Biochemistry of Muscle Tissue PDF
Document Details
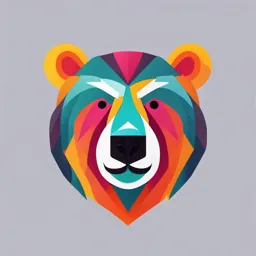
Uploaded by BestSellingTulip
Northeastern University
Victor Markus, PhD
Tags
Summary
This document provides a detailed overview of muscle tissue biochemistry. It covers topics such as the different types of muscles, the energy sources they use, and the process of muscle contraction. The document also explains the role of various proteins involved in these processes. It is suitable for undergraduate-level study.
Full Transcript
BIOCHEMISTRY OF MUSCLE TISSUE Asst. Prof. Victor Markus, PhD Department of Medical Biochemistry, Faculty of Medicine, NEU 1 BIOCHEMISTRY OF MUSCLE TISSUE Muscle tissue is a major biochemical transducer (mach...
BIOCHEMISTRY OF MUSCLE TISSUE Asst. Prof. Victor Markus, PhD Department of Medical Biochemistry, Faculty of Medicine, NEU 1 BIOCHEMISTRY OF MUSCLE TISSUE Muscle tissue is a major biochemical transducer (machine) that converts potential (chemical) energy into kinetic (mechanical) energy. Muscle, the largest single tissue in human body, makes up about 25% in babies, more than 40% in young adult and less than 30% in the aged adult. 3 types of muscles: Smooth muscle Skeletal muscle Cardiac muscle (unstriated, (striated, voluntary (striated, involuntary) involuntary) nervous control) Examples of the smooth muscles are muscles of the gastrointestinal tract, cardiovascular (blood vessel and lymphatic vessels), renal: (urinary bladder), genital (male and female reproductive tracts), respiratory tract, integument (erector pili of the skin), and sensory (ciliary muscle and iris of the eye) 3 DEVELOPMENT OF MUSCLE TISSUE * * * * * Myogenic regulatory factors (MRFs) :DNA-binding transcription factors with a helix-loop-helix (HLH) structure. DEVELOPMENT OF MUSCLE TISSUE – skeletal myogenesis Myogenin Profileration Differentin Differentiation Precursor Myoblast Committed Muscle cell Myostatin myoblast Cdk2; cyclin-dependent kinase-2, p21; an Cdk inhibitor, Rb; tumor suppressor protein (retinoblastoma). Myogenic regulatory factors (MRFs) DNA-binding transcription factors, Myo D and Myf 5:controlled by PAX3 and PAX7 homeotic genes Myogenin controls the myoblasts to be committed for terminal differentiation Myo-D, an early marker of muscle differentiation (from stem cells to myoblasts) Committed myoblasts do not divide, but fuse with each other and form multinucleated muscle fibers. The number of muscle fibers does not change after birth. Myogenin Profileration Differentin Differentiation Precursor Myoblast Committed Muscle cell Myostatin myoblast Myostatin inhibits proliferation of myoblasts Myogenic cells respond to myostatin by: downregulating the expression of Pax-3 and Myf-5, transcriptional regulators of myogenic cell proliferation Follistatin (a myostatin inhibitor) increases muscle mass 6 Genetic mutation or inhibition of myostatin produces a huge amount of muscle tissue Belgian Blue Mutations in the gene encoding myostatin leading to its inhibition explain the double muscled (muscular hypertrophy) Belgian Blue and Piedmontese cattles ENERGY SOURCES OF MUSCLE TISSUE ATP is not stored to a great degree in cells. Once muscle contraction starts the regeneration of ATP must occur rapidly. 3 primary sources of ATP (in order of utilization) Creatine phosphate (CP) cycle, Anaerobic glycolysis, Aerobic glycolysis and oxidative phosphorylation Energy from ATP derives from cleaving of terminal phosphate of ATP (ATP→ADP + Pi) 8 Creatine phosphate (CP) cycle Creatine phosphate (CP) cycle converts ADP back to ATP by donating its phosphate to ADP by the catalysis of creatine kinase (CK)/creatine phosphokinase (CPK) Fast but short lived: up to 30 seconds (cells do not store high amounts of CP) However, during short, high intensity contractions (sports requiring bursts of speed or power such as sprints of 10 seconds or less in duration) CP serves as the major source of energy. This form of energy generation neither produces lactate nor requires oxygen. 