Biotechnology: Gene Transfer & DNA Sequencing (PDF)
Document Details
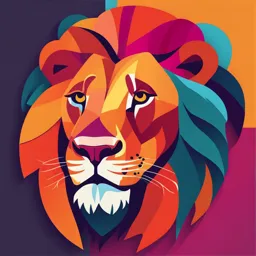
Uploaded by PhenomenalTinWhistle166
Tags
Summary
These lecture notes provide an overview of key concepts in biotechnology, including gene transfer, genetics, and DNA sequencing. They summarize the history and fundamental principles, focusing explicitly on the concepts and not on any particular exam.
Full Transcript
BIOTECHNOLOGY Objectives At the end of the lesson the learners should have: 1. Understood the meaning of genetically modified organisms(GMO) 2. Described processes in Genetic Engineering 3. Cited examples of plant and animal genetic modifications What is Genetics? Genetics Genetics is the stu...
BIOTECHNOLOGY Objectives At the end of the lesson the learners should have: 1. Understood the meaning of genetically modified organisms(GMO) 2. Described processes in Genetic Engineering 3. Cited examples of plant and animal genetic modifications What is Genetics? Genetics Genetics is the study of how genes and how traits are passed down from one generation to the next. The Phenomena of Gene Transfer One of the great mysteries of all times has been the ability of organisms to reproduce and create new organisms that resemble the parents. For thousands of years, humans have known that the offspring of plants and animals closely resemble their parents. Throughout most of history, they considered the transference of characteristics from parent to young as being part of the “miracle of birth”. Selective Breeding Even though people did not understand how this process worked, they made use of the phenomena through selective breeding. They selected seed to plant from the plants that they thought were superior to other plants. Animals that best suited their purposes were saved for breeding, and through-out several generations of genetic transfer, superior animals were developed. One phenomenon that people noticed was that although characteristics of parents were usually passed on to their offspring, offspring sometimes exhibited dramatically different characteristics. Gregor Mendel (1822–1884) Was an Austrian monk at the distinguished monastery of St. Thomas in the town of Brünn, now Brno, in the Czech Republic. High school teacher of physics and natural history. Conducted biological experiments in a small garden near the monastery. He carried out his famous experiments on crosses of garden peas (Pisum sativum) from 1856 to 1863. MENDEL’S EXPERIMENTS Mendel’s experiments with garden peas laid down the basic principles of inheritance. He chose garden peas for two main reasons. First, garden peas have several varieties that have observable, contrasting characteristics. Second, garden peas normally reproduce by self-pollination. How do you ensure cross pollination? By deliberately crossing two different varieties, that is, transferring the pollen of one to the pistil of another, he was able to follow the inheritance of a single, easily distinguishable trait. Mendel was able to produce hybrid offsprings for several contrasting traits by cross- pollination. Results: He made exact counts of the number of plants bearing a specific trait. It was from these quantitative data that Mendel deduced the principles governing inheritance. OBSERVATIONS From the results of his experiments, Mendel was able to make the following basic observations: The F1 generation showed only the dominant trait. In the F2 generation, both dominant and recessive traits reappeared in the offsprings. In the F2 generation, there were three times as many plants with dominant traits than plants with recessive traits. CONCLUSION Mendel concluded that the sex cells, or gametes, of garden pea plants contain factors that induced the appearance of a particular trait. For example, a certain factor caused an offspring to produce yellow seeds and another factor induced it to produce green seeds. Gene These factors Mendel was referring to were later called genes by a Danish biologist, Wilhelm Ludvig Johannsen, in 1909. Genes- a unit of heredity which is transferred from a parent to offspring and is held to determine some characteristic of the offspring Definition of terms Trait- is a specific characteristic of an individual. Traits can be determined by genes, environmental factors or by a combination of both. Traits can be qualitative (such as eye color) or quantitative (such as height or blood pressure). Gamete- an organism's reproductive cells. Also referred to as sex cells. Chromosome- A structure found inside the nucleus of a cell. A chromosome is made up of proteins and DNA organized into genes. Gene- The basic unit of heredity passed from parent to child. Genes are made up of sequences of DNA and are arranged, one after another, at specific locations on chromosomes in the nucleus of cells. Allele- one of