Botany (Plant Physiology) PDF
Document Details
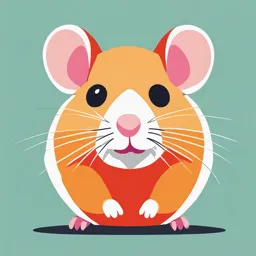
Uploaded by PreferableShakuhachi6937
Tags
Summary
This document provides an introduction to botany, focusing on plant physiology. It explores the role of water in plant life, including water uptake, loss, and its properties. It also covers water potential and different types of solutions within the context of plants.
Full Transcript
# BOTANY (PLANT PHYSIOLOGY) ## Introduction Plant physiology is the essential core curriculum for every botanist. Plants have special needs because they are usually fixed to a single location for their entire lifetime. They must cope with widely disparate and constantly changing environmental cond...
# BOTANY (PLANT PHYSIOLOGY) ## Introduction Plant physiology is the essential core curriculum for every botanist. Plants have special needs because they are usually fixed to a single location for their entire lifetime. They must cope with widely disparate and constantly changing environmental conditions. Physiological adaptations play a significant role in their evolutionary struggle for life. The science of physiology is about the function of living things. It encompasses functions from the level of atoms and small molecules to that of the whole organism. At the level of nucleic acids and enzymes, structure and function are so intimately linked that the physiologist is equally concerned with their structure and function. The core of life is found in the biochemical, enzymatically controlled reactions of protoplasm. These reactive components are in water solutions. Macromolecules like protoplasm (including protein enzymes, nucleic acids, starch and pectin) form a unique structure by associating with water molecules. These structures have a colloidal state. ## Water and Cell Water plays a crucial role in plant life. For every gram of organic matter produced by the plant, approximately 500 g of water is absorbed by the roots, transported through the plant body, and ultimately lost to the atmosphere. Even slight imbalances in this flow of water can lead to water deficits, which can severely impact cellular processes. Plants must delicately balance their water uptake and loss, a serious challenge for land plants. To carry on photosynthesis, plants need to draw carbon dioxide from the atmosphere, which exposes them to water loss and the risk of dehydration. ### Importance of Water for Plants Water makes up most of the mass of plant cells. Looking at microscopic sections of mature cells, we can readily see that each cell contains a large, water-filled vacuole. Usually, the cytoplasm only makes up 5 to 10% of the cell volume; the remainder is vacuole. Water typically makes up 80 to 95% of the mass of growing plant tissues. Common vegetables like carrots and lettuce contain 85 to 95% water. Wood, made mostly of dead cells, has a lower water content. Sapwood, which transports water through the xylem, contains 35 to 75% water, and heartwood has a slightly lower water content. Seeds, with a water content of 5 to 15% are among the driest of plant tissues, yet before germinating, they must absorb a considerable amount of water. Water is the most abundant and arguably the best solvent known. As a solvent, it creates the medium for the movement of molecules within and between cells, greatly influencing the structure of proteins, nucleic acids, polysaccharides, and other cell constituents. Water creates the environment in which most of the biochemical reactions of the cell occur, and it directly participates in many essential chemical reactions. Plants continuously absorb and lose water. Most of the water lost by the plant evaporates from the leaf as the CO2 needed for photosynthesis is absorbed from the atmosphere. On a warm, dry, sunny day, a leaf will change up to 100% of its water in a single hour. During a plant's lifetime, water equivalent to 100 times the fresh weight of the plant may be lost through the leaf surfaces. Such water loss is called transpiration. In addition to this transpiration, the stream of water taken up by the roots is an important means of ringing dissolved soil minerals to the root surface for absorption. Of all the resources that plants need to grow and function, water is the most abundant and at the same time, the most limiting for agricultural productivity. The fact that water is limiting is the reason for the practice of crop irrigation. Water availability also limits the productivity of natural ecosystems. Thus an understanding of the uptake and loss of water by plants is very important. ### Water Properties The water molecule consists of an oxygen atom covalently bonded to two hydrogen atoms. The two O-H bonds form an angle of 105 degrees. Because the oxygen atom is more electronegative than hydrogen, it tends to attract the electrons of the covalent bond. This attraction results in a partial negative charge at the oxygen end of the molecule and a partial positive charge at each hydrogen. Water is a polar molecule, meaning that it has a surface charge. Obviously, water is a dipolar substance in that the hydrogen pole is