9 ENERGY METABOLISM of MUSCLE Creatine phosphate cycle H2O H2O Pi Pi ATP ATP ADP ADP CK CK Creatine Creatine Creatine Creatine phosphate Phosphate Exercise Resting Creatine formed during exercise is excreted by urine. Anaerobic glycolysis As soon as muscle contraction starts, the process of anaerobic glycolysis also begins. Anaerobic glycolysis does not contribute as large an amount of energy as CP in the short term, but its contribution is likely to last from 30 - 60 seconds. The major substrate for anaerobic glycolysis is muscle glycogen and possibly some blood born glucose. Lactate is formed as the end product. 11 Aerobic glycolysis and oxidative phosphorylation The final, and virtually limitless supply of energy. Energy production rate not as high as from glycolysis → Aerobic events like the marathon are run at a considerably slower pace than a 440-yard dash (quarter- mile race) because of this fact. The substrates for oxidative metabolism are primarily glucose and free fatty acids, although protein can also act as an energy source through intermediate conversions to glucose, glucose precursors or free fatty acids. 12 USAGE OF ENERGY SOURCES DURING MUSCULAR CONTRACTION 45 40 Phosphocreatine 40 Kcal / kg / hour 30 30 25 Anaerobic Glycolysis 20 15 Aerobic Glycolysis 10 0 0 10 20 30 40 50 60 70 80 90 100 Time (Seconds) Involves 2 organs, muscle and liver, functions in anaerobic conditions Contracting muscles produce lactate (anaerobic GLYCOLYSİS) → to liver. In the liver GLUCONEOGENESIS converts lactate to pyruvate and glucose. Glucose is transported to the muscle and LDH LDH lactate is produced by anaerobic glycolysis. Anaerobic glycolysis and the Cori cycle goes on till oxygen is sufficient. Under aerobic conditions (sufficient oxygen), glucose is metabolised via aerobic glycolysis, to pyruvate and acetyl CoA and acetyl CoA enters TCA cycle 14 Cori/Lactic acid cycle LIVER Blood MUSCLE Glucose Glucose LDH LDH (2) Pyruvate (2) Lactate (2) Lactate (2) Pyruvate Lactate GLYCOLYSIS GLUCONEOGENESIS Involves 2 organs, muscle and the liver. During extended periods of FASTING, skeletal muscle protein is degraded as an alternative source of energy. Alanine is the major amino acid present when muscle protein is degraded. The amino group transported from the muscle to the liver in the form of alanine, is converted to urea in the urea cycle and excreted. The C skeleton of alanine is converted to glucose through pyruvate. Glucose-alanine cycle eliminates nitrogen while replenishing the energy supply (glucose) for muscle. 16 17 STRIATED MUSCLE TYPES AND PROPERTIES Slow-twitch, aerobic Fast-twitch, anaerobic STRIATED MUSCLE TYPES AND PROPERTIES Two types of striated muscle cells: Slow-twitch muscle (Red muscle): They contract slowly and they obtain their energy from aerobic metabolism: oxidative type cells. They appear red due to their heme content from myoglobin and mitochondrial heme containing proteins. Fast-twitch muscle (white muscle): They contract rapidly and they obtain their energy mainly from anaeorobic glycolysis: nonoxidative type cells. It uses ATP faster than it can replace it. They appear light pink because they have few mitochondria but no myoglobin. STRUCTURE OF SKELETAL MUSCLE repeats of sarcomere units Sarcomere : Basic contractile unit of muscle Myofibrils: composed of thick and thin filaments. Thick filaments are composed of the protein myosin; thin filaments are composed of the protein The contraction of a striated muscle fiber occurs as the sarcomeres, linearly arranged within actin. myofibrils, shorten as myosin heads pull on the actin filaments. 21 22 THICK FILAMENTS- MYOSIN 23 THICK FILAMENTS- MYOSIN Myosin II: 55% of muscle protein, 4th most abundant protein in human body (Collagen, actin, hemoglobin, myosin and keratin). Two heavy (HC) and four light chains (LC) Of the four LC’s: 2 essential:ELC, two regulatory:RLC (Essential light chain-18 kDa)) (Regulatory light chain) Trypsin treatment of myosin will divide myosin into two pieces: 1. Light meromyosin (LMM: Large part of the fibrous tail) has no ATPase activity and does not bind to F-actin. 