Biochemistry: Bioenergetics & Metabolism Lecture Notes PDF
Document Details
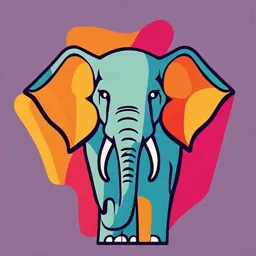
Uploaded by WellInformedMemphis
Beirut Arab University
Zeina Al Ariss
Tags
Related
- Biochemistry - Bioenergetics, Mitochondrial Electron Transport & Oxidative Phosphorylation PDF
- Biochemistry: Bioenergetics, Mitochondrial Electron Transport, and Oxidative Phosphorylation PDF
- Bioenergetics and Oxidative Phosphorylation PDF
- Bioenergetics and Oxidative Phosphorylation PDF
- Bioenergetics and Oxidative Phosphorylation PDF
- Bioenergetics and Oxidative Phosphorylation 2024 PDF
Summary
These lecture notes cover aspects of biochemistry, including bioenergetics and metabolism, with a focus on the respiratory chain and oxidative phosphorylation. The document contains detailed explanations of key concepts, diagrams, and pathways associated with energy production at the cellular level, with reference to the oxidation of NADH.
Full Transcript
Biochemistry PHAR 284 I. Bioenergetics & Metabolism Lecture 3 Dr. Zeina Al Ariss Faculty of Pharmacy Beirut Arab University I. Bioenergetics & Metabolism Respiratory Chain & Oxidative Phosphorylation...
Biochemistry PHAR 284 I. Bioenergetics & Metabolism Lecture 3 Dr. Zeina Al Ariss Faculty of Pharmacy Beirut Arab University I. Bioenergetics & Metabolism Respiratory Chain & Oxidative Phosphorylation Galal, A., Biochemistry for Pharmacy Students First Edition Respiratory Chain The respiratory chain (or electron transport chain) is composed of several protein complexes located in the inner mitochondrial membrane; these protein complexes are arranged in an order of decreasing reduction potential and act as electron carriers. Electrons (or –H) from NADH and FADH2 flow down the respiratory chain, so that a given carrier donates electrons to the following carrier (i.e. reduces it) and becomes itself oxidized. At the end, electrons reach their final acceptor, O2, which becomes reduced to H2O. This electron transfer is coupled to the formation of ATP; thus it is collectively known as oxidative phosphorylation. Components of the Respiratory Chain Components of the respiratory chain are contained in 4 large protein complexes in the inner mitochondrial membrane. Reactions involve flow of electrons (dotted line ) through these complexes, coupled with pumping ……………. of protons from the mitochondrial matrix to the intermembrane space with complexes I, III & IV, but not with complex II because energy released is not enough to pump protons. Complex I (NADH - Q Oxidoreductase) catalyzes the electron transfer from NADH to Q (co-enzyme Q or Q10 or ubiquinone). NADH + Q + 5 H+Matrix NAD+ + QH2 + 4 H+ intermembrane space Complex II (Succinate - Q Reductase) catalyzes the electron transfer from FADH2 to Q (FADH2 is formed in the Krebs cycle during the conversion of succinate to fumarate by succinate dehydrogenase). Complex III (Q – Cytochrome c Oxidoreductase) catalyzes the electron transfer from QH2 to Cytochrome c, which is an iron-containing dehydrogenase in which the iron atom oscillates between Fe3+ and Fe2+ during oxidation- reduction reactions. QH2 + 2 Cyt c (Fe3+) + 2 H+Matrix Q + 2 Cyt c (Fe2+) + 4 H+ intermembrane space Complex IV (Cytochrome c Oxidase) catalyzes the electron transfer from reduced cytochrome c to O2; cytochrome c oxidase contains copper atoms necessary for its functions, and is the only redox component in the chain that can directly react with O2. 4 Cyt c (Fe2+) + O2 + 8 H+Matrix 4 Cyt c (Fe3+) + 2H2O + 4 H+ intermembrane space Phosphorylation & ATP Synthase The flow of electrons from NADH or FADH2 to O2 does not directly result in phosphorylation of ADP to ATP. Alternatively, electron transfer is associated with pumping of protons from the mitochondrial matrix to the intermembrane space through specific H+ channels. As previously mentioned, this pumping is catalyzed by complexes I, III & IV, which can be considered as proton pumps. Actually, the pumped protons are the true driving force (proton-motive force) for ATP production as follows: 1. The inner mitochondrial membrane is impermeable to ions; therefore, protons pumped to the intermembrane space accumulate creating a proton gradient. 2. The proton gradient causes the flow of protons through proton channels in the Fo domain of ATP synthase (contained in complex V) leading to its rotation. 3. Rotation of the Fo domain leads to conformational changes in the F1 domain allowing it to bind to ADP and Pi, followed by phosphorylation of ADP to ATP, and finally release of ATP. Therefore, phosphorylation is tightly coupled to oxidation (hence the name oxidative phosphorylation) and occurs at the 3 sites of proton pumping in the respiratory chain. The respiratory chain extracts energy from reduced co-enzymes. Oxidation of Extra-Mitochondrial NADH NADH-linked dehydrogenase of the respiratory chain can accept electrons only from NADH generated in the mitochondrial matrix. Given that extra- mitochondrial NADH is unable to penetrate mitochondria, then, how is it oxidized in the respiratory chain? Special systems (known as the shuttle systems) can indirectly carry NADH generated outside the mitochondria (e.g. NADH generated in the cytosol in aerobic glycolysis) into the mitochondria. There are 2 shuttle systems: the malate-aspartate shuttle and the glycerol-3-phosphate shuttle. 