two or more versions of DNA sequence (a single base or a segment of bases) at a given genomic location. An individual inherits two alleles, one from each parent, for any given genomic location where such variation exists. Locous/loci- the actual location of the gene on a region of a chromosome. Traits Dominant Trait Recessive Trait Dominant traits are always A trait that tends to be masked expressed (Law of by other inherited traits, mostly Dominance). by a dominant trait. Usually represented by a Usually represented by a small capital letter. letter. Definition of terms Phenotype- refers to the observable physical properties of an organism; these include the organism's appearance, development, and behavior. (blue eye color, purple flower, etc.). An organism's phenotype is determined by its genotype. Genotype- combination of alleles that they possess for a specific gene (BB, Bb, bb) Homozygous- refers to having inherited the same versions (alleles) of a genomic marker from each biological parent. Thus, an individual who is homozygous for a genomic marker has two identical versions of that marker. (BB, bb) Heterozygous- having different alleles for a particular trait. (Bb) Alleles The trait of a plant is called its phenotype and the pair of alleles for that trait is called its genotype. A plant with two similar alleles for a trait is termed homozygous and that with dissimilar alleles is heterozygous. MENDEL’S PRINCIPLES OF INHERITANCE Law of Dominance In a cross of parents homozygous for contrasting traits, the F1 hybrid generation expresses only one of the parental trait— the dominant trait—while the other parental trait which is the recessive trait not shown. “When parents with pure, contrasting traits are crossed together, only one form of trait appears in the next generation. The hybrid offsprings will exhibit only the dominant trait in the phenotype.” In this law, each character is controlled by distinct units called factors, which occur in pairs. If the pairs are heterozygous, one will always dominate the other. Heterozygous= Bb (phenotype is the dominant trait) Codominance Refers to a type of inheritance in which two versions (alleles) of the same gene are expressed separately to yield different traits in an individual. That is, instead of one trait being dominant over the other, both traits appear, such as in a plant or animal that has more than one pigment color. Incomplete Dominance Incomplete dominance pertains to the genetic phenomenon in which the distinct gene products from the two codominant alleles in a heterozygote blend to form a phenotype intermediate between those of the two homozygotes. Law of Segregation Mendel knew nothing about chromosomes and genes, but his idea about how factors are passed on from parents to offsprings reflects the exact behavior of genes and chromosomes during meiosis and fertilization. “During the formation of gamete, each gene separates from each other so that each gamete carries only one allele for each gene.” Mendel inferred that the two factors that determine a trait segregate when the sex cells are formed (which is during meiosis). He also speculated that each gamete (egg cell or pollen) is equally likely to contain either factor. These gametes will then unite at random in fertilization. What does this organism look like? Punnett Square Punnett Square Reginald Crundall Punnett (1875-1967) Punnett devised the "Punnett Square" to depict the number and variety of genetic combinations. Practice The gene for hair color in rabbits has two alleles Q and q. Q is dominant and codes for brown hair. q is recessive and codes for white hair. If you cross two brown rabbits both with heterozygous genotype, what is the ratio of brown-haired rabbits to white-haired rabbits that will probably be born? Answer Q q Brown heterozygous genotype QQ Qq (Qq x Qq) QQ = brown Q Qq = brown Qq = brown qq = white q Qq qq Ans: 3 brown rabbits to1 white rabbit (p. ratio: 3:1), (probability: 75%-25%), (g. ratio: 1 QQ:2 Qq:1qq) Law of Independent Assortment “The law of independent assortment states that the alleles of different genes are inherited independently within the organisms that reproduce sexually.” For example, the allele for pea color (Y/y) in pea plants may be accompanied by either the allele for round shape (R) in some gametes or the allele for wrinkled shape (r) in other gametes. The genes for pea color segregate independently from the genes for pea shape. In other words, the inheritance of one trait does not affect the inheritance of another. A purple flower can be located along the stem or at the end. A yellow seed can be round, and it can also be wrinkled. The same goes with the green seed. A tall plant can both yield yellow and green seeds. The same goes with a short plant. He reported all his findings to a local natural history society, which then published the findings and interpretations of his research in its scientific journal in 1866. It was years later (1900), when three scientists rediscovered his work and credited him for pioneering this field of genetics. Hugo de Vries Karl Correns Erich von Tschermak The three Europeans, unknown to each other, were working on different plant hybrids when they each worked out the laws of inheritance. 