BS31013 Lecture 1 2024-25 Membranes Transport PDF
Document Details
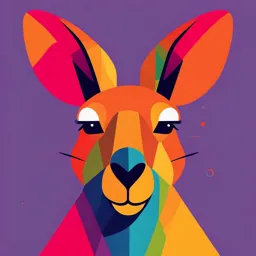
Uploaded by ShinyLongBeach6025
University of Dundee
2024
Stephen Kelley
Tags
Summary
This lecture covers membrane transport mechanisms, including passive diffusion, facilitated diffusion, and active transport. It discusses the role of electrical and concentration gradients in solute movement across cell membranes, and provides examples relevant to pharmacology.
Full Transcript
MEMBRAN E TRANSPOR T Stephen Kelley, PhD, FHEA, FBPhS Senior Lecturer in Pharmacology Learning outcomes Understand the regulation of normal membrane function and the physiological principles that underly this. Understand the role of electrical and concentration g...
MEMBRAN E TRANSPOR T Stephen Kelley, PhD, FHEA, FBPhS Senior Lecturer in Pharmacology Learning outcomes Understand the regulation of normal membrane function and the physiological principles that underly this. Understand the role of electrical and concentration gradients in transport of solutes across the membrane Understand the mechanisms involved with passive diffusion, aqueous diffusion, facilitated diffusion and active transport, including primary active transport and secondary active transport Be able to provide physiological examples of primary active transport and secondary active transport in the context of a physiological system Recommended reading: Total body water Body fluid compartments for a human adult weighing 70 kg (42L) Extracellular Intracellular Interstitial water Plasma water (13 L) Intracellular (3 L) [Na+] = 145 mM water (25 L) [K+] = 4.5 mM [Na+] = 15 mM [Cl-] = 116 mM [Na+] = 142 mM [K+] = 120 mM [protein]= 0 mM [K+] = 4.4 mM [Cl-] = 20 mM Osmolity = [Cl-] = 102 mM [protein]= 4 mM 290 mOsm [protein]= 1 mM Osmolity = Osmolity = 290 mOsm Epithelial cells 290 mOsm Significant Transcellular water concentration (1 L) gradients between Variable concentrations intracellular and Capillary extracellular fluid endothelium Ways in which small molecules can cross cell membranes 1. Passive Diffusion directly through the lipid or through aqueous pores formed by aquaporins that transverse the lipid bilayer. Many lipid soluble molecules cross cell membranes in this way. 2. Aqueous Diffusion via a channel that transverses the plasma membrane. Does not require energy, but does require a concentration gradient 3. Facilitated Diffusion via specialised carrier proteins that bind the drug on one side of the bind molecule on one side of the membrane then change conformation and release on the other side. Does not require energy, but does require a concentration gradient 4. Active Transport via specialised carrier proteins Requires Energy and can move molecules against the concentration gradient. 5. Endocytosis (pinocytosis)- invagination of a part of the membrane. The molecule is encased in a small vesicle then ‘released’ inside the cell. Graphic summary of transport mechanisms Aqueous High solute diffusion Facilitated Active transport down a against a concentrati concentration diffusion down a concentration concentration gradient via a gradient via on channel gradient via carrier protein carrier protein. Requires energy. Passive diffusion N down a Low solute H H H concentration HH gradient. H O H H Lipophilic concentrati Solute transport across cell membranes For passive diffusion and aqueous diffusion (i.e., non-coupled transport), a solute moves down its electrical and/or chemical gradient. The membrane will have to be permeable; either the solute will have the appropriate lipophilicity to simply cross the membrane or channels will have to be present in the membrane. Note that this is non-coupled transport. That is, the movement of the solute is not dependent upon the movement of another solute or a chemical reaction taking place. Some modelling equations and essentially a recap of earlier concepts you should have seen before. Electrochemical potential energy difference = Chemical potential energy difference + electrical potential energy difference OR Don’t let this equation scare you! All it is trying to model is the differences in solute concentration and differences in charged particles concentration across a membrane affecting the driving force () that determines passive diffusion and aqueous diffusion. In a state of equilibrium, the potential differences are equal but opposite At equilibrium: Vm: membrane potential R: Gas constant T: absolute temperature The Nernst z: ionic valence F: Faraday constant equation- remember this? Calculation of EK using the Nernst equat Net driving force in volts is Vm – Ex So, at resting potential the driving force of K+ is (-70mV) – (-88 mV) = 17.7 (outward), so not much. However, at the peak of an action potential ~+30 mV it is 117.7 (outward), much greater. Which is why K+ floods into the cell during repolarisation! See how this What about diffusion of electrically neutral solutes? For this we use Fick’s Law: ([ Where is the flux or how fast the solute X moves across the membrane (i.e., the number of moles of X crossing the membrane per unit of time). The higher the lipid-water partition coefficient of the solute the easier it is for the solute dissolve in the membrane. Once in the lipid tails, the faster the solute moves through them is determined by the diffusion coefficient (D). Smaller membrane thickness (m) also helps this movement. We combine these 3 factors to arrive at the permeability coefficient, ,of the solute: = The lipid-water partition coefficient (KP), logP Helps measure how lipid or water soluble a drug is. This is determined by how readily a drug partitions between hexane and water. For hydrophilic molecule: Kp < 1 For hydrophobic molecule: Kp > 1 These can be difference of 10 orders of magnitude, so for ease of comparison, we use log10 of Kp, and we will call this logP. This is the term that is used most often in pharmaceutical chemistry Simple passive diffusion- drug example Compartment 1 (Extracellular) Membrane Compartment 2 C is the (Intracellular) transmembrane m oncentration of DrugConcentration of Drug concentration gradient Cm Drug with high lipid solubility Ganaxolone C1 logP = 5.0 C2 Drug with low lipid solubility Mannitol C1 logP = - Cm C2 Application to pharmacology: pH, ionisation and diffusion of drugs across the plasma membrane Many drugs are weak acids or weak bases These drugs will exist in both their ionised and un- ionised forms The proportion of ionisation of a drug depends upon both the pKa of the drug and the local pH The pKa = pH at which 50% of drug is ionised and 50% un-ionised For many drugs the non-ionised form can AH permeate the membrane A +H - + For acids the ionisation reaction is BH+ B + H+ For bases the ionisation reaction is Application to pharmacology: pH trapping across plasma membranes -aspirin (acetylsalicylic acid) pKa = 3.5 99% un-ionised AH A- + H+ 1% ionised Stomach pH 1.5 Gastric Mucosal Barrier Plasma pH 7.4 Negatively charged AH A- + H + aspirin diffuses across the membrane of the gastric mucosa 0.01% un-ionised and is trapped in the 99.99 % ionised Good plasma Principal Blood brain barrier sites of carrier Gastrointestinal mediated Tract transport Placenta (both facilitated Renal Tubule diffusion and active transport) Biliary Tract The Importance of Transporters Intestinal solute carrier proteins (e.g. secondary active transport) vital to the absorption of electrolytes, macro- and micro-nutrients and vitamins also contribute to drug absorption From: Anderson and Thwaites (2010). Physiology 25: 364-377 Carrier mediated (facilitated diffusion or active transport) vs passive diffusion [ 𝑆 ] ∗ 𝑉 𝑚𝑎𝑥 𝑉= 𝐾 𝑚 +[ 𝑆] Solute X uptake into Carrier-mediated Follows the same process as Michaelis-Mention kinetics (because if involves a protein Diffusion in finite amounts!) [ 𝑋 ] ∗ 𝐽 𝑚𝑎𝑥 cell 𝐽 𝑥= 𝐾 𝑚 +[ 𝑋 ] Solute X is the solute concentration concentration that where is ½. The lower the (arbitrary units) higher the affinity of the solute for the transporter. Transporters in