1. Malate – Aspartate shuttle: This shuttle operates in the liver, kidney and heart. Steps: NADH reduces oxaloacetate to malate by the action of cytosolic malate dehydrogenase. Malate enters the mitochondria via a specific transporter. NADH enters the respiratory chain producing 3 ATPs. 2. Glycerol-3-phosphate shuttle: This shuttle operates in the skeletal muscle and brain. Steps: NADH reduces dihydroxyacetone phosphate to glycerol-3-phosphate. Glycerol-3-phosphate enters the mitochondria via a specific transporter. FADH2 enters the respiratory chain producing 2 ATPs. Regulation of oxidative phosphorylation: The activity of oxidative phosphorylation is tightly regulated by the cellular energy needs, and is limited by the availability of ADP as substrate for phosphorylation. If ADP is available and there is enough phosphate, ADP and Pi are converted to ATP, which is used in energy-requiring cellular activities. Interference with Oxidative Phosphorylation As previously mentioned, oxidative phosphorylation involves a tight coupling between electron transfer and generation of ATP. Different types of interferences exist, these are: 1. Blocking of electron transfer: Inhibitors of the respiratory chain block electron transfer at different sites in the chain with subsequent inhibition of ATP synthesis. The general rule is that all electron carriers can enter the respiratory chain ONLY after the block, where they can generate ATP. Rotenone (insecticide) or amytal (barbiturate) block electron transfer from NADH to Q; the oxidation of substrates generating NADH is therefore blocked. However, substrates that generate FADH2 can still enter the chain, by-passing the previous step, with the generation of 2 ATPs. Antimycin A (fish poison) blocks electron transfer to cytochrome c. However, substrates can still enter the chain, by-passing the 2 previous steps, with the generation of 1 ATP. Cyanide or carbon monoxide or H2S (fatal poisons) inhibit cytochrome c oxidase, with no generation of ATP. 2. Inhibition of ATP synthase: Oligomycin (toxic antibiotic) binds to the Fo domain of ATP synthase closing the H+ channels. This prevents re-entry of protons into the mitochondrial matrix, thus preventing phosphorylation of ADP to ATP. Because of the accumulation of protons in the intermembrane space, electron transport stops because of the difficulty of pumping additional protons. Therefore, electron transfer is blocked indirectly through the inhibition of phosphorylation, which indicates the tight coupling of the two processes. 3. Uncoupling of phosphorylation from electron transfer: Uncouplers are compounds that provide an alternative path for accumulated protons in the intermembrane space to return to the mitochondrial matrix rather than the F0 domain of complex V. Therefore, ATP is not synthesized and energy is rather released as heat, i.e. electron transfer proceeds while phosphorylation is not allowed, i.e. electron transfer & phosphorylation are uncoupled. Examples of uncouplers include: Dinitrophenol (DNP), which was highly used in 1930s in diet pills; DNP uncouples electron transfer & phosphorylation, which makes ATP production less efficient; as a result, cells counteract the lowered yield of ATP by oxidizing more stored reserves of carbohydrates and fats. Aspirin & other salicylates, which cause hyperthermia in overdose. Thermogenin, which is a natural uncoupling protein present in the inner mitochondrial membrane of brown fat of the newborn (fuel oxidation produces heat rather than ATP to keep the newborn warm). Thermogenin is also present in hibernating animals to adapt them to cold weather. Clinical Aspects I- Mitochondrial encephalomyopathy Mutation in mitochondrial genes that encode protein complexes of the respiratory chain can cause disease in the brain and skeletal muscle, being highly dependent on ATP supply. This disease is known as mitochondrial encephalomyopathy, and is prevalent in 16 out of 100,000. The condition is manifested as progressive loss of vision in early adulthood, epilepsy and muscle weakness. Management includes supplementation with: Ubiquinone, in case of Q10 deficiency. Succinate, in case of complex I deficiency, where succinate can directly donate electrons to complex II. Vitamin C, in case of complex III deficiency, where vitamin C can directly donate electrons to cytochrome c. Creatine, to increase phosphocreatine thus enhancing muscle strength. II- Statins inhibit the body synthesis of mevalonate, which is a precursor of both, cholesterol (hence their cholesterol lowering effect) and ubiquinone (thus causing rhabdomyolysis i.e. myotoxicity as a major side effect).