). Fructose Metabolism Sources of fructose a) Hydrolysis of Sucrose (table sugar) give glucose and fructose. b) Honey, fruits and vegetables. Importance of fructose: 1- Energy production 15% of daily energy is derived from fructose. 2- It is the major energy source for spermatozoa in the seminal vesicle. Metabolism of fructose in the liver: A- In liver contains fructokinase and aldolase B enzymes which are important for fructose. B- In extra-hepatic tissues: Because fructokinase is not available in muscle and adipose tissue so fructose is metabolized by hexokinase and other enzymes into pyruvate which oxidized. C- In testes (seminal vesicle), lens, peripheral nerves and renal glomeruli: Glucose is converted into fructose through sorbitol formation. Fructose is the main nutrient for sperms. Deficiency of fructose in semen correlates with male infertility. Genetic disorders of fructose metabolism: A- Essential fructosuria: It is due to deficiency of fructokinase and it leads to no serious condition only excess accumulated fructose is lost in urine. B- Hereditary fructose intolerance: It is due to deficiency of aldolase-B which leads to accumulation of fructose-1- phosphate It leads to: 1- Damage of liver and kidney tissues which leads to liver and kidney failure. 2- Inhibition of phosphorylase which leads to inhibition of glycogenlysis and hypoglycemia. Mechanisms of blood sugar regulation Blood sugar regulation is the process by which the body maintains the blood sugar, levels, primarily glucose, within normal range. Plasma glucose levels: Fasting blood glucose level is 65-110 mg/ dl. One hour after carbohydrate meal it is 120-150 mg/ dl. Two hour after carbohydrate meal it is 65-140 mg/ dl. Sources of blood glucose: A- Dietary carbohydrate (absorbed glucose after digestion of carbohydrate). B- Liver glycogen through glycogenlysis. C- Amino acids and other metabolites through gluconeogenesis. Regulation of blood glucose level: The aim of the regulation of blood glucose level is to maintained the blood glucose level within narrow range 65-150 mg/ dl in order to keep the body in homeostasis because: 1- Hypoglycemia (low blood glucose level) cause impairment of cerebral function. 2- Hyperglycemia (high blood glucose level) can cause cerebral dysfunction by its effect on extra cellular osmolality. A- Hormones regulation of blood glucose level Effect on Tissue of Hormone Metabolic Effect Blood Origin Glucose 1) Enhances entry of glucose into cells. 2) Enhances storage of glucose as glycogen, or conversion to fatty acids. Pancreatic 3) Enhances synthesis of fatty acids and proteins. Insulin Lowers B-cells 4) Suppresses breakdown of proteins into amino acids, 5) Suppresses breakdown of adipose tissue into free fatty acids. 1) Suppresses glucagon release from α cells Pancreatic Somatostat (acts locally). Sigma Lowers in 2) Suppresses release of Glucagon, Pituitary cells tropic hormones, gastrin and secretin. Pancreatic 1) Enhances release of glucose from glycogen. Gucagon Raises α cells 2) Enhances synthesis of glucose from amino acids or fatty acids. 1)Enhances release of glucose from glycogen. Epinephri Adrenal 2) Enhances release of fatty acids from adipose Raises ne medulla tissue. Adrenal 1)Enhances gluconeogenesis. Cortisol Raises cortex 2) Antagonizes Insulin. Anterior 1) Enhances release of cortisol; ) Enhances ACTH Raises pituitary release of fatty acids from adipose tissue. Growth Anterior Antagonizes Insulin Raises Hormone pituitary 1)Enhances release of glucose from glycogen Thyroxine Thyroid Raises 2) Enhances absorption of sugars from intestine B- Hepatic regulation Liver plays important role in keeping blood glucose level within range. 1- During fasting: a- Glucose production through glucogenlysis and gluconeogenesis. b- Conversion of FA released from adipose tissue to ketone bodies which provide energy supply for brain and other tissues. 2- After carbohydrate meal: Glucose is transported in portal blood to liver. 40% of blood glucose pass to extra-hepatic tissues. 60% of blood glucose reach liver and converted to glucose-6-phosphate by glucokinase which subsequently converted to glycogen or fatty acids. C- Renal regulation: Circulating blood glucose is filtered in glomerular filtrate and reabsorbed again by certain tubular enzymes to save blood glucose from loss in urine. If blood glucose level exceeds certain limits (180 mg/ dl) glucose will increase in glomerular filtrate and exceeds the capacity of tubular enzymes. Thus glucose appears in urine (glycosuria). This limit is called renal threshold for glucose reabsorption. Renal threshold for glucose reabsorption is the blood glucose level above which glucose appears in urine. Low renal threshold: where glucose appear in urine even when blood glucose level is low (may be 100mg/dl). This occurs in: A- Normally in some persons due to defective tubular enzymes for glucose Reabsorption (diabetes innocence). B- In 20% of pregnant females. High renal threshold: where glucose appears in urine in concentration above the average (renal threshold) which may reach 220 mg/dl or more. This occurs in: A- Elderly people due to reduced glomerular filtration rate. B- in case of diabetes associated with renal damage. Variation in blood glucose Hypoglycemia Def. It is decrease of blood glucose concentration below normal fating level (65 mg/dl) Symptoms and Effect of A- It causes cerebral dysfnction. Anxiety, tremors, palpation, sweating, hunger, headache, confusion, slurred speech. B- Sever and prolonged hypoglycemia leads to coma and then death. Mechanism that corrects hypoglycemia Hypoglycemia stimulates: A- Secretion of Glucagon which stimulates glycogenlysis to increase blood glucose. B- Secretion of epinephrine which stimulate glycogenlyis to increase blood glucose. C- Secretion of Growth hormone which antigonize the effect of insulin. D- Secretion of ACTH which stimulates Glucocorticoids which stimulates gluconeogenesis to increase blood glucose. Types of hypoglycemia A- fasting hypoglycemia It occurs due to inability to maintain normal glucose level in fasting state which my be due to organ diseases such as: 1- Pancreatic disease (pancreas tumor) which leads to insulinomia (increased insulin secretion). 