1900 When they reviewed the literature before publishing their own results, they were startled to find Mendel's old papers spelling out those laws in detail. Each man announced Mendel's discoveries and his own work as confirmation of them. Hugo de Vries Carl Correns Erich von Tschermak Hugo De Vries Dutch botanist and geneticist who introduced the experimental study of organic evolution. Cultivation of the evening primrose new forms or varieties appearing randomly among the host of ordinary specimens Carl Correns German botanist and geneticist In attempting to ascertain the extent to which Mendel’s laws are valid, he undertook a classic study of non- Mendelian heredity in variegated plants, such as the four-o’clock (Mirabilis jalapa). Erich von Tschermak Was an Austrian botanist. In the spring of 1898 Tschermak began breeding experiments on the garden pea in the Botanical Garden of Ghent. While writing the results of his experiments, Tschermak saw a cross-reference to Mendel’s work and had the papers sent to him from the library of the University of Vienna. He found that Mendel’s work with the garden pea duplicated and in some ways superseded his own. They helped expand awareness of the Mendelian laws of inheritance in the scientific world. By 1900, cells and chromosomes were sufficiently understood to give Mendel's abstract ideas a physical context. Finally, Mendel’s achievement was fully recognized only after 50 years. Subsequent research revealed that genetic transfer takes place within the cells of organisms. This research occurred over about the first 25 years of the century, and simultaneously knowledge of the existence of chromosomes was developed. Once this knowledge was established, scientists then discovered the molecular basis of inheritance during the next 25 year. The development of the electron microscope in the mid-1900s allowed scientists to thoroughly examine the inner workings of living cells. Around that time, two scientists, James Watson and Francis Crick of the Cavendish Laboratory in Cambridge, England, discovered how the building blocks of genetic trans-fer, known as deoxyribonucleic acid (DNA), are organized in the cell and the process by which they replicate. Scientists in the latter half of the twentieth century uncovered the molecular mechanisms by which genetic information in cells is encoded and decoded. This allowed them to better understand the way the molecules of inheritance functioned in the cell by carrying information about physical characteristics and behavior from one generation to the next. This, in turn, provided the means to finally begin to comprehend how genetic transfer functions. DNA SEQUENCING Higher-ordered animals and plants such as those produced in agriculture are composed of billions upon billions of cells. The DNA within the nucleus of each one of these cells contains a complete genetic code for creating an identical organism. DNA is composed of units called nucleotides that are made up of a sugar molecule, a phosphate molecule, and a nitrogen molecule containing chemicals called bases. The units or nucleotides of DNA are arranged together on a strand called a helix that resembles a long, twisted ladder. At each point on the helix, where the two halves of the ladder are connected, different nitrogen- containing bases— adenine (A), thymine (T), guanine (G), and cytosine (C)— are attached to each other at the center of the rung. These nitrogen bases are shaped so that each one can pair with only one particular base. Adenine (A) can pair only with thymine (T), and cytosine (C) can pair only with guanine (G) It is the sequence of DNA’s nucleotides that functions as the genetic code. Nucleotides are arranged in functional segments called genes that encode specific biological traits. This is what controls the creation of certain characteristics in the organism. Within each organism are sequences of different sizes. One sequence may be relatively short and control a certain trait. Another sequence may be relatively long and control a different trait. For example, as far as we know now, the shortest sequence in the human genetic code is about 50 nucleotides, and the longest contains about 250 million. Individual genes may work independently to govern biological traits. For example, one gene may control hair color in an animal, and another gene may control the hair length or the texture of the animal’s