positively charged, and the other pole is negatively charged due to the electrophilic (electron-attracting) properties of oxygen. Water molecules at an air-water interface are more strongly attracted to neighboring water molecules than to the gas phase in contact with the water surface. As a consequence of this unequal attraction, an air-water interface minimizes its surface area. To increase the area of an air-water interface, hydrogen bonds must be broken, which requires an input of energy. The energy required to increase the surface area is known as surface tension. Surface tension not only influences the shape of the surface, but also may create a pressure in the rest of the liquid. As we will see later, surface tension at the evaporative surfaces of leaves generates the physical forces that pull water through the plant's vascular system. The extensive hydrogen bonding in water also gives rise to the property known as cohesion, the mutual attraction between molecules. A related property, called adhesion, is the attraction of water to a solid phase such as a cell wall or glass surface. Cohesion, adhesion, and surface tension give rise to a phenomenon known as capillarity, the movement of water along a capillary tube. In a vertically oriented glass capillary tube, the upward movement of water is due to the attraction of water to the polar surface of the glass tube (adhesion) and the surface tension of water, which tends to minimize the area of the air-water interface. Together, adhesion and surface tension pull on the water molecules, causing them to move up the tube until the upward force is balanced by the weight of the water column. The smaller the tube, the higher the capillary rise. ## Water Potential The chemical potential of water represents the free energy of water. All living things, including plants, require a continuous input of free energy to maintain and repair their highly organized structures, as well as to grow and reproduce. Processes such as biochemical reactions, solute accumulation, and long-distance transport are all driven by an input of free energy into the plant. The chemical potential of water is a quantitative expression of the free energy associated with water. In thermodynamics, free energy represents the potential for performing work. Note that chemical potential is a relative quantity: it is expressed as the difference between the potential of a substance in a given state and the potential of the same substance in a standard state. The unit of chemical potential is energy per mole of substance (J mol¯¹). For historical reasons, plant physiologists have most often used a related parameter called water potential, defined as the chemical potential of water divided by the partial molal volume of water (the volume of one mole of water). Water potential is a measure of the free energy of water per unit volume (J.m³). These units are equivalent to pressure units such as the Pascal, which is the common measurement unit for water potential. The major factors influencing the water potential in plants are concentration, pressure, and gravity. Water potential is symbolized by Yw (the Greek letter Psi), and the water potential of solutions may be dissected into individual components, usually written as the following sum: S +Ψ P g The terms Ys, Yp, and Yg denote the effects of solutes, pressure, and gravity, respectively, on the free energy of water. The reference state used to define water potential is pure water at ambient pressure and temperature. Let's consider each of the terms on the right-hand side of Equation. Water potential is referred to as the difference between the free energy of water in a cell and of pure water at atmospheric pressure. Water always moves from a region of higher water potential to one of lower water potential. It is represented by the Greek letter Ψw (Psi) and is measured by 'bars,' one bar being close to one atmosphere of pressure (1 bar = 0.987 atmosphere of pressure). At atmospheric pressure, the water potential of pure water is 0 (zero). Hence all solutions at atmospheric pressure have lower water potential than water, i.e., they have a negative value. There are two basic factors that affect the water potential: - Effect of Dissolved Solute on Water Potential Pure water at atmospheric pressure has a zero water potential. Addition of solutes lower the water potential Y of 0.1 M solution containing solutes = -2.3 bars. Since the water solution in soil containing dissolved minerals has a higher potential and the plant cells of roots have a lower water potential, water from the soil moves into the root cells of the plant. - Effect of External Pressure on Water Potential The increase in pressure, increases the water potential. If a pressure greater than atmospheric pressure is applied to pure water or a solution, the water potential can be raised from zero to a positive value. The term Yp is the hydrostatic pressure of the solution. Positive pressures raise the water potential; negative pressures reduce it. Sometimes Yp is called pressure potential. The positive hydrostatic pressure within cells is the pressure