BMS2011-L02_Dr Faye McLeod.pptx
Document Details
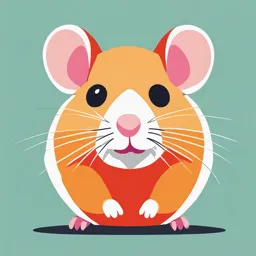
Uploaded by EnthusiasticChrysoprase4588
Tags
Full Transcript
Basic Cellular Neurophysiology Lecture 2 Dr Faye McLeod [email protected] Overview Part 1 - Excitable cells - The resting membrane potential Part 2 - The action potential Part 3 - Neurotransmission across central synapses Part 1 Exci...
Basic Cellular Neurophysiology Lecture 2 Dr Faye McLeod [email protected] Overview Part 1 - Excitable cells - The resting membrane potential Part 2 - The action potential Part 3 - Neurotransmission across central synapses Part 1 Excitable cells and the resting membrane potential Objectives of part 1 You should be able to understand: Why and how cells communicate Types of excitable cells The ionic basis of the resting membrane potential Why do cells need to communicate? To synchronise events To integrate function Adapt to their environment. Turn pathways on and off. Faulty cellular communication is at the heart of many neurological disorders Motor neuron disease Myasthenia Gravis Normal Abnormal Signal Signal Multiple sclerosis Depression Types of communication Cells send and receive chemical messages constantly to coordinate the actions of distant organs, tissues, and cells. Between cells Within Cells Slow Hormones Autocrine Fast Neurotransmission Electrical Hormonal signalling E.g. chemical hormones in local or systemic circulation Autocrine - General - Slow - Sustained - ‘ball park’ control Electrical signalling – excitable cells! A form of intracellular communication via rapid changes in membrane potential. - Local - Fast - Transient - Accurate Occurs in: – neurons in the CNS in the PNS – muscles smooth striated Resting membrane potential What is a membrane potential? All cells (living!) have a potential difference across their cell membrane - with the inside negative to the outside -ve +ve An inactive (non-signalling) neuron has a voltage across its membrane called the resting membrane potential How does the resting membrane potential arise? Cells have a lipid bilayer membrane Lipid bilayer membrane The membrane itself is impermeable to small charged atoms/molecules But there are pores (ion channels) Ions and pumps embedded in the membrane… …differentially permeable to different Ion channel ions Ions move across the membrane through passive and active processes There are a handful of crucial ions which contribute to the resting potential, with sodium (Na+) and potassium (K+) providing a dominant influence. Various negatively charged intracellular proteins and organic phosphates that cannot cross the cell membrane are also contributory. Na+ K+ A- Ion permeability in cells (unequal): mM mM K + 100 K+ 5 Na+ 150 Na + 15 Cl- 150 Cl - 13 A- 0 A- 385 There are far fewer channels for Na+ making it relatively impermeable to Na+ There are lots of large anions (A-) fixed inside the cell Na+ is concentrated on the outside and K+ on the inside The cell membrane is (almost) impermeable to Na+ The Na+/K+ ATPase pumps Na+ out of the cell (and K+ in) Requires a lot of energy! Na+ Na+ K+ + Na+ Na+ Na+ K + K K+ Na+ Na+ Na + K + K+ Na+ K+ K+ Na+/K+ ATPase pump Na+/K+ ATPase pump Na+ is concentrated on the outside and K+ on the inside The cell membrane is (almost) impermeable to Na+ The Na+/K+ ATPase pumps Na+ out of the cell (and K+ in) K+ leak channel K+ K+ flows out of cell via K+ K+ ‘leak channels’. A- A- K+ A- A- Large anions (A-) A- Na+ Na+ are stuck inside K + + Na+ Na+ Na+ K + K K+ Cl- Na+ Cl- stays outside to Na+ Na + K + K+ Na+ Cl- balance the anions Cl- K+ K+ Na /K ATPase pump + + Cl- Cl- Electrochemical gradient This consists of two parts: - the chemical gradient (concentration difference inside and out) - the electrical gradient (ionic charge difference across the membrane) Chemical gradients REMEMBER: there is more Na+ on the outside and more K+ on the inside K+ can move outside down its chemical gradient via leak channels but Na+ cannot move inside down its chemical gradient due to membrane impermeability Electrical gradients The Na+/K+ ATPase switches 3 Na+ for 2 K+ -therefore increasing the -ve charge on the inside The direction of the electrical driving force results in the ion moving toward a region where the opposite charge exist Cations want to go into the cell to equalize the charge - Na+ can’t go into the cell - K+ wants to stay inside Eventually get to an equilibrium state What is an equilibrium state? K+ as an example: K+ wants to: - move outside down its chemical gradient - stay inside because of the electrical