Facilitated Diffusion solute Transporter Transporter Hydrophilic, polar molecules can enter the cell through specialised carrier proteins Does not require energy Can show saturation kinetics The glucose transporters (e.g., GLUT1) are members of the SLC2 superfamily (SLC=solute carrier) Facilitated diffusion of glucose by GLUT1 is responsible for insulin secretion by pancreatic beta cells. Joseph C. Koster et al. Diabetes 2005;54:3065-3072 GLUT2 and GLUT5 are responsible for facilitated diffusion of fructose and glucose in the gut Glucose and galactose are absorbed by secondary active transport mediated by SGLT1; fructose by facilitated diffusion mediated by GLUT5. Exit for all monosaccharides is mediated by facilitated diffusion by GLUT2 Substrate must be: (i) A hexose in the D- Glucose 1 Glucose conformation (or (or (ii) One that can galactose) galactose) SGLT1 form a 2 Na+ pyranose ring 2K+ (SLC5A1) GLUT2 (SLC2A2) 3 Na+ GLUT5 (SLC2A5) Fructose Fructos Na+/ e H 2O K+ATPase Transporters in active transport solute Transporter Transporter ADP ADP ATP ATP Hydrophilic, polar molecules can enter the cell through specialised carrier proteins Requires energy Is capable of moving molecules against a concentration gradient Primary active transport- the Na+/K+ ATPase pump Na+/K+ ATPase is the most important primary Helps maintain the normal trans- membrane electrochemical gradients of active transporter Na+ and K+ in association with the membrane permeability properties. because it sets up the concentration gradients for secondary active transport mechanisms! Na+ K+ Na+ K+ Na+ Primary active transport is moving a solute against Na + its electrochemical gradient K+ + via ATP hydrolysis. Na + K Na + Secondary active transport is moving a solute against its Energy in the Na gradient is used to drive secondary active electrochemical gradient by transport systems for sugars and amino acids coupling an ‘uphill’ movement with the 1) Starting conformation- High affinity for Na+, low affinity for K+, Binding on one molecule of ATP. 2) Substrate binding - Binding of 3 Na+. 3) Phosphorylation of the enzyme - - Gamma phosphate of ATP is transferred to an aspartyl - ß-carboxyl group with release of ADP. 4-6) Transformation of the phosphoenzyme - Transition from an ADP-sensitive to an ADP- insensitive phosphointermediate which undergoes spontaneous hydrolysis that is stimulated by the presence of K+. Transition involves a reduced affinity for ADP and a drastic change in Na+ and K+ affinities. 7) Hydrolysis of the phosphoenzyme - phosphate is released into intracellular space. The hydrolysis of the aspartyl- phosphate bond is ouabain sensitive. 8) Return to the native enzyme form - the pump undergoes a conformation change from the occluded E2-K form (Step 5) to the Figure from Boron and Boulpaep (2017) Transport Mechanism Studies with the reconstituted enzyme and use of radiotracer labelled Na+ and K+ has shown that the pump can operate in at least five modes. This is largely because a number of the steps in the reaction cycle are reversible. · Normal mode (Na+/K+ exchange) Na+ K+ · Reverse mode · Na+-Na+ exchange (1:1) Na+ K+ · Uncoupled Na+ efflux · K+-K+ exchange (1:1) Nai = 0 K+ ATP / Pi K Na,K-ATPase – a member of the P-type ATPases K+ K+ Catalytic subunit - possesses binding sites for: a Na, K, ATP and Mg and cardiac glycosides (e.g. ouabain) Intrinsic ATPase activity 4 isoforms (α1, α2, α3 & α4) Molecular size ~ 112kDa Developmentally regulated and expressed in a tissue-specific manner Regulatory subunit Three isoforms (ß1, ß2 & ß3) Heavily glycosylated (28% w/w) – Protein moiety ~35kDa Crucially required for full enzyme activity, but also for - b Enzyme assembly Intracellular transport of complete enzyme molecule Stability of the a subunit In rat skeletal muscle ß1 and ß2 expressed in fibre-specific manner. ß2 also known as AMOG. Small auxillary protein (8-14 kDa) belonging to the g FXYD protein family – named after an invariant motif Single-spanning membrane peptide. Evidence