referred to as turgor pressure. Pressure potential may be positive or negative; the higher the pressure, the greater potential energy in a system, and vice versa. Therefore, a positive Ψp (compression) increases Yw, while a negative Yp (tension) decreases Yw. Positive pressure inside cells is contained by the cell wall, producing turgor pressure in a plant. Turgor pressure ensures that a plant can maintain its shape. A plant's leaves wilt when the turgor pressure decreases and revive when the plant has been watered. ## Solutions Many types of solutions can be formed by dissolving one substance (the solute) in another substance (the solvent). Solutions can be formed from all three states of matter (solid, liquid and gas) and in various combinations. According to the size of solute particles, solutions can be divided into three types: - *Electrolyte solution (true solution)*: In which the substance is broken down into molecules or ions which cannot be seen by any optical means since their diameter is less than 0.001 micron (micron = 1/1000mm). Such a system is stable. For example, sodium chloride or sucrose dissolves freely in water forming a true solution. Sodium chloride splits up into ions, while sucrose breaks down into molecules. In both cases, the dissolved substances can be crystallized out from their solutions. - *Suspension and Emulsion*: In which the substance is broken down into particles which can be seen by ordinary microscope since their diameter is more than 0.1 micron. They are unstable systems since the two original components are separated within relatively short periods of time. If it is liquid, the system is an emulsion. For example, If we stir some silt into water, we get a suspension which is not stable since the silt particles slowly settle down. If we vigorously shake two immiscible liquids such as water and oil, we get an emulsion which is also unstable since the two components separate within a short period unless a third component called an emulsifier is added to the system, like sodium hydroxide. - *Colloidal System*: Which is intermediate in its properties between true solutions on one hand and suspension or emulsion systems on the other hand. The dispersed particles are not separate molecules nor ions but particles (aggregate of molecules), the diameter of which ranges between 0.1 micron and 0.001 micron. Unlike suspension and emulsion, the colloidal system is relatively stable and their particles cannot be seen under ordinary microscopes. The colloidal particles can be detected by the electron microscope or by the ultramicroscope. Unlike true solutions, the colloidal systems do not form crystals. In general, if the dispersed particles fall within the range of 0.001 - 0.1 micron in diameter, the system is considered a colloidal, if larger than this, a suspension or an emulsion, if smaller a true solution. In dealing with the colloidal systems, one always refers to the solvent as the dispersion medium or the continuous phase and the solute particles as the dispersed phase or the discontinuous phase. Colloidal solutions can be classified into lyophobic (solvent fearing) in which no affinity exist between the particles of the dispersed phase and the dispersion medium and lyophilic (solvent loving) in which there is an appreciable affinity between the particles of the dispersed phase and the dispersion medium. If the dispersion medium is water, the corresponding terms hydrophobic (water fearing) and hydrophilic (water loving) are employed. Some refer to the lyophobic as suspensoid and lyophilic as emulsoid. Protein, gelatin, starch, agar, glue and gum sols are examples of hydrophilic systems. On the other hand, most colloidal systems composed of metallic substances dispersed in water are examples of hydrophilic sols, e.g., sols of aluminum hydroxide Al(OH)3, ferric hydroxide Fe(OH)3, sols of gold (Au) and silver (Ag). ## The Plant Cell Plants are multicellular organisms composed of millions of cells with specialized functions. At maturity, such specialized cells may differ greatly from one another in their structures. However, all plant cells have the same basic eukaryotic organization: They contain a nucleus, a cytoplasm, and subcellular organelles, and they are enclosed in a membrane that defines their boundaries. Certain structures, including the nucleus, can be lost during cell maturation, but all plant cells begin with a similar complement of organelles. An additional characteristic feature of plant cells is that they are surrounded by a cellulosic cell wall. Inside the cell wall of the living cell is the living substance known as the protoplasm. Protoplasm is the physical basis of life. It is a thick fluid and a very complex substance found dispersed in the medium of water. The proteins of protoplasm are found in water in a colloidal state. Generally, protoplasm is an emulsoid in which the proteins remain dispersed in the water. Many particles possess the power of holding and absorption of water. This is called hydration and the colloids which absorb water are said to be hydrated. The colloids of protoplasm remain