BioChem Makeup Final Review Lectures 1-22 PDF
Document Details
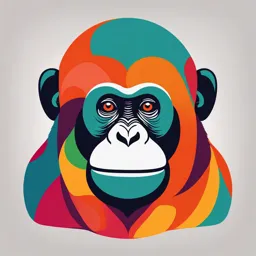
Uploaded by AmazingEiffelTower
West Virginia University
Tags
Summary
These are lecture notes from a biochemistry course, covering lectures 1-22. The notes discuss various topics including biomolecules, water properties, biochemical reactions, enzyme kinetics, and more. The document is formatted as a PDF.
Full Transcript
Lecture 1: Biomolecules Distinguish different organic bio-molecules by their family name, group structure, group name and significance List the 4 major classes of small bio-molecules, the polymers/macromolecules they form and identify each based on their chemical structure...
Lecture 1: Biomolecules Distinguish different organic bio-molecules by their family name, group structure, group name and significance List the 4 major classes of small bio-molecules, the polymers/macromolecules they form and identify each based on their chemical structure ○ ○ ○ ○ ○ ○ Lecture 2: Water Structure and Properties Diagram a water molecule ○ Label positive and negative dipole moments ○ Diagram H bonds between water molecules Describe hydrogen bonds ○ Properties: electrostatic in nature and partially covalent in nature (shares electrons) ○ Positive dipole on the H+ is attracted to the negative dipole on the O of another water molecule ○ Whenever H is covalently bonded to an O or an N, the molecule forms a dipole- these can form H bonds ○ Recognize functional groups that hydrogen bond Recognize functional groups that hydrogen bond ○ ○ Functional groups that can participate in hydrogen bonding include: hydroxyl (-OH), amine (-NH2), amide (-CONH2), carboxylic acid (-COOH), and sometimes nitrile (-CN), as they all contain a hydrogen atom bonded to a highly electronegative atom like oxygen or nitrogen, allowing them to act as hydrogen bond donors; additionally, the lone pairs on the oxygen or nitrogen atoms in these groups can act as hydrogen bond acceptors Describe properties of water ○ Thermal properties of water, solvent properties of water Liquid at most of the range of temperatures on earth due to H bonding Water forms H bonds with 4 other waters as ice - need to break H bonds to melt and boil Water is an ideal biological solvent Water can dissolve charged and polar substances Water is attracted to charged ions and as ions are hydrated their attraction to each other decreases as they dissolve Water has a high dielectric constant - capacity to reduce electrostatic attractions between charges ○ Interactions with hydrophilic, hydrophobic, and amphipathic molecules Hydrophobic molecules do not interact with water: this is a driving force for membrane formation and protein folding Amphipathic: part of the molecule is hydrophilic and part of the molecule is hydrophobic Explain osmosis ○ Spontaneous passage of water (solvent) through a semipermeable barrier Driven by concentration of solute ○ Understand the concept of osmotic pressure Pressure required to stop the net flow of water (solvent) ○ Define isotonic, hypotonic, and hypertonic Isotonic: inside = outside Hypotonic: inside > outside Hypertonic: inside < outside Lecture 3: Water ionization, pH, Buffers ○ pH = -log(H+) ○ Acid: can donate a proton ○ Base: can accept a proton ○ Ka: acid dissociation constant Ka= (H+)(A-)/(HA) pKa= -log(Ka) ○ Acid functional groups: presence of hydrogen ○ Base functional groups: absence of hydrogen ○ Buffers: maintain a relatively constant pH Commonly mixtures of weak acids and conjugate bases ○ Acidosis: blood pH < 7.35 ○ Alkalosis: blood pH > 7.45 ○ Physiological buffers Bicarbonate buffer: important in blood Phosphate buffer: important in cells Proteins as buffers: important in living organisms ○ Henderson-Hasselbalch Equation pH= pKa + log (A-)/(HA) Lecture 4: Biochemical Reactions ○ Nucleophilic substitutions Nucleophile attacks electrophile, displaces leaving group Electrophile is often a carbonyl carbon of an ester, amide, or anhydride Anhydride: molecule with two carbonyl groups linked via oxygen ○ Elimination reactions Atoms lost and a double bond forms ○ Addition reaction 2 reactants combine to form 1 ○ Isomerization Changes order but no gain or loss of electrons ○ Oxidation/Reduction Transfer of electrons O gains or loses H gains or loses One molecule is oxidized where the other is reduced NAD+/NADH often participates in oxidation/reduction