for multiple isoforms of Na,K-ATPase Sensitivity to cardiac glycoside different in different tissues (compare brain and kidney) Kidney Brain ATPase ATPase activity activity [Ouabain] [Ouabain] Tissue specific antibodies against purified enzyme. Antibodies against one tissue only partially inhibited Na,K-ATPase activity of other tissue. Tissue specific expression of a subunit mRNA's a1 a2 a3 a4 Kidney +++ - - - Brain + +++ +++ - Heart +++ ++ -/+ - Sk. Muscle + +++ - - Sm. Muscle ++ +/- +/- - Liver ++ - - - Fat + ++ - - Testis + - - +++ a1 - Ubiquitous expression- contains the binding site for drugs such as digoxin a2 - excitable tissues/insulin responsive tissues a3 - excitable tissues a4 – only expressed spermatozoa + and - indicate relative expression down the column not across Application to pharmacology- Digoxin Obtained from Foxglove, Digitalis, plants. Its medicinal properties were noted by William Withering in 1775 to alter heart rate. Digoxin is Digoxin an inhibitor of the Na+/K+ ATPase pump and binds to the alpha subunit. This causes an increase in Na+/K+ intracellular Na+. The increased Na+ ATPase pump Na+/Ca2+ exchanger concentration reduces the action of 2K+ 3Na+ Ca2+ the Na+/Ca2+ exchanger in the resulting in increased intracellular Ca2+ which is then stored in the sarcoplasmic reticulum. The Ca2+ is 2K+ 3Na+ Ca2+ released during a cardiac action potential thus increasing the force ↑[Ca2+]i of a contraction Depolarisation= disturbs cardiac rhythm at high doses. Note that digoxin has a narrow therapeutic window. Application to pharmacology: P-glycoprotein transporters- another primary active transport system Adapted from Syvanen and Eriksson 2012 Multidrug transmembrane transporters (ATP dependent) Responsible for multi-drug resistance Functions in various parts of the body include: Liver: Transporting drugs into bile for elimination Kidneys: Pumping drugs into urine for excretion Placenta: Transporting drugs back into maternal blood Intestines: Transporting drugs into intestinal lumen, reducing drugs absorption into the blood Brain capillaries: Pumping drugs back into the blood, limiting distribution in the brain Primary active Secondary active Transport Transport- symport Na+/K+ pump SGLT1-3 Cell membrane ATP Secondary active Transport- antiport Na-Ca exchanger Primary and Secondary Transport and Na+ Absorption (1) From Boron and Boulpaep (2017) Medical Physiology (3 rd ed.) Na+/glucose and Na+/amino acid cotransport are the major mechanisms of postprandial Na+ absorption in the jejunum o Both are examples of secondary active transport and are electrogenic, as is the Na+/K+ ATPase – collectively the overall transport of Na+ generates a transepithelial potential (VTE) in which the lumen is negative – this drives the parallel absorption of Cl- Primary and Secondary Transport and Na+ Absorption (2) From Boron and Boulpaep (2017) Medical Physiology (3rd ed.) Na+/H+ exchange in the jejunum occurs at both the apical (NHE2 and NHE3) and basolateral (NHE1) membranes, but only NHE2 (not shown) and NHE3 contribute to transepithelial movement of Na+ (and the regulation of intracellular pH). NHE1 is a ‘cellular pH housekeeper’ o Exchange at the apical membrane, in the jejunum, is stimulated by the alkaline environment of the lumen (i.e. high pH = low proton concentration) due to presence of bicarbonate from the pancreas Primary and Secondary Transport and Na+ Absorption (3) From Boron and Boulpaep (2017) Medical Physiology (3 rd ed.) Na+/H+ and CI-/HCO3- exchange in parallel occurs in the ileum and proximal colon and is the primary mechanism of Na+ absorption in the interdigestive period, but does not contribute greatly to postprandial absorption o Absorption is electroneutral o Regulated by intracellular cAMP, cGMP and Ca2+, all reduce NaCl absorption o Reduction in NaCl absorption is a cause of diarrhoea (e.g. secretory diarrhoea due to infection with E. coli – heat stable enterotoxin from which activates adenylate cyclase and increases intracellular cAMP)