2. Heavy meromyosin (HMM: Globular head + small fibrous tail; has ATPase activity and binds to F-actin (fibrous actin) 18 kDa polypeptides are essential light chains. 22 kDA light chains are regulatory and their phosphoryl- ation starts muscle contraction. (Thin Filament) 27 THIN FILAMENTS – ACTIN (F-Actin) Globular/monomeric actin (G- G-ACTIN F-ACTIN (Globular actin) (Fibrous actin) actin) polymerizes to form actin basic structure of thin filaments filaments (F-actin) by the hydrolysis of ATP. Polymerization requires Mg++ and K+. F-actin: basic structure of the thin filaments in sarcomere. F-actin helix, formed from 2 identical F-actins Regulatory proteins: TROPONIN and TROPOMYOSIN 4 Ca++ Ca ++ Two tropomyosin chains intertwine F-actin helix Troponin C Troponin C and stabilize it. ( - Ca++) Troponin complexes are added on this structure at regular intervals: Troponin T ✓ TnC subunit binds Troponin I Ca2+, ✓ TnI inhibits binding of myosin to F-actin , and Troponin C ✓ TnT binds to Tropomyosin tropomyosine. Actin Regulatory proteins: TROPONIN and TROPOMYOSIN 4 Ca++ Ca ++ Troponin C Troponin C ( - Ca++) Troponin I Troponin T Relaxed state Tropomyosin binds to troponin to form a troponin-tropomyosin Troponin C complex Tropomyosin Tropomyosin winds around Actin prevents myosin actin filament and covers the “heads” from binding to myosin-binding sites to active sites on actin prevent actin from binding to myosin. Muscle Fibre 31 Transverse tubules (T-tubules): invaginations of sarcolemma (plasma membrane) penetrating into skeletal and cardiac muscle cells T-tubules conduct electrical impulses. SR stores calcium and regulates Two terminal cisternae (SR) and one T-tubule intracellular calcium levels T-tubules contain ion channels that allow for electrical impulses (action potentials) travelling along the sarcolemma, to enter rapidly into the cell, to initiate muscle contraction. 32 MUSCLE CONTRACTION 1. When a nerve impulse reaches a skeletal muscle cell, it causes a change in electric potential across plasma membrane (depolarization) 2. Ca2+ release from SR: rise in cytosolic Ca2+ (chemical signal), initiates contraction (3) 4. Relaxation (Resting) of a Muscle Fiber: Ca++ ions are pumped back into SR by Ca-ATPase Sliding Filament Model for Muscle Contraction CALCIUM CONTROLS MUSCLE CONTRACTION When a muscle is relaxed, myosin- binding site on actin filament is blocked by tropomyosin. The first step for contraction is Ca++ to bind to troponin so that tropomyosin can slide away from the binding sites on the actin strands. This allows the myosin heads to bind to these exposed binding sites and form cross-bridges. Actin filaments are then pulled by myosin heads to slide past the thick filaments toward the center of the sarcomere. 34 Sliding Filament Model for Muscle Contraction HOW CALCIUM CONTROLS MUSCLE CONTRACTION Troponin-Ca++ complex pulls tropomyosin away, exposing myosin binding sites on actin 35 ROLE OF CALCIUM IN MUSCLE CONTRACTION Myosin head can NOT Myosin head can bind to actin bind to actin Sliding Filament Model for Muscle Contraction actin myosin When signaled by a motor neuron a sarcomere contracts (shortens), thin and thick filaments overlap. Thin (actin) filaments slide past thick (myosin) filaments during muscle contraction. Sliding can only occur when myosin-binding sites on the actin filaments are exposed by a series of steps that begins with Ca++ entry into the sarcoplasm. 