gradient + + + _ + _ + K + _ _ K+ Chemical K+ + K+ K+ K + K+ _ _ K+ + _ Electrical + _ K+ K+_ + _ + + + + The membrane is permeable to K+ (‘leak channels’) K+ will move until these two forces are equal and opposite This is called the equilibrium potential for K+: electrical gradient chemical gradient i.e. no movement in either direction and ions are unequally distributed All determined by the Nernst equation Gives the equilibrium potential of an ion present on both sides of a membrane (assuming permeability to that ion) Ei = 2.3RT log [C]out zF [C]in Ei = equilibrium potential (mV) Z = ion charge R = gas constant F = Faraday constant T = absolute temperature [C]out = concentration outside [C]in = concentration Every ion has its equilibrium potential EK = -80 mV ENa= +60 mV ECl = -65 mV Now back to the resting membrane potential… The resting membrane potential (RMP) is the potential across the membrane when the cell is at rest If the membrane were permeable only to K+ the RMP would be -80 mV BUT, the membrane is differentially permeable to many ions! The Nernst equation doesn’t account for this so we need the Goldmann Equation True resting membrane potential The Goldmann Equation: The RMP is the sum of the equilibrium potentials for all the ions K+ contributes the most because of permeability Em= -70 mV (RMP) Key points from part 1 The unequal distribution of ions leads to a negative charge inside the cell The RMP is maintained in cells by: – fixed anions (A-) inside the cell – the active transport of Na+ out of the cell (Na+/K+ ATPase) – the high permeability of the membrane to K+ (leak current) The RMP is approximately -70 mV Part Part 22 The action potential Objectives of part 2 You should be able to understand: The changes in membrane currents responsible for the action potential How these changes are brought about The structure of the neuron How the action potential is conducted along the axon The action potential A rapid, transient change in membrane potential All-or-nothing phenomenon Most excitable cells (e.g. neurons and muscle cells) can generate action potentials They are the output response to integrated inputs The action potential +30 Membrane potential (mV) Depolarisation Repolarisation -15 After hyperpolarisation -70 RMP 0 1 2 3 time (ms) Role for voltage-gated ion channels Ions cross the membrane through: - the voltage-gated Na+ channel - the voltage-gated K+ channel Open at particular voltages (membrane potentials) When open, allow the passage of ions into or out of the cell Are selective for particular ions on the basis of size (e.g. Na+ vs K+) and charge (cation vs anion) Active Inactive REMEMBER! Not to be confused with the non-gated K+ leak channel or Na+/K+ ATPase pump! K+ K+ K+ The voltage-gated Na+ channel (VGSC): Responsible for the depolarization phase of the action potential 1. When the membrane potential +30 Sodium permeability reaches -55 mV Potential (mv) 2. The VGSCs open -15 very rapidly 3. Na+ rushes into the cell down its concn. -70 gradient 4. Causing further 0 1 2 3 depolarization time (ms) 5. The Na+ channel stays open for less than 1 ms 6. The channel undergoes time-dependent inactivation 7. Na+ is pumped out of the cell causing repolarization 8. The Na+ channel returns to its closed state +30 Sodium permeability Potential (mv) -15 -70 0 1 2 3 time (ms) Voltage gated sodium channels can exist in three states: inactivated time dependent time dependent fast slow open closed voltage-gated, fast The voltage-gated K+ channel (VGKCs): Responsible for the repolarization phase of the action potential 1. When the Potassium permeability membrane +30 potential reaches - Potential (mV) 55 mV -15 2. VGKCs open (slowly) 3. K+ moves out of the -70 cell down its concn. gradient 0 1 2 3 4. Causing time (ms) repolarization 5. When the membrane potential repolarizes to -15 mV 6. The voltage-gated K+ channels close (slowly) Potassium permeability +30 Potential (mV) -15 -70 0 1 2 3 time (ms) Current flow The ions are charged so carry current Na+ moves inward increasing +30 membrane potential towards Potential (mV) Na+ in the +ve -15 K+ moves outward decreasing membrane -70 potential 0 1 K+ out time 2(ms) 3 The two currents summate to make the action potential The after hyperpolarization The voltage-gated K+ channels opens slowly K+ moves down its concentration gradient out of the cell The voltage-gated K+ channels close slowly allowing too much +ve charge to leave the cell This results in the ‘after hyperpolarization’ (AHP) The absolute refractory period: For a short time after an