reactions ○ Energy: capacity to do work ○ In life most energy comes from oxidation/reduction reactions ○ In cells when an electron is transferred energy is released Cells have mechanisms to capture and store some of this energy A series of redox reactions in the cell release energy that is used to generate ATP, the main energy carrying molecules in cells The more reduced a molecule is (more H+), the more energy it has ○ Metabolism Sum of all enzyme catalyzed reactions (3 pathways) Metabolic ○ Anabolic pathway Biosynthetic and uses energy ○ Catabolic pathway Degradation and some release energy Energy transfer ○ Captures energy and transforms it into a useable source Signal transduction ○ Receive and respond to signals ○ Receive, transduce, and respond Lecture 5: Energy, Reactions, ATP Energy in chemicals is used to drive biological processes and most are directly or indirectly driven by ATP Energy and matter are interconvertible ○ Matter is “condensed” energy Laws of Thermodynamics ○ 1st Law Energy in the universe is constant in that it can neither be created or destroyed ○ 2nd Law Disorder of the universe always increases reaction/processes occur spontaneously only when the disorder of the universe increases ○ 3rd Law As temperature approaches 0 kelvin; disorder approaches zero Open system ○ Energy and matter are exchanged with surroundings (living organisms) Closed system ○ Only energy is exchanged with surroundings State function ○ Depends only upon the initial state and final state of the process, is independent of the pathway e.g. energy Non-state function ○ Depends upon the pathway of the process e.g. heat (q) and work (w) Delta E = q (heat) the w (work) Enthalpy (H) ○ State function ○ Measure of the system’s internal energy i.e heat content ○ H = E + PV where PV is work done by changes in pressure and volume ○ Delta H < 0 Heat is given off - exothermic ○ Delta H > 0 Heat is absorbed - endothermic ○ Delta H = 0 Reaction is isothermic Entropy (S) ○ Degree of disorder - state function ○ 2nd law - entropy is positive for all spontaneous reactions (entropy in the universe) ○ In living organisms, disorder does not increase in the cells, it increases in the surroundings ○ Delta S (universe) = Delta S (surrounding) + Delta S (system) ○ Gibbs Free Energy: Delta G = Delta H - T Delta S (system) ○ Delta G < 0 - spontaneous reaction (exergonic) ○ Delta G > 0 - non-spontaneous reaction (endergonic) ○ Delta G = 0 - process is at equilibrium ○ Delta G tells you if a reaction occurs spontaneously but tells you nothing about the rate of the reaction ○ Standard Free Energy: Delta G = Delta G prime + RTln(X) Reaction Rate = k(A)(B) ○ Depends upon reactants ○ Some reactions are fast and slow and this is reflected in the rate constant K ○ Forward reaction rate = K1 (A)(B) ○ Reverse reaction rate = K-1 (C)(D) ○ Keq = (C)(D)/(A)(B) = K1/K-1 Coupled Reactions ○ Lecture 6: Prokaryotes, Eukaryotes, and Biological Membranes Cells are the basic unit of life Prokaryotes ○ Lack a nucleus, single celled Two classes: bacteria and archaea (extremophiles) Eukaryotes ○ Have a nucleus ○ Can be multicellular Biological Membranes ○ Thin, flexible sheet like structures surrounding cells and organelles Hydrophilic and hydrophobic components are important for organization ○ Provides a selective physical barrier ○ Act as barriers Semipermeable and only allow some molecules into the cell Hydrophobic chains of lipid bilayer are the impermeable barrier to ion and polar molecules Lipid bilayer ○ Organized through non-covalent interactions Membrane proteins ○ Integral Embedded in the membrane ○ Peripheral Attached to the surface of the membrane ○ ○ Simple diffusion Non ionic and some small molecules diffuse across the membranes (simple diffusion) ○ Facilitated diffusion Larger molecules and ions can diffuse through channels ○ Active transport Requires energy and is often against concentration gradient ○ Primary active transport Energy for transport comes from ATP ○ Secondary active transport Electrochemical gradient is the driving force Lecture 7: Amino Acids Amino acids are an organic compound that contains both an amino (R-NH2) and carboxyl (R-COOH) functional groups ○ Other functional groups may be present as well The alpha carbon is connected to the carboxyl group In the alpha amino acid, the amino group is connected to the alpha carbon and the beta amino acid