Neurophysiology Chapter 1 Study Guide PDF
Document Details
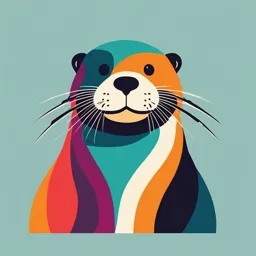
Uploaded by HeartwarmingJasper9156
Tags
Summary
This document is a study guide on chapter 1 of neurophysiology. It covers cellular components of the nervous system, neuron structure and mechanisms, and different types of neurons and synapses. It's structured as a guide, not as an exam paper.
Full Transcript
Neurophysiology CHAPTER 1 – STUDY GUIDE GOOD LUCK! 1 Index Cellular Components of the Nervous System:...........................
Neurophysiology CHAPTER 1 – STUDY GUIDE GOOD LUCK! 1 Index Cellular Components of the Nervous System:................................................................................. 2 Structure and Mechanisms of a Neuron........................................................................................... 3 Functional Organization of a Neuron................................................................................................ 4 Types of Neurons................................................................................................................................. 5 Types of Synapses............................................................................................................................... 6 Types of Glia......................................................................................................................................... 7 Basic Neurophysiology........................................................................................................................ 8 Components of Neurophysiology:................................................................................................. 8 Voltage-Activated IonChannels......................................................................................................... 10 Voltage-Activated IonChannels during an Action Potential............................................................ 10 Refractory Periods............................................................................................................................. 10 Types of Refractory Periods......................................................................................................... 10 Continuous Conduction..................................................................................................................... 11 Saltatory Conduction.......................................................................................................................... 11 Factors Affecting Velocity of the action potential.......................................................................... 11 Vesicles................................................................................................................................................ 12 Definition.......................................................................................................................................... 12 Types of Vesicles in Neurophysiology........................................................................................ 12 Synaptic Signal Transduction........................................................................................................... 12 Neurotransmitters............................................................................................................................... 14 Glutamate............................................................................................................................................ 15 GABA and glycine.............................................................................................................................. 15 Acetylcholine....................................................................................................................................... 16 Biologic amines................................................................................................................................... 16 ATP....................................................................................................................................................... 17 Neuropeptides..................................................................................................................................... 17 2 Cellular Components of the Nervous System: 1) Neurons: Neurons are the fundamental units of the nervous system, specialized cells responsible for transmitting electrical and chemical signals. They consist of three main parts: Dendrites – receive signals from other neurons. Cell body (soma) – processes the information. Axon – transmits signals to other neurons, muscles, or glands. Neurons communicate through electrical impulses and chemical neurotransmitters at junctions called synapses. Their ability to generate and transmit signals makes them essential for all nervous system functions, including sensation, movement, cognition, and reflexes. 