hydrated and the amount of hydration depends upon various conditions. Thus the cell is the principal site of metabolic events. ### The Structure of Protoplasm - **Nutrition**: Among the cell organelles, the Chloroplast is the most important structure unique to plant cells and involved in the most important physiological process called Photosynthesis. This process is the source of food for life on earth. - **Respiration**: The organelle of the plant cell involved in cellular respiration is the mitochondrion. This organelle is concerned with the oxidation of food substances to release CO2, water, and energy in the form of Adenosine Triphosphate (ATP). - **Protein Synthesis**: The synthesis of proteins and their transport is carried out by the ribosomes and the endoplasmic reticulum. - **Secretion**: Cell secretion takes place by the organelle called the Golgi body which is referred to as the dictyosome in plants. The plant is thus able to co-ordinate the physiological activities of the various organelles and behaves as a perfect physiological unit. Thus the cell is the site of important physiological functions. ### Properties of Protoplasm: The protoplasm is the living component of the plant cell and involves four parts (i) the cytoplasm, (ii) the vacuoles, (iii) a number of organelles and (iv) the nucleus. In young cells, the vacuoles are many, small, and scattered, whereas in a mature cell, there is a single large vacuole occupying the center of the cell and the cytoplasm forms a thin peripheral layer around the vacuole. #### The Physical Nature of Protoplasm: Many theories have been put forth to explain the physical nature of the protoplasm. - **Alveolar Foam Theory Butschili** in 1882, said that the protoplasm is a semi-transparent, viscous and slimy substance, essentially a liquid possessing a foaming or alveolar structure. - **Colloidal Theory**: Wilson Fischer (1894) considered the protoplasm as a polyphase colloidal system. This theory is widely accepted one as the protoplasm is seen to exhibit the properties of colloids. #### Properties: - **Colloidal System**: The protoplasm forms a colloidal system composed of a water phase in which mineral matter is dissolved, also having a protein phase, a fat phase, and many minor phases. So it is said to be a polyphase colloidal system. - **Solation and Gelation**: The protoplasm exists mostly as a sol (which is semi-liquid) but sometimes it becomes rigid and is viewed as a gel (which is semi-solid). Some of the hydrophilic sols under certain conditions are able to form an extremely viscous solid-like mass. For instance, hot aqueous gelatin will set to form a jelly-like mass called a gel. When a sol is converted into a gel, it is called gelation. On the other hand, when a gel of gelatin is heated, it will form a sol, and the process is called solution. Typically, a hydrophilic sol-gel system is reversible; its state depends upon temperature. However, a blood clot is an example of irreversible gel. It is believed that gel structure comprises both solid and liquid phases, and these are continuous. The solid phase constitutes a meshwork of long interwoven threads of sub-microscopic dimensions, and the spaces within them are occupied by the liquid phase. This structure is supported on several grounds, e.g., diffusion of solutes, electrical conductivity and the velocity of chemical reactions. Hydrophobic colloids are inorganic compounds and their preparation involves chemical reactions. When a concentrated solution of FeC13 is added to hot water, a dark-red colloidal suspension of Fe (OH)3 is formed. FeCl3 ionizes, and the hydrolysis of Fe3+ ions occurs to form Fe (OH)3. Aggregation of the Fe(OH)3 molecules forms the colloidal particles of the dispersed phase - **Brownian Movement**: The particles of the protoplasm show an erratic zig-zag movement. This random motion, caused by the uneven bombardment of particles is called Brownian movement. - **Tyndall Effect**: The scattering of a beam of light by the particles of a colloid is termed tyndall effect. This is a property of the protoplasm also. - **Ultrafiltration**: The particles of the protoplasm cannot be filtered through ordinary filter paper, but can be filtered through ultrafilters such as millipore filters. - **Electrical Properties**: The particles of the colloid carry a uniform electric charge. - **Flocculation or Coagulation**: When the particles of a colloid lose their charges, they tend to aggregate and increase in size. As a result, they fall out and get precipitated. In other words, protoplasm loses its living property. These properties of the protoplasm indicate that it is a living substance and has rightly been termed as the physical basis of life (vivum fluidum). ### Chemical Nature of Protoplasm: The major constituent of the protoplasm is water, which makes up 90% of it. The dry matter has several organic and inorganic substances. Proteins and other nitrogen-containing compounds constitute the bulk of organic matter. Liquids like fats and oils are also present in small amounts. Compounds consisting of chlorides, phosphates, sulfates, and carbonates