to the beta carbon Two isomers that are mirror images of each other called enantiomers ○ Such isomers have identical chemical properties, but rotate polarized light in different directions right (D) or left (L) Only L-amino acids can be used by the living cells for protein biosynthesis Titration Curve ○ An amino acid can carry both positive and negative charges which makes it a zwitterion ○ Amino group is a base ○ Carboxyl group is an acid ○ Isoelectric point ○ The pH at which an amino acid has zero net charge ○ pI = pKa (-COOH) + pka (-NH3+)/2 Lecture 8: Proteins and Peptides Linear polymers of standard amino acids are called proteins ○ Polymerization involves formation of amide linkages between primary carboxyl and amino groups of two amino acids ○ Unshared electron pair of the alpha amino nitrogen atom attacks the alpha carboxyl carbon in a nucleophilic acyl substitution reaction ○ The linked amino acids are referred to as amino acid residues because water has been removed (dehydration reaction) ○ The alpha amino group of incoming amino acid reacts with the open alpha carboxyl of the growing chain ○ The open alpha amino and alpha carboxyl groups of a polypeptide are referred to as the N and C terminus respectively ○ Peptide bonds are rigid and planar due to a partial double bond character ○ No rotation is allowed along the C-N bond Fibrous and globular proteins ○ Fibrous proteins are long, rod-shaped molecules that are insoluble in water and physically tough Many structural proteins are fibrous proteins Keratin, silk fibroin, and collagen are fibrous proteins ○ Collagen Structural proteins found in skin, bones, tendons, blood vessels, and corneas Collagen fibers appear to have a banding pattern Hole zones are caused by gaps in packing of collagen molecules ○ Collagen Alpha chains Collagen alpha chains form a unique left handed helix and is different from the alpha helical protein fold Three left handed alpha chains are twisted together into a right handed coil-coil; a triple helix stabilized by numerous hydrogen bonds between separate chains To be able to form a triple helix collagen has unique amino acid sequence with frequent Gly-Pro-Pro repeats Globular Proteins ○ Compact spherical molecules that are usually water soluble ○ Soluble because their most polar side chains face the outside of the protein and interact with water while hydrophobic side chains are buried ○ Exemplified by myoglobin and hemoglobin involved in oxygen transport and storage Myoglobin ○ Responsible for the oxygen storage in mammalian muscle tissue ○ O2 binds to the prosthetic group heme ○ Directs O2 binding by heme Hemoglobin ○ Responsible for the oxygen transfer from the lungs to every tissue in the body ○ HbA molecule is a tetramer commonly designated A2B2 ○ Both alpha and beta subunits are homologous to myoglobin and contains a single heme Myoglobin vs. Hemoglobin ○ Myoglobin has normal saturation behavior and hyperbolic oxygen binding curve ○ Hemoglobin shows cooperative behavior and sigmoidal oxygen binding curve ○ As a result of the cooperativity oxygen dissociates from hemoglobin under venous pressure ○ Myoglobin won’t be able to accommodate this function The Bohr Effect ○ O2 binding by hemoglobin is pH dependent Glutathione ○ Contains an unusual gamma amide bond ○ Found in almost all organisms ○ Plays roles in protein and DNA synthesis, drug and environmental toxin metabolism, amino acid transport ○ Protects cells from the destructive effect of oxidation by the reactive oxygen species Lecture 9: Protein Structure Protein Primary Structure ○ Unique sequence of amino acid residues Encoded in the gene and inherited Hold by the peptide bond, a strong covalent interaction which would survive extreme temperatures, ionic strength and radiation Determines all higher levels of protein structure ○ Linear polymers of 20 standard amino acids connected by the primary amino and carboxyl groups ○ Starts with N terminal residue and ends with C terminal residue ○ Assembled from aminoacyl-RNAs by the ribosome according to the mRNA template Protein Folding ○ Linear polypeptides promptly assume compact shapes called native states ○ Transition of linear (unfolded) polypeptide into the native state is called folding ○ Correct folding is a prerequisite for the normal activity and function ○ Folding gives rise to 3 additional levels of protein structure ○ Protein