2) Circuits: Neural circuits are functional groups of neurons that work together to process information and generate responses. These circuits form the basis of all neural activity, from simple reflexes to complex thought processes. For example, the reflex arc is a basic neural circuit involving sensory neurons (detect stimuli), interneurons (process information), and motor neurons (execute responses). Circuits can be excitatory or inhibitory, shaping the overall neural output. 3) Neural networks: Neural networks are large-scale interconnected systems of neurons and circuits that process and integrate information across different brain regions. While circuits handle specific tasks, networks allow for distributed processing, meaning different parts of the brain can work together to perform complex functions like memory, decision-making, and consciousness. Neural networks can be localized (e.g., visual processing in the occipital lobe) or distributed (e.g., attention and cognition involving multiple brain areas). 4) Glial Cells: Glial cells are non-neuronal cells that provide structural and functional support to neurons. They play crucial roles in maintaining the neural environment, protecting neurons, and aiding in signal transmission. There are several types of glial cells, including: Astrocytes – regulate the chemical environment and blood flow. Oligodendrocytes (CNS) & Schwann Cells (PNS) – produce myelin, which insulates axons and speeds up signal transmission. Microglia – actasthebrain’simmunecells,removingwasteandpathogens. Ependymal Cells – produce cerebrospinal fluid (CSF) and help circulate it. Glial cells were once thought to be passive support cells, but they are now recognized as essential players in brain function and health. 5) Glia: "Glia" is another term for glial cells, collectively referring to all non-neuronal support cells in the nervous system. The word "glia" means "glue" in Greek, reflecting the early belief that these cells merely held neurons together. However, research now shows that glia actively influence neural signaling, brain plasticity, and even learning and memory. 3 Structure and Mechanisms of a Neuron Active Potential: An action potential is a rapid, temporary electrical signal that travels down a neuron's axon. It allows neurons to transmit information over long distances. Steps of an Action Potential: 1. Resting State – The neuron is at rest, with a charge of around -70 mV inside (more negative ions inside than outside). 2. Depolarization – When a stimulus reaches a threshold, voltage-gated sodium (Na ) channels open, allowing Na to enter. This makes the inside more positive. 3. Repolarization – Voltage-gated potassium (K ) channels open, allowing K to leave the cell, restoring a negative charge inside. 4. Hyperpolarization & Refractory Period – The neuron temporarily becomes more negative than its resting state before stabilizing. This prevents immediate re-firing. 5. Return to Resting Potential – The sodium-potassium pump (Na /K ATPase) restores the original ion balance, preparing the neuron for the next signal. The all-or-nothing principle states that an action potential only occurs if the stimulus reaches the required threshold; otherwise, no signal is sent. Synapses: A synapse is the connection between two neurons, where communication occurs. There are two main types: 1. Electrical Synapse – Direct connection through gap junctions, allowing ions to pass quickly between neurons (found in reflex pathways). 2. Chemical Synapse – Most common; uses neurotransmitters to transmit signals across a gap called the synaptic cleft. A single neuron can form thousands of synapses, allowing complex neural communication. Synaptic Cleft: The synaptic cleft is the small gap (~20-40 nm) between the axon terminal of one neuron and the dendrite or cell body of the next. This space prevents direct electrical transmission and ensures that signals are passed chemically. How Transmission Occurs (Synaptic Transmission): 1. Action potential reaches the axon terminal, triggering calcium (Ca² ) channels to open. 2. Calcium influx causes vesicles to release neurotransmitters into the synaptic cleft. 3. Neurotransmitters bind to receptors on the next neuron, causing an excitatory or inhibitory response. 4. Neurotransmitters are cleared by reuptake, enzyme breakdown, or diffusion. This process ensures controlled and directional communication between neurons. 