of magnesium, potassium, sodium, calcium, and iron are also present. Since the protoplasm contains all the chemical constituents required for life, it has been called the "Physical and Chemical Basis of Life". ## Diffusion With this background, the process of diffusion becomes relatively simple. Diffusion is usually defined as the net movement of a given substance from an area of its own high concentration into another area of lesser concentration due to its free transitional kinetic energy. This movement is continuous and in random, such as the ions of potassium permanganate diffusing from a crystal placed in a beaker of water. In terms of concentration, it is not difficult to understand this process in an intuitive way. Imagine two rooms connected through an opening, and the first room is having perfectly elastic white balls in free motion, while the second room contains perfectly elastic black balls in motion. At the beginning, the concentration of black balls in one room is much higher than it is in the other. Gradually, a condition of equilibrium will be approached in which the number of black balls is the same in both rooms. At this time, the chances of a black ball going in one direction through the opening are the same as the chances of a black ball going in the opposite direction. Of course, the same would be true for the white balls, and indeed the direction of diffusion of each will be independent of the other. In terms of the entropy concept (entropy is the extent of randomness), there was a relatively high degree of order existing when the balls were separated in the two different rooms (or when any concentration gradient exists, such as the gradient from the potassium permanganate crystal to the surrounding pure water). Because of this high degree of order, the level of entropy is low. At equilibrium, there is much less order, a maximum state of randomness has developed (the ions of potassium permanganate have become equally distributed throughout the entire beaker). Difference in quantity of free energy in different parts of the system is due to differences in concentration. Diffusion occurs from the point of high energy towards the point of low free energy, a state is reached in which free energy content throughout the system becomes equal is called equilibrium. Diffusion of a substance will tend to occur downward along any free energy or chemical potential gradient, regardless of how the gradient is established. ## Factors Affecting Rate of Diffusion - **Temperature**: Suppose molecules on one side of the membrane are warmer than those on the other. Thermodynamic considerations indicate that raising the temperature increases the free energy or chemical potential. Diffusion will thus occur toward the cooler side of the container. Temperature increases the rate of molecular movement; therefore, increases the rate of diffusion. - **Pressure**: Pressure increases in one side of a system will increase the pressure-volume product and raise the water potential. This will cause diffusion of water towards the side exposed to lower pressure. Pressure gradients across cell membranes greatly influence water movement in the plant. - **Presence of Dissolved Solutes**: Suppose that a small amount of sugar is added to the water in one compartment of the system, accompanied by the removal of an amount of water which keeps the pressure constant. But, because of a difference in water potential across the membrane, water will diffuse into this compartment again. The difference in water potential is caused by the interacting of water molecules with solute particles in such a way that the free energy of the water molecules is decreased. The number of solute particles is important. So, the rate of diffusion is directly proportional to the concentration gradient. The greater the difference in concentration between two areas, the greater the rate of diffusion. Thus, when the gradient is zero, there will be no net diffusion. - **Adsorption**: Imagine that the compartment of one side of a membrane is filled with nearly dry particles of clay and the other compartment is filled with pure water. Water will diffuse across the membrane into the clay. There is a great tendency for water molecules to become adsorbed onto the surfaces of the clay particles, and so there is a steep gradient in water potential between the water molecules in pure water and the surfaces of the clay particles. The result of this water potential gradient is diffusion of water toward the clay. This is of obvious importance to a plant in the soil. - **Size and Mass of the Diffusing Particles**: At a given temperature, the smaller the particles, the higher its velocity and hence the greater the diffusion rate of this particle. So, the chlorine ion, for example, diffuses more rapidly than a ferric ion and many times more rapidly than a glucose molecule. When two particles of the same size, but of different masses diffuse, the heavier particle will diffuse more slowly. The rate of diffusion is indirectly related to molecular weight. ## Permeability The entry and exit of water into and out of the