folding is a spontaneous process driven by small negative changes in free energy Growing protein backbone interacts with water (cytosolic protein) or the lipid bilayer (integral membrane protein) Loss of native state is termed denaturation which is usually reversible Protein denaturation could be caused by a temperature spike (heat shock), shift in pH, exposure to organic solvents, salts, heavy metal ions Anfinsen’s Experiment ○ Ribonuclease A (RNase A) is a protein made of 124 amino acids including 8 cysteine forming 4 disulfide (S-S) bridges 8M urea unfolds RNase A while not breaking any covalent bond Beta-Mercaptoethanol reduces cysteines which “opens” S-S bridges ○ Using restoration of RNase A activity as a readout it was found that if urea was gradually removed first and then RNase A was oxidized by atmospheric O2, the correct S-S bridges appeared and almost 100% of activity was regained ○ However, if RNase A was oxidized first, gradual removal of urea resulted in restoration of only 1% of activity ○ Restoration of activity was hindered by incorrect S-S bridges leading to appearance of “scrambled” structure ○ ○ Protein folding cannot work as a random search process Each protein residue can assume only 3 different configurations Spontaneous process (delta G < 0) that occurs efficiently and fast in the living cells Protein folding can be facilitated by specialized proteins called molecular chaperones Non native states are actively recycled ○ Molecular Chaperones Heat shock causes massive protein misfolding In response cells induce expression of genes to deal with non-native proteins Many chaperones were discovered in the heat shock studies Secondary Structure ○ Hydrogen bonds stabilize secondary structure ○ Two types of secondary structures are the alpha helix and beta pleated sheet ○ Regions with the secondary structure are connected by unstructured regions Alpha Helix Rigid rod-like right handed helix Maintained by hydrogen bonds 3.6 amino acid residues per turn Side chains of all amino acid within the alpha helix extend outward and determine properties of its surface Beta pleated sheet Hydrogen bonds are formed between different segments of the polypeptide called beta strands Can be parallel and antiparallel Pelated shape because adjacent peptide bonds can’t be rotated freely Tertiary Structure ○ Is a native state i.e unique three dimensional shape predominantly present in the living cells Protein folding leads to acquisition of tertiary structure Core free dimensional structure of a domain called a fold Interactions that maintain tertiary structure Hydration Hydrophobic interactions Hydrogen bond Water bridges Salt bridges Disulfide bridges Quaternary structure ○ Many proteins are composed of multiple polypeptide chains Each polypeptide chain within a protein is called a subunit Subunits are held together by non-covalent interaction including electrostatic interactions, hydrophobic interactions, and hydrogen bonds In some rare cases covalent interaction, such as disulfide bridges, can also maintain quaternary structure Protein can be classified as simple or conjugated ○ Simple proteins contain only amino acids ○ Conjugated protein consists of a simple protein covalently bound to a non-protein component called a prosthetic group ○ Some proteins need a non-protein component which is NOT covalently bound to them for their functions; such component is called a coenzyme Coenzymes ○ CoA and NAD+ are examples of coenzymes that carry chemicals groups between enzymes ○ Usually both are not covalently bound to the protein ○ Coenzyme A participates in acyl-group transfer enzymatic reaction ○ NAD+ carries e- released in biological oxidation reactions Lecture 10: Properties of Enzymes Rate of Chemical Reaction ○ Can be expressed in terms of the rate constant V= k(A) ○ The rates of two reactions going in opposite directions are expressed as V= K1(A) and V=K-1(B) ○ The rate of a higher order reaction is expressed as V=k(A)(B) ○ If the reaction is reversible the rates are expressed as V= K1(A)(B) and V= K-1(C) Units of the Rate Constant K ○ Units of K depend on which order kinetics the reaction is following For a zero order reaction: mole x L-1 x s-1 For the first order reaction: s-1 For the second order reaction: mole -1 x L x s-1 ○ A reaction has attained equilibrium when delta A/delta t = delta B/delta t = delta C/delta t = 0 ○ At