Biochemistry Lecture Midterms PDF
Document Details
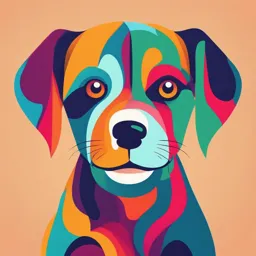
Uploaded by SensationalQuasimodo
Kimberly Shane Villa
Tags
Related
- Bioquímica Aula 14 - 22.23 PDF
- BIOL111 Lectures 5 and 6 2024 PDF
- Metabolism Lecture Notes PDF
- 5415 Metabolism (2024-2025, 1st Semester) PDF
- Lecture 3: Glycolysis, Krebs Cycle, PDH Complex, and ETS Pathways PDF
- SIJ1003 Glycolysis, Citric Acid Cycle, electron transport and oxidative phosphorylation PDF
Summary
This document is a biochemistry lecture midterm covering the overview of biochemical energy production. It details the stages of energy production, discusses the digestion process, and describes the principal compounds involved in the process including ATP and related functions. The document also touches on energy storage, carriers, and cofactors.
Full Transcript
BIOCHEMISTRY LECTURE MIDTERMS OVERVIEW OF THE BIOCHEMICAL ENERGY PRODUCTION Energy needed to run the human body is obtained from ingested through a multistep process that involves several different catabolic pathways. There are several 4 general stag...
BIOCHEMISTRY LECTURE MIDTERMS OVERVIEW OF THE BIOCHEMICAL ENERGY PRODUCTION Energy needed to run the human body is obtained from ingested through a multistep process that involves several different catabolic pathways. There are several 4 general stages in biochemical energy production Energy needed to run the human body is obtained from ingested through a multistep process that involves several different catabolic pathways. The diagram shows the breakdown There are several 4 general stages in of glucose, amino acids, and fatty biochemical energy production acids into Acetyl-CoA, which then →Stage 1: Digestion enters the citric acid cycle. → Stage 2: Acetyl group formation The citric acid cycle produces → Stage 3: Citric acid Cycle electron carriers (NADH and → Stage 4: Electron transport chain and FADH2) that are used in the electron oxidative phosphorylation transport chain, which generates STAGE 1: DIGESTION ATP, the primary energy currency of Begins in mouth (saliva contains cells. starch digesting enzymes), continues The diagram highlights the three in the stomach (gastric juice), main stages of cellular respiration: completed in small intestine (the glycolysis, the citric acid cycle, and majority of digestive enzymes and oxidative phosphorylation. It also bile salts). shows the production of CO2 and Results in small molecules that can H20 as byproducts of the process. cross intestinal membrane into the Principal Compounds participating in the blood common catabolic pathway End Products of digestion: A. Energy storage and Carrier → Glucose and monosaccharides from B. Cofactors carbohydrates Agent for electron transfer → Amino acids from proteins Agent for Acetyl groups → Fatty acids and glycerol from fats and Vitamins oils - Adenosine Triphosphate (ATP) : The digestion products are absorbed The Energy Currency of Life into the blood and transported to Fundamental molecule in all living body's cells. organisms, serving as the primary Kimberly Shane Villa 1 BIOCHEMISTRY LECTURE MIDTERMS energy carrier for cellular NAD processes. Nicotinamide Adenine PROPERTIES OF ATP Dinucleotide ATP is produced from adenosine Derived from vitamin NIACIN diphosphate (ADP) and inorganic Reduced by accepting two electron phosphate (mainly by the process of and a proton to become NADH NH2 oxidative phosphorylation. FAD The free energy released when Flavin Adenine ATP is hydrolyzed is used to drive Dinucleotide reactions that require energy. Derived from vitamin ATP can transfer phosphate groups RIBOFLAVIN to other compounds such as glucose, Reduced by accepting two electrons forming ADP. and two proton to become FADH2 ADP can accept phosphate groups FMN from compounds such as Receives electrons from NADH in phosphocreatine, forming ATP. complex I and transfers them FUNCTIONS OF ATP through Fe-S