37 MOVEMENT OF MYOSIN ON F-ACTIN Sliding filament theory Troponin-Ca++ complex pulls tropomyosin away, exposing myosin binding sites on actin Contraction of a Muscle Fiber A cross-bridge forms between actin and the myosin heads triggering contraction. As long as Ca++ remains in sarcoplasm to bind to troponin, and as long as ATP is available, the ATP hydrolysis by myosin heads muscle fiber will continue to contract/shorten. Contraction of a Muscle Fiber 39 Relaxation of a Muscle Fiber. Ca-ATPase Ca++ ions are pumped back into SR, which causes the tropomyosin to reshield the binding sites on actin strands. Relaxation of a Muscle Fiber 40 Cross-bridge attaches. ATP breakdown provides energy to ready the myosin head for a power stroke Myosin head attaches to exposed binding site on actin and the power stroke is accomplished 4 STEP 3 Cross-bridge (myosin head) springs from raised position and pulls on the actin filament. 5 STEP 4 Cross bridges break ATP binds to cross-bridge (but is not yet broken down) Myosin heads are released from actin 6 STEP 5 As long as calcium ions and ATP are present, this walking continues until the muscle fiber is fully contracted 41 THE WALKING OF MYOSIN ON ACTIN Myosin complex Actin helix Tropomyosin OTHER MUSCLE PROTEİNS Protein Location Comment or Function Reaches from the Z- The largest protein in the body. Titin line to the M-line Important in the relaxation of muscle. From Z-line along Regulate assembly and length of actin Nebulin length of actin filaments filaments Anchors actin to Z- Attach the actin filaments to Z-line and -Actinin lines stabilizes Lateral organisation of myofibrils and Desmin and Lies alongside actin filaments their attachment to sarcolemma Vimentin (plasmalemma) Sarcoplasmic Binds calcium ions tightly and hold them Calsequestrin reticulum in cysterna of sarcoplasmic reticulum C-protein At the M-line Attach myosin to titin Organisation of myosin filaments to M-protein At the M-line opposite directions Inside of Attachment of some specific Dystrophin Plasmalemma glycoproteins to plasmalemma. TITIN (Connectin) Titin is the third most abundant protein in muscle (after myosin and actin), Titin is the largest known protein (~3.5 MDa). It connects the Z line to the M line in the sarcomere. Titin is important in the relaxation of striated muscle tissues. Controls the assembly of the myosin myofilament by acting as a template Mutations in titin gene are associated with familial hypertrophic cardiomyopathy (thickened heart muscle, the most common cause of sudden cardiac death) Titin Ca++ BINDING PROTEIN - CALSEQUESTRIN a calcium-binding protein of the SR, helps SR store large amounts of Ca++ helps to hold calcium in the cisterna of SR after muscle contraction, even though the concentration of calcium in SR is much higher than in cytosole SR Ca++-ATPase actively pumps Ca++ back into SR where Ca rebinds to calsequestrin Ca++-ATPase SR DYSTROPHIN a rod-shaped cytoplasmic protein, between the sarcolemma (muscle cell membrane) and the outermost layer of myofilaments in the muscle fiber links actin filaments to the surrounding extracellular matrix DG: Dystro-Glycan, SG: Sarcospan Dystrophin supports muscle fiber strength, and the absence of dystrophin reduces muscle stiffness, increases sarcolemmal deformability, 47 Dystrophin deficiency causes Duchenne’s muscular dystrophy a X-linked recessive degenerative muscle disease characterized by a defective dystrophin gene. 48 Duchenne’s muscular dystrophy 49 contract rhythmically 50 PROPERTIES OF CARDIAC MUSCLE CELLS Cardiac myocytes contract rhythmically. Rhythmic contraction is controlled by extracellular Ca2+. Ca2+ enters the cell through voltage-gated Ca2+ channels. During rest, most of the cytosolic Ca2+ is exported from myocyte by Ca2+- Na+ exchanger pump, the contribution of Ca2+-ATPase is minor. Contain many mitochondria and high amounts of myoglobin. 51 SMOOTH MUSCLE CELLS They have a single nucleus and NO myofibrils. They DO NOT contain troponin. The sarcoplasmic reticulum is not as well developed as in the striated muscles. T tubules are not present/rudimentary. Smooth muscle contraction is also controlled by Ca2+. Contraction causes cell shortening Contraction starts with the and phosphorylation of myosin light chain. a change in shape from elongated to globular. 