plant cells is due to a phenomenon called permeability of the plasma membrane. Permeability is a property of membranes which determines the readiness with which such membranes allow materials to pass through them. The discussion of the movement of materials across membranes is basically a discussion of permeability. The membranes are divided into the following types on the basis of their permeability: - **Permeable membrane**: Such membranes allow movement of both solutes and solvent through them. Cell wall is usually considered to be a permeable membrane, i.e., it allows the diffusion of water as well as most of the solutes, unless it contains large proportions of subrin and cutin, both of which are relatively impermeable to water solutes. - **Impermeable membrane**: These types of membrane don't allow the movement of substances through them, e.g., cutinized cell wall. - **Semi-permeable membrane**: Such membranes allow the passage of solvent molecules, but don't allow the passage of solute e.g., parchment membrane, celloidin membrane. - **Selective permeable membrane or differentially permeable membrane**: Such membranes allow selective solutes to pass through them along with the solvent molecules, e.g., plasma membrane, Tonoplast. This membrane is permeable for CO2, N2, O2 gases, and alcohol, ether, and water, but impermeable for polysaccharides and proteins. ### Membrane Structure and Function Membranes represent the primary permeability barrier of cells and therefore regulate the follow of substances into and the products out of cells. These membranes separate the cytoplasm of the cell from the surrounding medium and divide it into several compartments, each of which plays a specialized role in the economy of the cell. The surface membrane of a cell is the only part of that cell directly exposed to the rest of the world, and as such, it is the part of the cell recognized in cell-cell interaction. Membranes are water-insoluble, relatively tough structures of very high molecular weight. Membranes differ in their lipid content, in the level of certain enzyme "markers," and in their detailed appearance in the electron microscope, and these differences often allow the biochemist to obtain a preparation greatly enriched for a particular type of membrane. Purified membranes consist almost exclusively of lipid and protein; most membranes also contain a certain amount of carbohydrate, which is covalently linked either to lipid or to protein, in the form of glycoprotein or glycolipids. The ratio of lipid to protein is widely variable in membranes from different sources. The type as well as the proportion of lipid varies considerably among membranes from different sources. Water can cross plant membranes by diffusion of individual water molecules through the membrane bilayer, as shown on the left, and by microscopic bulk flow of water molecules through a water-selective pore formed by integral membrane proteins, such as aquaporins. ### Main Factors Affecting the Permeability of Plant Cell: - **Temperature**: Within a certain range of temperature (0 - 45°C), the permeability of plasma membranes increases with the rise of temperature and decreases with lowering the temperature. The changes in permeability within this range of temperature are reversible. The increase in permeability due to rise in temperature is due to the increase in the kinetic energy of the solvent and the protoplasm, allowing faster movement of the diffused particles. At temperature nearly higher than 50°C, the permeability of the plasma membranes is greatly increased, and such an increase is irreversible. Such a temperature is referred to as a lethal temperature. This temperature is proposed to cause irreversible coagulation and accumulation of the colloidal particles of the protoplasm, including the plasma membranes, leading to a marked increase in the permeability, which causes the death of the plant cell. Lower temperatures that cause ice formation in the cell result in irreversible increases of permeability like higher temperatures. It was first proposed that such lower temperatures cause an increase in the size of water present in the plasma membranes when being changed to ice leading to a destruction of these membranes. But, the microscopically examination of these cells shows that this view is wrong since the plasma membranes of such cells are found not to be destroyed. It is believed that lower temperatures leading to ice formation, cause the dehydration of the protoplasm, including the plasma membranes as a result of the change of water into ice, and so the colloidal particles of the protoplasm are irreversibly accumulated and coagulated leading to irreversible increases in the permeability which may lead to the death of the cell. - **Light**: It has been found that the permeability of the plasma membranes increases in the presence of light and decreases in darkness. Light may increase the kinetic energy of the diffused particles, and it may have a heating effect, both of which cause an increase in the permeability of the illuminated cells. It has been shown that short wave lengths