centers to CoQ. ATP is constantly being consumed FMN is derived from riboflavin. and regenerated. Coenzyme Q The free energy released when ATP receives electrons from FMN and is hydrolyzed Is used to drive also through Fe-S centers from reactions that require energy. FADH2 ATP can transfer phosphate groups CoQ can be synthesized in the body. to compounds such as glucose, It is not derived from a vitamin. forming ADP. NADPH ATP is a precursor for provides reducing equivalents for the deoxyribonucleotide triphosphates synthesis of fatty acids and other (dNTPs), the building blocks of compounds and for the reduction of DNA, and for ribonucleotide glutathione. triphosphates (NTPs), the building NADP blocks of RNA. is identical to NAD+ except that it ATP plays a role in intracellular contains an additional phosphate signaling pathways, acting as a group. substrate for kinases, which Lipoic Acid phosphorylate proteins to regulate A cofactor that acts as an electron their activity. carrier in the pyruvate Certain cofactors or enzymes are involved In dehydrogenase and a-ketoglutarate the transfer of electrons from macronutrients dehydrogenase complexes. This to a process that generates energy for the means it helps transfer electrons production of ATP. Kimberly Shane Villa 2 BIOCHEMISTRY LECTURE MIDTERMS during these important Vitamin C energy-producing reactions. It is involved in hydroxylation Coenzyme A reactions, such as the hydroxylation acting as a carrier of acyl groups, of proly! residues in the precursor of which are essentially carbon chains collagen. with a carbonyl group (C=0) at one It functions in the absorption of iron. end. It is an antioxidant CoA is essential for the breakdown Vitamin K of fatty acids. It attaches to the fatty Involved in the activation of acid, forming an acyl-CoA precursors of prothrombin and other derivative, which then enters the clotting factors by carboxylation of mitochondria for B-oxidation. glutamate residues. Vitamin B1/ Thiamine B. OTHER COFACTORS Thiamine is a crucial component of Biotin thiamine pyrophosphate (TPP), a Involved in the carboxylation of coenzyme involved in the conversion pyruvate (which forms oxaloacetate of pyruvate to acetyl-CoA, the ), acetyl-CoA (which forms starting molecule for the citric acid malonyl-CoA), and propionyl-CoA cycle. (which forms methyl malonyl-CoA). Vitamin B3/ Pantothenic Acid Thiamin pyrophosphate Pantothenic acid is a precursor to Involved in the decarboxylation of coenzyme A (CoA), which is a-keto acids. essential for the formation of also the cofactor for the enzyme acetyl-CoA and for the function of transketolase of the pentose many enzymes in the citric acid phosphate pathway. cycle. formed from ATP and the vitamin Vitamin B5/Niacin thiamin. Niacin is a component of NAD Pyridoxal phosphate (nicotinamide adenine dinucleotide), the active form of vitamin B6 a crucial electron carrier in the citric (pyridoxine), is a versatile coenzyme acid cycle. involved in over 140 different Vitamin B12 enzymatic reactions, primarily in which contains cobalt, is involved in amino acid metabolism. an aldehyde, two reactions in the body: Interacts with an amino acid to form It transfers methyl groups from a Schiff base. tetrahydrofolate to homocysteine (to Tetrahydrofolate form methionine). transfers 1-carbon units from It is Involved In the conversion of compounds such as serine to methyl malonyl-CoA to compounds such as deoxyuridine succinyl-CoA. Kimberly Shane Villa 3 BIOCHEMISTRY LECTURE MIDTERMS monophosphate to form - Anaerobic Conditions: In the deoxythymidine monophosphate absence of oxygen, pyruvate is Tetrahydrofolate is synthesized from converted to either lactate (in the vitamin folate. animals) or ethanol (in some GLYCOLYSIS organisms). Also known as the Embden-Meyerhof-Parnas pathway. The first stage of cellular respiration, a metabolic pathway that breaks down glucose (a six-carbon