4 Functional Organization of a Neuron A. Soma: The soma, also called the cell body, is the central part of a neuron. It contains the nucleus, which holds the neuron’s DNA, and the cytoplasm, where essential cell functions occur. The soma integrates signals from the dendrites and determines whether to generate an action potential. It also produces proteins and neurotransmitters needed for neuron function. B. Perikaryon: The perikaryon is another term for the cytoplasm of the soma, excluding the nucleus. It contains organelles like mitochondria (for energy), Golgi apparatus (for packaging proteins), and ribosomes (for protein synthesis). The perikaryon plays a crucial role in maintaining neuron health and function. C. Nissel substance: The Nissl substance consists of rough endoplasmic reticulum (RER) and ribosomes, giving neurons a distinctive granular appearance under a microscope. It is responsible for making proteins, including enzymes, neurotransmitters, and structural components. It is absent in axons but abundant in the soma and dendrites, supporting neuron metabolism and repair. D. Microtubules: Microtubules are long, hollow protein filaments that act as "highways" inside the neuron. They help transport materials (such as proteins and neurotransmitters) and maintain the neuron's shape. They are especially important for moving molecules along the axon through anterograde and retrograde transport. E. Anterograde transport: Anterograde transport moves molecules from the soma to the axon terminals. It is essential for delivering neurotransmitters, enzymes, and organelles needed at the synapse. This process is powered by a motor protein called kinesin, which "walks" along microtubules. There are two types: Fast anterograde transport (moves vesicles and neurotransmitters at ~400 mm/day). Slow anterograde transport (moves cytoskeletal proteins at ~1-10 mm/day). F. Retrograde transport: Retrograde transport moves molecules from the axon terminals back to the soma. It allows neurons to recycle materials and send signals about cell health. It is powered by a motor protein called dynein. This process is also how some viruses (like rabies and herpes) enter the nervous system, traveling from peripheral nerves to the brain. G. Dendrites: Dendrites are tree-like extensions of the neuron that receive signals from other neurons. They contain Nissl substance and many receptors for neurotransmitters. Their branching structure increases theneuron’sabilitytocollectinformation,influen learning and memory. H. Spines: Dendritic spines are small protrusions on dendrites where synapses form. They increase surface area, allowing more connections with other neurons. Spines are dynamic— they grow and change based on learning and experience. Dysfunction in spines is linked to neurological disorders like autism and schizophrenia. I. Postsynaptic densities: Postsynaptic densities (PSDs) are protein-rich structures found on the postsynaptic side of a synapse. They contain neurotransmitter receptors, signaling proteins, and scaffolding molecules that help organize synaptic connections. PSDs play a crucial role in synaptic strength and plasticity, which are essential for learning and memory. J. Axon: An axon is a long, cable-like extension of a neuron that transmits electrical signals (action potentials) away from the soma to other neurons, muscles, or glands. Axons can 5 be short or extremely long (e.g., the sciatic nerve axon runs from the spinal cord to the foot). Axons may be myelinated or unmyelinated, which affects signal speed. K. Axon hillock: The axon hillock is the cone-shaped junction between the soma and the axon. It is the site where the neuron decides whether to fire an action potential. The summation of excitatory and inhibitory signals at the axon hillock determines whether the threshold is reached to trigger an impulse. L. Initial segment: The initial segment is the first part of the axon, located just beyond the axon hillock. It is the true site of action potential generation because it has a high concentration of voltage-gated sodium (Na ) channels, making it highly responsive to electrical changes. M. Myelin: Myelin is a fatty insulating sheath that wraps around axons to increase the speed of electrical signals. It is produced by: a. Oligodendrocytes in the central nervous system (CNS). b. Schwann cells in the peripheral nervous system (PNS). Myelin allows signals to "jump" between gaps called Nodes of Ranvier in a process called saltatory conduction, making neural communication much faster and more efficient. Myelin loss (as seen in multiple sclerosis) leads to impaired neural function. Types of Neurons A. Multipolar Neurons: A multipolar neuron is the most common type of neuron in the nervous system. It has: One axon (to send signals). Multiple dendrites (to receive signals). These neurons are found mostly in the brain and spinal cord (CNS) and play a role in complex processing and movement control. Their multiple dendrites allow them to integrate signals from many sources, making them ideal for higher functions like thinking and reflex coordination. B. Pseudounipolar neurons: A pseudounipolar neuron has: A single process that splits into two branches: o One branch connects to the spinal cord (CNS). o The other extends to the periphery (skin, muscles, joints, etc.). These neurons are sensory neurons and are found in the dorsal root ganglia of the spinal cord. Even though they look like they have a single process, their function is similar to bipolar neurons, transmitting signals efficiently over long distances. C. Bipolar neuron: A bipolar neuron has: One axon (to send signals). One dendrite (to receive signals). These neurons are rare and mostly found in sensory pathways. Their simple structure helps them transmit specific, highly refined sensory information to the brain. 