of light, such as blue or violet (the energy of light is directly proportional to its energy) are more effective in increasing the permeability of the plasma membranes than long wave lengths of light such as red. - **Alkaline and acids**: At relatively low concentrations, alkalis increase the permeability of the plasma membranes, while acids produce opposite effects. This is reversible. However, exposure of the cells to relatively high concentrations of acids and alkalis tend to increase the permeability of these cells. This effect is usually irreversible, and it results in injury and death of the treated cells. - **Anaesthetics**: It has been shown that anaesthetics like chloroform, ether, and alcohols greatly affect the permeability of the plasma membranes of plant cells. Very low concentrations of these substances decrease the permeability, and this effect is usually reversible. Relatively high concentrations of anaesthetics, on the other hand, increase rapidly the permeability, and if this left for longer time, it will be irreversible leading to injury and death of the plant cells. High concentrations of these anaesthetics are likely to dissolve the lipid components of the plasma membrane leading to the irreversible increase in the permeability of these membranes. - **Composition of the external solutes**: It has been found that a solution of a single salt markedly increases or sometimes decreases the permeability of the plasma membranes towards the other substances. If the cells are left in such solution for relatively long time, they are injured, and they may be killed. The injurious effect of the single salt solution can be eliminated by mixing such solution with another one, usually with different valency. Under such conditions, each substance is considered to antagonize the injurious effects of the second one, and this phenomenon is referred to as antagonism. If a marine plant is provided with a solution of a single salt, such as sodium chloride, the concentration of which is similar or even less than that of the sea water, the plant is injured, and may die. Such an effect can be eliminated by adding to sodium chloride solution an amount of calcium chloride solution which will antagonize the harmful effect of the former. So, this explains how plants live in sea water which contains a relatively high concentration of sodium chloride. In sea water, fresh water, and soil solutions, there is a suitable balance between the various salts present so that the plants can live without any injury. Such solutions are known as balanced solutions. #### Explanation of Antagonism: - It is proposed that movement ions may act in the direction of dispersing or decreasing the binding forces between adjacent molecular components of the plasma membranes, whereas di- or polyvalent cations function in the reverse way, binding adjacent components of the plasma membranes more closely together. Each effect independently is harmful to the plant cell, while both eliminate each other - It is proposed that monovalent ions cause the liquefaction of the protoplasm, while di- and polyvalent ions, cause solidification of the protoplasm, each of which is harmful to the protoplasm, whereas both actions antagonize each other leaving the protoplasm in a normal state. In some cases the entrance of a substance is accelerated by the presence of another one. Such a phenomenon is referred to as synergism. In each case, the harmful action of the mixed solutions is greater than that of each solution alone. ## Osmosis Because in the plant there are many restrictions upon the free diffusion of solutes, the special diffusion of water transfer called osmosis is of particular importance. There are cellular membranes, vacuolar membrane, nuclear membranes, membranes around plastids such as chloroplasts and even membranes around plastids such as chloroplasts and even membranes around mitochondria. In each of these examples, water molecules may pass through the membranes more rapidly than solute particles, and thus the essential features of an osmotic system are present. The consequences include not only the development of pressure (turgor) within the cells, but also the bulk movement of solutions within the plant. Osmosis is the term used to refer specifically to the diffusion of water across a differentially or semi-permeable membranes. Osmosis occurs spontaneously in response to a driving force. The net direction and rate of osmosis depends on both the pressure gradient. Water will move from its region of higher chemical potential (or concentration) to its region of lower chemical potential until equilibrium is reached. At equilibrium, the ### The Osmotic System: A system in which osmosis occurs is called an osmometer. This may be a laboratory device or a living cell may be thought of as an osmometer. In either case, two things are essential: - **First**: Solutions or pure water must be isolated by something that restricts the movement of solute particles more than it restricts the movement of solvent molecules. In most cases, however, the restriction is a membrane. - **Second**: An osmometer