2- Liver diseases which leads to decrease glycogenlysis and gluconeogenesis. 3- Adrenocortical diseases which decreased the secretion of epinephrine and glcocorticoids which decrease glycogenlysis and gluconeogenesis. B- Simulative hypoglycemia It is occurs due to some stimuli after taking meals. Causes 1- Drugs and poisons such as over dose of insulin, sulphonylurea, chloroform, phosphorus and alcohol. 2- Postgastrectomy which increase the absorption of glucose and increase insulin secretion. 3- Leucine hypersensitivity which stimulate insulin secretion. 4- Inborn errors of metabolism such as galactosemia and hereditery fructose Intolerance which inhibit glycogenlysis. Hyperglycemia Def. It is the rise of blood glucose above normal level. Causes 1- Diabetes mellitus the most common cause. 2- Intravenous fluid containing glucose. 3- Sever stress. 4- Cerebro-vascular accidents. 5- Disturbance in hyperglyemic hormones. Diabetes mellitus Def. It is a state of chronic hyperglycemia accompanied by glycouria. N.B. Diabetes means increase urine volume. Types of diabetes mellitus: Two types 1-Type I Diabetes mellitus (Insulin-dependent diabetes mellitus) (IDDM). 2- Type II Diabetes mellitus (Non Insulin-dependent diabetes mellitus) (NIDDM). Insulin-dependent Non Insulin-dependent diabetes mellitus diabetes mellitus Synonym Juvenile-onset diabetes Adult-onset diabetes Age During childhood (young) After 35 years (old) Nutritional status at Undernourishment (thin) Obesity time of onset of disease Prevalence 10-20% of diagnosed 80-90% of diagnosed diabetic cases diabetic cases Genetic predisposition Moderet Very strong Defect or deficiency B-cells destruction (no Inability of B-cells to insulin) produce insulin or insulin resistance Ketosis common Rare Plasma insulin Low or absent Normal or high Acute complication Ketoacidosis Hyperosmolar comma Oral hypoglycemia drug No response Responsive Treatment with insulin Always necessary Usually not required Biochemical disturbance of diabetes mellitus Insulin deficiency causes disturbance in carbohydrate, lipid and protein metabolism. A- Carbohydrate metabolism: Insulin deficiency leads to decrease glucose uptake by tissues which decrease glucose oxidation, while increased glconeogenesis and glycogenlysis which lead to: 1- Decreased intracellular glucose which leads to hunger which leads to polyphagia. 2- Increased blood glucose which increased plasma osmolality which leads to dehydration which leads to symptoms and signs of dehydration. Dehydration of brain cells leads to hyperglycemic, hyperosmolal coma. Dehydration of body cells which leads to sense of thirst which leads to polydepsia. 3- Glycosuria which leads to excessive and frequent urination (polyurea) which leads to loss of water soluble vitamins and minerals. B- Protein metabolism Insulin deficiency leads to increased protein breakdown and stimulation of Gluconeogensis which results in 1- Phosphate release which result in hyperphosphatemia. 2- Excessive breakdown of tissue proteins causing muscle wasting. 3- Decreased antibody formation causing low resistance and infection. 4- Poor healing of wounds. C- lipid metabolism: Insulin deficiency leads to excessive lipolysis in adipose tissue which leads to mobilization of free fatty acids and glycerol in the blood then to the liver and other tissues this leads to: 1- Loss of weight. 2- Hyperlipedimia which leads to atherosclerosis. 4- Fatty liver due to restrification of fatty acid with glycerol to give TG. 5- Ketone bodies formation which leads to ketonemia and ketosis which leads to coma (ketotic coma) and hyperlkalemia D- Microangiopathy: It is a degenration that affects small blood vessels as capillaries especially those of The kidney and retina of the eyes. Thus the two common chronic complication of diabetes milletus are renal failure and blindness. Types of diabetes 1- Diabetes mellitus due to defective insulin action. 2- Diabetes insipidus it is hereditary disease due to defective action of antidiuretic hormone. 3- Diabetes innocence (renal diabetes) it is due to low renal threshold for glucose reabsorption. 4- Bronze diabetes it is a hereditary disease caused by excessive absorption of iron and its precipitation in tissues as: a- Skin causing its bronze discoloration. b- Pancreas leads to diabetes mellitus. c- Liver leads to hepatic cirrhosis. Glycosuria Def. It is the presence of glucose in urine in amount detectable by ordinary method. Causes 1- Diabetes mellitus it is the common cause of glycosuria about 90% of all types. 2- Excess excretion of diabetogenic hormones such as growth hormone, Glucocorticoids, epinephrine and glucagon. 3- Diabetes innocence (renal glyosuria) due to abnormal low renal threshold for glucoseabsorption 4- Pregnancy glycosuria it occurs in 20% of all pregnancies. It is due to increase of glomerular filtration rate by about 50% during pregnancy.