6 Types of Synapses Axodendritic Synapses: An axodendritic synapse is a type of synapse where the axon of one neuron connects to the dendrite of another neuron. These are the most common type of synapses in the brain. They can be: Excitatory (increasing the chance of an action potential). Inhibitory (reducing the chance of an action potential). Most excitatory synapses occur on dendritic spines, while inhibitory synapses are often found on the dendritic shaft. Temperospatial summation: Neurons receive thousands of inputs from different synapses. Whether a neuron fires an action potential depends on summation, which occurs in two ways: 1. Temporal Summation: o Multiple signals from the same synapse occur in rapid succession. o If the signals arrive close together in time, they add up and may trigger an action potential. 2. Spatial Summation: o Multiple signals from different synapses occur at the same time. o If excitatory inputs are strong enough (or if inhibitory inputs are weak), they add up and can cause an action potential. This process allows the neuron to integrate all incoming signals and decide whether to fire. Axosomatic Synapses: An axosomatic synapse is a synapse where the axon of one neuron connects directly to the soma (cell body) of another neuron. These synapses have a strong influence because they are closer to the axon hillock (where action potentials start). They are often inhibitory, making it harder for the neuron to fire. Axoaxonic Synapses: An axoaxonic synapse is a synapse where the axon of one neuron connects to the axon of another neuron. Thesesynapsesdon’tdirectly trigger action potentials but instead regulate neurotransmitter release by modifying activity at the presynaptic terminal. They can be facilitatory (enhancing neurotransmitter release). Or inhibitory (reducing neurotransmitter release). 7 Types of Glia Glial cells, or glia, are the support cells of the nervous system. Unlike neurons, they do not generate action potentials but play essential roles in insulation, protection, nutrient supply, and communication. 1) Oligodendroglia: Found in the central nervous system (CNS) (brain and spinal cord). Their main function is to produce myelin, which insulates axons and increases the speed of electrical signals. A single oligodendrocyte can myelinate multiple axons at once. Damage to oligodendrocytes is seen in multiple sclerosis (MS), where the loss of myelin leads to nerve signal disruptions. 2) Schwann Cells Found in the peripheral nervous system (PNS) (nerves outside the brain and spinal cord). They also produce myelin, but unlike oligodendrocytes, each Schwann cell myelinates only one axon segment. Schwann cells also help in nerve regeneration after injury, unlike oligodendrocytes, which have limited regenerative ability. Diseases like Guillain-Barré syndrome involve Schwann cell damage, leading to muscle weakness. 3) Astroglia Star-shaped cells found in the CNS. They have many functions: o Provide structural support for neurons. o Regulate the blood-brain barrier (BBB), controlling what enters the brain from the bloodstream. o Maintain ion balance, especially potassium (K ), which affects neuron excitability. o Help in neurotransmitter recycling, particularly glutamate. o Respond to injuries by forming glial scars. They are the most abundant glial cells in the CNS. 4) Polydendrocytes Also called NG2 cells, these are a unique type of glial cell found in the CNS. They serve as precursors for oligodendrocytes, meaning they can develop into myelin-producing cells. Unlike most glia, they can also receive synaptic input from neurons, though their role in communication is still being studied. 8 5) Microglia The immune cells of the CNS. Their main function is to detect and remove harmful substances, including dead neurons, pathogens, and damaged cells. They become activated during infections or brain injuries, releasing inflammatory molecules to fight damage. Overactive microglia are linked to neurodegenerative diseases, such as Alzheimer’sandParkinson’s , where they may contribute to chronic inflammation and neuronal death. 