action potential has been generated, the membrane is incapable of generating another action potential This is the ‘absolute refractory period’ An action potential is initiated by the opening of Na+ channels After opening, Na+ channels become inactivated Inactivated channels must return to the closed state before they can open again REMEMBER Summary of action potential key parts Influx of Na+ produce the depolarizing peak of the action potential Efflux of K+ produces the repolarizing phase The slow closing of K+ channels causes an after hyperpolarization after the action potential +30 Potential (mV) -15 -70 RMP 0 1 2 3 time (ms) How does the action potential start? To answer that we need to introduce neurotransmission: Transmission of nerve impulses between neurons Axon from excitatory neuron Neurotransmitter Dendrites SYNAPSE Pre Axon hillock Axon cell body Post Axon terminal Axon from Postsynaptic excitatory or inhibitory neuron inhibitory receptors Action potential generation Stimulation of neurotransmitter receptors (e.g. ligand gated ion channels) around the cell body Causes small changes in membrane potential called excitatory postsynaptic potentials (EPSPs) or inhibitory postsynaptic potentials (IPSPs) +30 EPSPs and IPSPs occurring close in EPSP Potential (mV) -15 time will summate IPSP Threshold At threshold (-55mV), -70 RMP VGSCs open 0 1 2 3 time (ms) Action potential initiation An action potential will start when the membrane reaches the threshold for opening of VGSCs Action potentials start at the axon hillock, where there are many VGSCs. Axon hillock +30 Potential (mV) -15 Threshold -70 RMP 0 1 2 3 time (ms) How does the action potential move along the neuron? ++++ - - - - ++++ ++++ - - - - ++++ - - - - - - - - Immediately adjacent to a region of depolarized membrane the charge is opposite Ions move to try to equalise this potential difference along the axon The adjacent section reaches threshold (-55mV) and Na+ channels open Action potential propagation ++++ - - - - ++++ ++++ - - - - ++++ - - - - - - - - ++++++++ - - - - ++++ - - - - - - - - ++++ - - - - Region of depolarized membrane moves along the axon The region behind the AP is refractory forcing the direction of travel Action potential conduction The speed of movement of action potentials is dependent on: – Axon diameter – Membrane resistance (myelination) The highest conduction velocity will be for a neuron with: - Large axon diameter (more room for current to flow) - High membrane resistance (less leakage of potential) Velocity (V) of action potential = 1-100 metres/s Importance of myelination Some neurons have myelinated axons Myelin provides electrical insulation Conduction is faster in myelinated axons Central Nervous System (CNS) Peripheral Nervous System Oligodendrocytes form the Schwann cells form the myelin myelin sheath sheath Faulty myelination can result in diseases such as Multiple Sclerosis... Multiple sclerosis (MS) is a condition that affects your brain and spinal cord. In MS, the myelin is damaged. Cause is unclear. Normal Abnormal Results in symptoms such as blurred vision and problems with how we move think and feel. Signal Signal In the CNS: Nodes of Ranvier have lots of Na+ channels allowing current jumps: ‘saltatory conduction’ Nodes of Ranvier Current spreads further inside the axon because of better insulation Membrane potential reaches threshold at nodes of Ranvier Action potential jumps from node to node Conduction is faster because of jumping. Note: unmyelinated axons have a slower ‘smooth conduction’ Key points from part 2 Excitable cells can generate an action potential The action potential – voltage gated channels open – Na+ to enters cell (depolarizing) – K+ leaves cell (repolarizing) Action potentials are initiated at the axon hillock and propagate along the axon via current loops The refractory period prevents the action potential from travelling backwards Part Part 2 3 Neurotransmission Objectives of part 3 You should be able to understand: Structure and types of synapses in the CNS Neurotransmitters types Elements of neurotransmission at central synapses How drugs can interact with neurotransmission How do neurons communicate with other cells? Via neurotransmission – chemical signalling or electrical signalling Junctions – Neuron - neuron – Neuron – muscle – Neuron - secretory cell Junctions between neurons and other cells are called synapses Axon from excitatory neuron Neurotransmitter Dendrites SYNAPSE Presynaptic Axon hillock Axon cell body Postsynaptic Axon terminal Axon from inhibitory neuron