hair. Genes control the color of flowers, the amount of protein or carbohydrates in a seed, and the height of the plant at maturity. Different forms of the same gene are called alleles. Another factor in genetic transfer of characteristics is that of the additive expression of genes. This means that a number of different genes may be “added” together to produce a certain trait in an animal. For instance, the amount of milk produced by the female is controlled not by a single pair of alleles but by several pairs. The size and body capacity of the female, the ability to produce the proper amounts of hormones, and the mammary gland’s size and functioning ability are all controlled by different pairs of alleles. Yet all of these factors contribute to the female’s overall ability to produce milk. Genes are grouped together on dense physical structures known as chromosomes. Chromosomes are composed of chromatin, which is made up of DNA tightly wound around structural proteins, and are housed within the nucleus of each eukaryotic cell. Remember that eukaryotic cells have a nucleus and prokaryotic cells have no true nucleus. Chromosomes are also present in prokaryotic cells, though prokaryotic chromosomes are typically small, circular structures with a simple architecture. The biological purpose of chromosomes is to wind up and organize the DNA in a structure that is easy to duplicate and divide during cell division (mitosis). Chromosomes line up in the center of the cell and are carefully separated to make sure that each daughter cell receives a full copy of genetic information. Chromosomes may be classified as either autosomes or sex chromosomes. Autosomes are chromosomes that carry genes for all the traits of an organism except sex determination. Sex chromosomes are those that carry genes that will determine the gender (male or female) of an organism. A karyotype is an individual's complete set of chromosomes. The term also refers to a laboratory- produced image of a person's chromosomes isolated from an individual cell and arranged in numerical order. A karyotype may be used to look for abnormalities in chromosome number or structure. GENOME SEQUENCING (GENE MAPPING) What is a genome? The genome is the entire set of DNA instructions found in a cell. In humans, the genome consists of 23 pairs of chromosomes located in the cell’s nucleus, as well as a small chromosome in the cell’s mitochondria. A genome contains all the information needed for an individual to develop and function. Genomics Genomics is the study of an organism's genome – its genetic material – and how that information is applied. All living things, from single- celled bacteria, to multi-cellular plants, animals and humans, have a genome. The series and sequences of nucleotides arranged on chromosomes is the genetic code that controls all the characteristics of an organism that are passed from one generation to the next. A goal of scientists during the 1980s and 1990s was to figure out just how the nucleotide sequence of DNA for various organisms is arranged in ALL of the genes and chromosomes of the entire body of an organism. The reasoning behind the goal was that once we understood the arrangement of the nucleotides on the chromosomes, we could then begin unlocking the mechanisms that control certain traits. For example, if we know the precise gene that controls any number of genetically transmitted diseases in humans, the specific gene may be treated, and the disease may be eradicated. In fact, the control genes for several diseases, such as Parkinson’s disease, have already been identified—although it took scientists over 7 years to locate this specific gene. However, although we have a significant amount of DNA sequence data for humans and many other organisms, several important details need to be studied. For each gene in the database, numerous laboratory experiments must be carried out to characterize the biological function of the gene. In addition, (as previously mentioned), genes act in concert to yield certain traits. We have yet to understand how gene interactions are occurring within cells and in response to environmental signals. The complete DNA sequencing of the human—was completed in 2001 and scientists predicted that the human gene map would contain up to 150,000 genes. The smaller number came as a surprise, considering that lower-order worms may contain half as many genes as those of a human. Another surprise was that humans share around 200 genes with bacteria. The mystery now is how an organism as complicated as a human being could have only twice as many genes as that of a worm as well as sharing common genes with bacteria. The genome of humans, as well as hundreds of other species, is made available to the public on the National Center for Biotechnology Information (NCBI) website.