6) Ependymal Cells Found in the central nervous system (CNS), lining the ventricles of the brain and the central canal of the spinal cord. Their main function is to produce, circulate, and regulate cerebrospinal fluid (CSF), which cushions the brain and spinal cord. They form a barrier between the CSF and brain tissue, but unlike the blood-brain barrier, they allow some exchange of molecules. Key Features: Cilia – Tiny hair-like structures that help move CSF. Microvilli – Small projections that assist in regulating CSF composition. Tight Junctions – Control selective passage of substances between CSF and brain tissue. Choroid Plexus: Specialized ependymal cells here actively secrete CSF Clinical Relevance: Dysfunction in ependymal cells can lead to hydrocephalus, a condition where excess CSF accumulates in the brain. They may also play a role in neuroregeneration, as they have some stem-cell- like properties in certain conditions. Basic Neurophysiology Neurophysiology: the study of ion movements across a membrane. These movements can initiate signal transduction and the generation of action potentials. The study of neurophysiology also includes the action of neurotransmitters. Components of Neurophysiology: Ion Movements: Ion movement refers to the flow of charged particles (ions) across the neuron’s membrane , which is essential for generating electrical signals in the nervous system. o Key Ions Involved: Sodium (Na ) – Moves into the neuron during depolarization. Potassium (K ) – Moves out of the neuron during repolarization. Chloride (Cl ) – Usually moves into the neuron, making it more negative. 9 Calcium (Ca² ) – Plays a major role in neurotransmitter release at synapses. o Ion Channels & Pumps: Voltage-gated channels – Open or close in response to changes in membrane voltage (e.g., Na and K channels during action potentials). Ligand-gated channels – Open in response to neurotransmitter binding (e.g., at synapses). Sodium-Potassium Pump (Na /K ATPase) – Restores resting potential by pumping 3 Na out and 2 K in, maintaining the neuron’s negative charge inside. Action Potential (Already explained) Synaptic Transmission (Already Explained) Neurotransmitters: Neurotransmitters are chemical messengers that allow neurons to communicate at synapses. Types of Neurotransmitters: o Excitatory (increase activity): Glutamate – Main excitatory neurotransmitter in the brain. Acetylcholine (ACh) – Involved in muscle activation and memory. o Inhibitory (decrease activity): GABA ( -aminobutyric acid) – Main inhibitory neurotransmitter in the brain. Glycine – Inhibitory, mainly in the spinal cord. o Modulatory (affect broader neural activity): Dopamine – Involved in motivation, pleasure, and movement (Parkinson’s disease istolinked dopamine deficiency). Serotonin – Regulates mood, sleep, and appetite (low levels are linked to depression). Norepinephrine – Involved in alertness and stress response. Neurotransmitter Clearance: o Reuptake – Transporters take neurotransmitters back into the presynaptic neuron (e.g., serotonin reuptake is blocked by SSRIs, used for depression). o Enzymatic Degradation – Enzymes break down neurotransmitters (e.g., acetylcholinesterase breaks down ACh). o Diffusion – Neurotransmitters drift away from the synapse. 10 Voltage-Activated Ion Channels Voltage-activated (or voltage-gated) ion channels are proteinsembeddedintheneuron’s membrane that open and close in response to changes in voltage. These channels are crucial for action potential generation and propagation. Key Characteristics: o Open or close depending on membrane voltage. o Selective for specific ions (e.g., Na , K , Ca² ). o Essential for electrical signalling in neurons. Types of Voltage-Activated Ion Channels: o Voltage-Gated Sodium (Na ) Channels – Open during depolarization, allowing Na to rush into the neuron. o Voltage-Gated Potassium (K ) Channels – Open during repolarization, allowing K to exit the neuron. o Voltage-Gated Calcium (Ca² ) Channels – Found at synapses, allowing Ca² to enter and trigger neurotransmitter release. o Voltage-Gated Chloride (Cl ) Channels – Less common but can help regulate neuron excitability. Voltage-Activated Ion Channels during an Action Potential 1. Resting State (-70mV): a. Na and K voltage-gated channels are closed. b. The neuron is at its resting membrane potential. 2. Depolarization (Threshold Reached, ~ -55mV): a. Voltage-gated Na channels open, allowing Na to enter the neuron. b. The inside of the neuron becomes more positive. 3. Peak of Action Potential (~+30mV): a. Na channels inactivate (stop allowing Na in). b. Voltage-gated K channels open, allowing K to exit. 4. Repolarization: a. The neuron becomes more negative as K leaves. b. Na channels reset (ready to reopen for the next signal). 5. Hyperpolarization (~ -80mV): a. K channels close slowly, causing the neuron to become more negative than its resting state. b. The Na /K pump restores the resting potential. Refractory Periods The refractory period is the time after an action potential during which a neuron is either completely unable or less likely to fire another action potential. It ensures one-way signal transmission and regulates the frequency of nerve impulses. Types of Refractory Periods 1. Absolute Refractory Period o Occurs during depolarization and early repolarization. o All voltage-gated Na channels are either open or inactivated, meaning no new action potential can be triggered, no matter how strong the stimulus. 11 o Ensures that each action potential is separate and unidirectional. 