includes some means of allowing pressure to build up. Such an increase in pressure is not essential for osmosis to occur, but it is typically a part of a laboratory osmometer and an extremely important part of the plant cell osmometer. In the laboratory osmometer, pressure usually builds up hydrostatically by the lifting of solution in a tube. In the cell, pressure can be built up because of the rigidity of the plant cell wall. It is important to differentiate between the cell wall and the membrane. The membrane allows water molecules to pass more readily than solute particles; the cell wall normally allows both to pass readily. It is the plant cell membrane that makes osmosis possible but the cell wall provides the rigidity to allow a build-up in pressure. A perfect osmometer can be constructed in the laboratory; but a cell is never a perfect osmometer. ### The Pressure of Osmosis The diffusion of solute particles compared to solvent molecules can result in the establishment of a water-potential gradient. If there is pure water on one side of the membrane and a solution on the other side (typically inside the laboratory osmometer or the cell), then the water potential of the solution will be lower than that of the pure water. By definition, water potential at atmospheric pressure is equal to zero, and so water potential of the solution will be some negative number (less than zero). Water molecules will diffuse down this water-potential gradient into the solution. The result will be a build-up of pressure within the osmometer, either a raising of liquid in the tube of the laboratory osmometer or a pressure upon a plant cell wall. Increasing pressure will raise the water potential, and so the water potential within the osmometer will begin to increase toward zero. The osmotic pressure is the pressure which is developed in a solution in which solution and water is separated by a semi-permeable membrane or as the hydrostatic pressure developed in a solution which is just enough to stop the entry of the solution into the solution when the two are separated by a semi-permeable membrane. ### Osmotic Potential (Ys ) Osmotic pressure is a confusing term because it develops due to osmosis. It is potential pressure in the absence of energy. Therefore, the term osmotic potential is used. Osmotic potential is to express the absence of energy in solution, due to the amount of solvent-solute interaction and is represented by negative sing. _OP = 22.4 atm = osmotic potential = -22.4 atm (1M glucose solution)._ ### Turgor Pressure or T.P. and Wall Pressure or WP Physiologists have long differentiated between turgor pressure, the protoplast pushing against the cell wall and wall pressure, equal and opposite pushing of the wall against the cell contents. This usage has been taken from Newton’s third law of equal and opposite reactions. Turgor pressure is not applicable for a free solution. This is only applicable for living cells. Turgor pressure also known as hydrostatic pressure. When a cell is immersed in water, the water enters into the cell because osmotic pressure of the cell sap is higher (hypertonic). The cell content press upon the wall, or develop a pressure against the membrane which is called turgor pressure (T.P). The turgor pressure is counterbalanced by an equal and opposite pressure of the thick cell wall on the enclosed solution, known as wall pressure. It means the amount of pressure exerted by cytoplasm on the cell wall is same, and in opposite cell wall towards the inner side on the cytoplasm. _T.P = W.P_ A flaccid cell has zero turgor pressure. The highest value of turgor pressure is found in a turgid cell, and it is equal to the osmotic pressure. In fully turgid cell: _O.P = T.P_ The value of turgor pressure is negative during the plasmolysis of the cell. Now a days, Turgor pressure is known as pressure potential. ### Diffusion Pressure Deficit (DPD) or S.P DPD is a characteristic of water in solution. But generally, it is called DPD of solution or cell. DPD of solution is the difference between the diffusion pressure of the water which is present in the solution and diffusion pressure of pure water. DPD determines the direction of osmosis and the power of absorption of the cell. The definition DPD as follows in relation to plants: It is the amount of that pressure by which water is sucked into the cell or expels outside the cell. Therefore, it is known by various names like Suction Pressure (S.P) or Absorption Potential or Suction Force etc. The diffusion of water or solute takes place from the region of lower S.P to the region of higher S.P in the process of osmosis. Normally, osmotic pressure is greater than the turgor pressure in a cell. The difference between osmotic pressure, and turgor pressure is called suction pressure (S.P). Suction pressure is the power of water absorption. The DPD of any free solution is equal to the osmotic pressure of that solution. _DPD = O.P_ #### Types of solution according to osmosis - **Isotonic solution**: If solution in which a cell is placed has equal osmotic pressure to that of cell sap, the outer solution is