equilibrium V1=V-1 thus K1(A)(B) = K-1(C) ○ Note that equilibrium does not require that K1=K-1 or (A)(B) = (C) Thermodynamics of equilibrium ○ The Gibbs energy change delta G predicts whether or not reaction occurs, e.g reaction A to B can proceed as written only if its delta G < 0 ○ For a reversible reactions A to B, the G is related to the mass action constant Keq as Delta G = Delta G prime + RTln Keq Understanding the standard free energy change Delta G prime ○ When a reaction A to B has attained equilibrium, its free Gibbs energy change delta G = 0 At this state the standard energy change of the reaction is related to the equilibrium constant Therefore Delta G prime predicts (A) and (B) at equilibrium Delta G prime predicts (A) and (B) at equilibrium ○ Conversion of A to B is possible with negative free Gibbs energy change; if the reaction is reversible, at some point B will start converting back to A ○ Catalysts reduces the activation energy of the reaction ○ A catalyst alters the free energy of activation but not the standard free energy of the reaction ○ The transition state occurs at the apex of both reaction pathways What happens when activation energy is reduced ○ A reduction of activation energy leads to increase of the rate constant K, and of the reaction rate v ○ Both reverse and forward reactions are affected to exactly the same degree ○ As a result the equilibrium is achieved faster with NO change of the equilibrium constant Difference between Gibbs free energy and Activation energy ○ The activation energy designates how much energy is needed to achieve the transition state ○ Activation energy is related to the rate constant K, as it determines the rate at which the reaction proceeds at a given T ○ A negative free gibbs energy change of a reaction predicts that it can be spontaneous however a high activation energy can prevent it from happening Properties of Enzymes ○ Enzymes are protein catalysts utilized in most of the chemical reactions within the cell ○ Enzymes speed up reactions but are not permanently altered or consumed during this process ○ In contrast to a general catalyst, an enzyme is usually suited for only one type of chemical reaction Lecture 11: Enzyme Kinetics How to measure enzyme kinetics ○ A simple enzyme catalyzed reaction in which substrate (S) is consumed and product (P) is generated in the presence of enzyme (E) ○ To study kinetics one would need to have Solution of S of known concentration Pure E of known concentration Assay to measure -delta(S)/delta t or delta(P)/delta t Rate is determined as a function of (S) Michaelis-Menten constant, Km ○ Km is concentration of substrate (S) at which V0= Vmax/2 ○ Km units are mole L -1 Vmax ○ Vmax = K2(Eo) ○ Kcat ○ K2 is termed Kcat or turnover constant ○ Kcat units are s-1 ○ Kcat = Vmax/(Eo) Specificity Constant ○ Kcat/Km Lecture 12: Enzyme Inhibition Molecules that reduce enzymes’ activity are called enzyme inhibitors Inhibitors could be classified as reversible or irreversible ○ The effect of reversible inhibitors could be turned off by either adding increasing amounts of substrate or removing inhibitor from the media, while the enzymes remains intact ○ Irreversible inhibitors bind to enzymes by covalent associations resulting in their terminal inactivation Kinetic analysis of reversible inhibitors ○ Reversible inhibitors could be divided into two types Competitive and noncompetitive inhibitors Each type is characterized by the specific mechanism of inhibition which could be recognized from the kinetic analysis Competitive inhibition ○ Inhibitor (I) binds to the substrate binding site ○ Competitive inhibitors are often substrate analogs ○ EI + S = no reaction ○ Competitive inhibitor increases the Km, so it appears as if enzyme’s affinity for the substrate decreases ○ Has no effect on the Vmax because while concentration of the inhibitor in the assay remains constant, it will be eventually outcompeted by increasing (S) Non-competitive inhibition ○ Inhibitor (I) binds to a site distant from the substrate binding site, thus inhibitor and substrate can bind simultaneously ○ Inhibitor doesn’t structurally resemble substrate ○ Reduces the Vmax ○ No effect on Km ○ Two types of non-competitive inhibition Pure and mixed Pure inhibition if Ki1 =Ki2 Mixed inhibition if Ki1 doesn’t equal Ki2 Uncompetitive inhibition ○ A case when the inhibitor only binds ES ○ Ki1=0