52 Smooth muscle contraction is also controlled by Ca2+. BUT in smooth muscle contraction Ca+2 binds to CALMODULIN (no troponin in smooth muscle) 53 When intracellular calcium levels increase, the calcium is bound to calmodulin. Ca 2+- calmodulin is bound to myosin light-chain kinase to form a Ca 2+ - calmodulin- kinase complex. This complex catlayzes the phosphorylation of one of the two myosin light chains on the myosin heads. That phosphorylation allows binding of actin to myosin. A specific phosphatase dephosphorylates the myosin light chain, which returns the actin and myosin to the inactive, resting state. The actin-tropomyosin interactions are similar in smooth and skeletal muscle. 54 CONTRACTION OF SMOOTH MUSCLES Troponin is absent in smooth muscle, so the high-speed actin-myosin interaction regulatory system found in striated muscle is not operative in smooth muscle. 56 Smooth muscle relaxation by caldesmon Caldesmon (CD) and calponin, in smooth muscle cells, at low [Ca2+] bind to tropomyosin (TM) and actin, thus prevent the actin-myosin interaction and keep muscle in the RELAXED state. Smooth muscle myosin thick filament composed of myosin heavy chains and light chains (essential and regulatory)and thin filament composed of actin, tropomyosin, caldesmon, and calponin. P: phosphorylated myosin head. 57 REGULATION OF SMOOTH MUSCLE CONTRACTION 1. A low-speed alternative regulatory system is present in smooth muscle cells, based on the actin-binding protein CALDESMON. 2. When caldesmon is bound to actin it blocks actin - myosin interaction and contraction is inhibited → Relaxation 3. Caldesmon can be removed from actin either by phosphorylation using MAP kinase or by Ca2+-calmodulin complex in presence of Ca2+. 4. Ca2+-calmodulin complex also activates the enzyme, myosin light chain kinase (MLCK). 5. Actin and activated myosin associate and muscle cells contract. 58 Regulation of smooth muscle contraction by caldesmon At low Ca2+ concentrations caldesmon binds to tropomyosin and actin, reducing the binding of myosin to actin and keeping muscle in the relaxed state. At higher Ca2+ concentrations, a Ca2+-calmodulin complex binds to caldesmon, releasing it from actin; thus myosin can interact with actin and the muscle can contract. Phosphorylation by several kinases, including MAP kinase, and dephosphorylation by phosphatases also regulate caldesmon’s actin-binding activity. CD; Caldesmon, TM; Tropomyosin, CaM; Calmodulin 59 MUSCLE TYPES and PROPERTIES Skeletal Cardiac Smooth Cross striations (sarcomeres) Cross striations (sarcomeres) NO striations Small T tubules Large T tubules No or rudimentary T-tubules S.R. well developed and Ca2+ S.R. present and Ca2+ pumps act S.R. often rudimentary and Ca2+ pumps act rapidly relatively rapidly pumps act slowly No hormone receptors on Hormone (epinephrine) Several hormone receptors, receptors on plasmalemma( - plasmalemma and -adrenergic). depending on location Nerve impulses initiate Contraction initiated by nerve Has intrinsic rhythmicity contraction impulses, hormones, etc. NOT dependant on ECF dependant on ECF [Ca2+] for dependant on ECF [Ca2+] for [Ca2+] for contraction contraction contraction Troponin is the regulatory Ca Troponin is the regulatory Ca NO Troponin, binding protein binding protein Calmodulin is the regulatory Ca binding protein NO caldesmon and calponin NO caldesmon and calponin Caldesmon and calponin are major regulatory proteins REFERENCES Biochemistry of Muscle Tissue Lecture Note, Prof. Nevbahar TURGAN, Near East university, Nicosia, North Cyprus. Harper's Illustrated Biochemistry, 31edition. Victor W. Rodwell, David A. Bender, Kathleen M. Botham, Peter J. Kennelly, P. Anthony Weil., 2018 Medical Biochemistry, 4th Edition. John Baynes , Marek Dominiczak, 2014 The Medical Biochemistry Page: themedicalbiochemistrypage.org vivo.colostate.edu