2. Relative Refractory Period o Begins when enough Na channels reset and K channels are still open. o The neuron can fire only if the stimulus is stronger than usual, because: The membrane is hyperpolarized (more negative than resting potential). A greater influx of Na is needed to reach threshold. o This controls how frequently neurons can fire and allows adaptation to strong, repeated stimuli. Continuous Conduction Occurs in unmyelinated axons The action potential is propagated along the entire length of the axon. Each segment of the membrane depolarizes sequentially, causing a slower transmission speed. More energy is required because ion channels must open and close at every point. Example: Found in pain and autonomic neurons, where speed is less critical. Saltatory Conduction Occurs in myelinated axons The action potential jumps from one Node of Ranvier to the next, skipping the insulated sections. This dramatically increases speed while conserving energy. Example: Found in motor neurons and sensory neurons where rapid responses are necessary. Factors Affecting Velocity of the action potential 1. Axon: The long, tube-like extension of a neuron that transmits electrical signals. It carries action potentials from the axon hillock to the axon terminals, where neurotransmitters are released. The structure of the axon— particularly its diameter and myelination— determines how fast signals are transmitted. 2. Diameter: Larger diameter = faster conduction This is because wider axons have lower resistance, allowing ions to flow more freely inside the neuron. Example: Squid giant axons (used in early neuroscience research) are large- diameter axons that allow for rapid reflex responses. 12 3. Myelination: Myelin is a fatty insulating layer that wraps around axons, produced by Schwann cells (PNS) and oligodendrocytes (CNS). Myelination increases conduction speed by enabling saltatory conduction, where the action potential jumps between Nodes of Ranvier. Example: Motor neurons controlling muscle movement are highly myelinated for fast responses. Vesicles Definition Vesicles are small membrane-bound sacs inside cells that store and transport various substances, including neurotransmitters in neurons. They play a critical role in synaptic transmission, ensuring signals are efficiently passed between neurons. Types of Vesicles in Neurophysiology 1. Synaptic Vesicles o Found in the axon terminals of neurons. o Store neurotransmitters (e.g., dopamine, acetylcholine). o Release neurotransmitters into the synaptic cleft during synaptic transmission. 2. Dense-Core Vesicles o Larger than synaptic vesicles. o Store neuropeptides (larger signaling molecules). o Involved in modulating neuronal activity rather than fast synaptic transmission. Synaptic Signal Transduction When a neuron communicates with another neuron at a synapse, it can either excite or inhibit the next neuron. This process involves different types of postsynaptic potentials and receptor mechanisms that determine the neuron's response. Inhibitory postsynaptic potential (IPSP) Definition: An IPSP is a temporary hyperpolarization of the postsynaptic membrane, making it less likely for the neuron to fire an action potential. How It Happens: Neurotransmitters like GABA or glycine bind to inhibitory receptors. These receptors open chloride (Cl ) or potassium (K ) channels. 13 Chloride enters the neuron or potassium exits, making the inside more negative (further from the action potential threshold). Effect: Reduces neural activity, preventing excessive excitation in the brain. Important for functions like muscle relaxation and preventing seizures. Excitatory postsynaptic potential (EPSP) Definition: An EPSP is a temporary depolarization of the postsynaptic membrane, making it more likely for the neuron to reach threshold and fire an action potential. How It Happens: Neurotransmitters like glutamate bind to excitatory receptors. These receptors open sodium (Na ) or calcium (Ca² ) channels. Sodium or calcium enters the neuron, making the inside less negative (closer to the threshold). Effect: Increases neural activity, promoting communication between neurons. Critical for learning, memory, and sensory processing. Synaptic Signal Transduction 1. Temporospatial summation: Definition: The process by which multiple EPSPs and IPSPs combine over time (temporal) and space (spatial) to determine whether a neuron fires an action potential. Temporal Summation: When multiple signals from the same synapse arrive in quick succession, their effects add up. Spatial Summation: When multiple synapses on the neuron receive signals at the same time, their effects combine. Effect: If the total EPSPs > IPSPs, the neuron fires an action potential. If IPSPs > EPSPs, the neuron remains inhibited. 2. Synaptically evoked potential Definition: The electrical response of a neuron after it receives a signal from another neuron at a synapse. Can be excitatory (EPSP) or inhibitory (IPSP). 14 Used in research to study how neurons communicate. 3. Ionotropic Receptors Definition: Fast-acting receptors that directly open ion channels when neurotransmitters bind. How They Work: Neurotransmitterbinds:Ionchannelopens:Ionsflowin orout: Immediate response. Effect: Allows rapid neural communication, important for reflexes and fast synaptic transmission. 