Synaptic cleft Synapses in the central nervous system (CNS) Synapses in the CNS Neurons in the CNS receive inputs from hundreds of other neurons at many locations (cell body, dendrites, axons…) Excitatory input: – Depolarises postsynaptic membrane – E.g. by opening Na+ channel Inhibitory input: – Hyperpolarises postsynaptic membrane, or – Holds membrane at resting potential – E.g. by opening Cl- channels Neurotransmitters Many different types of molecule function as neurotransmitters: Amino acids – glutamate, GABA Amines –dopamine, noradrenaline, 5-HT Peptides –CRH, opioids, substance P ATP Nitric Oxide Neurotransmitter Neurons are generally classified according to the main transmitter they contain Elements of Neurotransmission 1. Synthesis 2. Storage 5. Termination 3. Release 4. Receptor interactions 1. Synthesis Neurotransmitters are synthesised in the cell body (peptides) or close to the site of release (small molecules) Microtubules Precursors are substrates for enzymes Biosynthetic enzymes Newly synthesised transmitter may be inactivated by cytosolic enzymes Precursors Newly synthesised neurotransmitter Transporter 2. Storage Neurotransmitters are stored in synaptic vesicles Protecting them from enzymatic degradation Providing a ready package for release Synaptic vesicles 3. Release Exocytosis of synaptic vesicles 1. Neurotransmitter is released in response to an action potential 2. Depolarization opens voltage- Voltage gated calcium channel gated Ca++ channels 3. Ca++ influx promotes fusing of the vesicles 4. Vesicles fuse with membrane and empty contents Ca++ 4. Receptor interactions Neurotransmitters bind to specialised proteins called ‘receptors’ The receptors are linked to ion channels or 2nd messengers Ca++ The binding of the transmitter to the receptor causes membrane and/or intracellular responses There are many different types of receptors for each neurotransmitter (see later) Receptors 5. Termination Most neurotransmitters are removed from the synaptic cleft by an active uptake process Specialised protein transporters Transporters remove the neurotransmitter from the synaptic cleft This process terminates the action of the neurotransmitter Once in the nerve terminal, enzymes breakdown most transmitters Classes of neurotransmitter receptors Two major classes: Ionotropic - ions pass through the receptor - Ligand-gated ion channels - Can be excitatory or inhibitory - Fast signalling Metabotropic – a 2nd messenger is required to signal - G-protein coupled receptors - 7-transmembrane structure - Activate downstream events - Slower signalling 1. Ionotropic receptors E.g. Excitatory ligand-gated ion channel Binding of the transmitter Ligand +ve (ligand) causes receptor to open Na+ enters the cell down its -ve Na+ concentration gradient Depolarizing the membrane -70mV 2. Metabotropic receptors Neurotransmitter Binding of transmitter binding site Adenylyl cyclase causes G-protein to (enzyme) 2nd messenger hydrolyse (GDP>GTP) Enzymes are activated/inhibited +ve Intracellular 2nd -ve messengers are GDP cAMP increased/reduced Intracellular GTP GTP ATP Kinases are loops activated/deactivated Intracellular Ca++ is G-protein Protein kinase mobilised/sequestered activation Membrane is more/less excitable Receptor Subtypes Each neurotransmitter binds to several distinct receptor subtypes, e.g. Noradrenaline (NA) – α and β (both G-protein linked) GABA – GABAA (ligand-gated ion channel) – GABAB (G-protein linked) Drugs at central synapses Many drugs interact with neurotransmission They can modify precursors, enzymes, release machinery, transporters…and many more! Therapeutic agents include: – antidepressants, antihypertensives, bronchiodilators Drugs of abuse include: – heroin, ecstasy, cocaine Antidepressants Some drugs work by blocking the metabolism or uptake of 5-HT (serotonin) transmission TRYP Tryptophan (TRYP) 5-HTP 5-HT Transporters Monoamine oxidase (MAO) – breaks down 5-HT. 5-HT Monoamine oxidase inhibitors increase vesicular Selective Serotonin content reuptake inhibitor MAO (SSRI) blocks uptake of 5HT 5-HT receptors Psychostimulants Drugs which cause the non-exocytotic release of dopamine (DA) are psychostimulant and have abuse potential Normal Exocytosis Amphetamine and ecstasy cause non- impulse dependent release of DA DA DA Key points from part 3 CNS synapses Five elements of neurotransmission – synthesis, storage, release, receptor interaction and termination. Two major classes of receptors – ionotropic (fast) and metabotropic (slow) Different subtypes of receptors All highly specific!