4. Metabotropic receptors Definition: Slower-acting receptors that indirectly influence ion channels by activating intracellular signaling pathways. How They Work: Neurotransmitterbinds:Activates G-protein :Triggersa cascade of chemical reactions :Eventually modulates ion channels or other cell functions. Effect: Leads to longer-lasting effects in the neuron, important for modulating neural circuits and memory formation. Neurotransmitters Neurotransmitters are chemical messengers that transmit signals between neurons. They can be excitatory, increasing neural activity, or inhibitory, reducing it. Below are the key neurotransmitters involved in neurophysiology. Glutamate GABA and glycine Acetylcholine Biogenic amines ATP Neuropeptides 15 Glutamate Function: Glutamate is the primary excitatory neurotransmitter in the brain. It is crucial for learning, memory, and synaptic plasticity (the ability of synapses to strengthen or weaken over time). Mechanism of Action: Binds to ionotropic receptors (AMPA, NMDA, and Kainate receptors), causing Na and Ca² influx, which depolarizes the neuron and increases firing. Also acts on metabotropic glutamate receptors (mGluRs), modulating neural activity. Clinical Relevance: Excess glutamate can lead to excitotoxicity, which damages neurons (seen in stroke, Alzheimer's, and epilepsy). Glutamate dysfunction is linked to schizophrenia and depression. GABA and glycine Function: GABA (gamma-aminobutyric acid) is the main inhibitory neurotransmitter in the brain. Glycine is the main inhibitory neurotransmitter in the spinal cord. Mechanism of Action: GABA binds to GABA-A (ionotropic) receptors, opening Cl channels, causing hyperpolarization (inhibition). GABA also binds to GABA-B (metabotropic) receptors, which activate intracellular signaling for longer inhibition. Glycine works similarly by opening Cl channels, preventing overexcitation. Clinical Relevance: GABA deficiency can cause seizures, anxiety, and insomnia. Drugs like benzodiazepines (e.g., Valium) and alcohol enhance GABA effects, leading to relaxation. Glycine dysfunction is linked to spasticity and movement disorders. 16 Acetylcholine Function: Used in the neuromuscular junction to control muscle contraction. Plays a key role in learning, attention, and memory in the brain. Important for autonomic nervous system function. Mechanism of Action: Binds to nicotinic receptors (ionotropic) for fast muscle contraction and fast synaptic transmission. Binds to muscarinic receptors (metabotropic) in the brain and autonomic nervous system for slower, modulatory effects. Clinical Relevance: Acetylcholine loss is linked to Alzheimer’sdisease. Myasthenia gravis is caused by autoimmune attack on nicotinic receptors, leading to muscle weakness. Neurotoxins like botulinum toxin (Botox) block ACh release, causing paralysis. Biologic amines Biogenic amines are a group of neurotransmitters derived from amino acids. They include: a) Dopamine (DA) Involved in reward, motivation, movement, and mood regulation. Dysfunction linked to Parkinson’sdiseaselow ( DA) schizophrenia (excess and DA). b) Serotonin (5-HT) Regulates mood, sleep, appetite, and cognition. Low levels associated with depression and anxiety (treated with SSRIs like Prozac). c) Norepinephrine (NE) & Epinephrine Control alertness, attention, and autonomic nervous function (fight-or-flight response). Low NE linked to depression, high NE linked to anxiety and hypertension. d) Histamine Involved in wakefulness, arousal, and immune response. Antihistamines (like Benadryl) block histamine in the brain, causing drowsiness. 17 ATP Function: ATP is primarily known for energy storage, but it also acts as a neurotransmitter. Released with other neurotransmitters to modulate synaptic activity. Mechanism of Action: Binds to purinergic receptors (P2X ionotropic, P2Y metabotropic) to influence neuronal excitability. Adenosine (a breakdown product of ATP) inhibits neural activity and promotes sleep. Clinical Relevance: Caffeine blocks adenosine receptors, leading to increased alertness. Neuropeptides Definition: Neuropeptides are small protein-like molecules that act as neurotransmitters or neuromodulators. They have longer-lasting effects than classical neurotransmitters. Examples & Functions: a) Substance P Involved in pain perception. Released in response to injury and inflammation. b) Endorphins & Enkephalins Act as natural painkillers, reducing pain perception. Bind to opioid receptors (same receptors as morphine and heroin). c) Oxytocin & Vasopressin Oxytocin regulates social bonding and trust. Vasopressin controls water balance and blood pressure. d) Neuropeptide Y (NPY) Regulates hunger, stress, and anxiety. Clinical Relevance: Opioid drugs (like morphine) mimic endorphins but can cause addiction. Dysregulated neuropeptides are linked to chronic pain, mood disorders, and appetite issues.