sugar) into pyruvate (a three-carbon molecule). Glycolysis occurs in the cytoplasm of all living cells. Glycolysis can occur in the presence Glucose is a highly polar molecule or absence of oxygen (anaerobic). and cannot enter cells by passive Liver is the primary site of diffusion across the membrane. glycolysis. Transporter proteins called GLUTs Glycolysis occurs in the cytosol and (GLUcose Transporters), catalyze consists of 10 different steps glucose import into cells. ✔️ - Steps 1 to 5 Glucose transporters are highly ✔️ Energy investment phase specific for glucose, binding to it ATP is consumed and facilitating its transport. ✔️ - Steps 6 to 10 Insulin, a hormone crucial for ✔️ Energy generating phase glucose regulation, normally ATP is produced stimulates glucose uptake into cells, Pentose Phosphate Pathway: A separate particularly in skeletal muscle and pathway that produces NADPH and adipose tissue, via GLUT essential precursor molecules for transporters. biosynthesis. Pyruvate's Fate: Pyruvate's fate depends on the presence or absence of oxygen: - Aerobic Conditions: Under oxygen-rich conditions, pyruvate is converted to acetyl-CoA, which enters the TCA cycle (also known as the Krebs cycle) for further energy production. Kimberly Shane Villa 4 BIOCHEMISTRY LECTURE MIDTERMS STEP 1: PHOSPHORYLATION OF GLUCOSE Glucose enters cells via transporters, phosphorylation rapidly traps it inside. The ATP-dependent phosphorylation STEP 4: The six-carbon fructose is split of glucose to give glucose-6- into two 3-carbon units phosphate is catalyzed by Fructose-1,6-bisphosphate binds to hexokinase. This reaction is the enzyme "aldolase". irreversible under intracellular The six-carbon fructose is split into conditions. two 3-carbon units, dihydroxyacetone phosphate (DHAP) and glyceraldehyde-3-phosphate (G3P) STEP 2: PHOSPHOHEXOSE ISOMERIZATION The phosphorylated glucose, an aldose sugar, is now isomerized to phosphorylated fructose, a ketose sugar, in a reaction catalyzed by STEP 5: Interconversion of the triose phosphohexose isomerase. phosphates DHAP is immediately isomerized to G3P, and glycolysis continues from G3P only. The enzyme triose phosphate isomerase catalyzes the conversion of DHAP into G3P STEP 3: Phosphorylation of fructose-6-phosphate The conversion of fructose-6-phosphate to fructose- 1,6-bisphosphate by phosphofructokinase. The reaction is irreversible under STEP 6: Oxidation of G3P to produce a normal cellular conditions, ensuring high-energy bond and NADH. that the pathway proceeds in the This reaction is the energy key to direction of glucose breakdown. glycolysis. Kimberly Shane Villa 5 BIOCHEMISTRY LECTURE MIDTERMS In the enzyme-catalyzed process, intermediate G3P is bound to a cysteine residue 2,3-bis-phospho-glycerate on the enzyme G3P dehydrogenase and oxidized to an enzyme-bound thioester. The thioester is then attacked by inorganic phosphate, yielding the product 1,3-bis-phosphoglycerate STEP 9: Dehydration of 2-phosphoglycerate The enzyme, enolase - eliminates water to create a double bond. The systematic name for this enzyme STEP 7: Phosphoryl transfer from is phosphopyruvate hydratase; it is 1,3-BPG to ADP: the first substrate level named on the basis of the reverse phosphorylation reaction, the hydration of The free energy of hydrolysis of the phospho-enol-pyruvate (PEP) anhydride bond is now recovered in the form of ATP, in a reaction catalyzed by phosphoglycerate kinase Two moles of 1,3-bisphosphoglycerate per mole of STEP 10: Transfer of the phosphoryl glucose, this reaction generates two group from PEP to ADP ATP per glucose. The last enzyme is pyruvate kinase. The free energy liberated by PEP hydrolysis is sufficient to drive ATP generation at this step. STEP 8: Conversion of 3-phosphoglycerate to 2-phosphoglycerate mutases catalyze reactions in which a functional group (phosphate) is shunted between different positions in a molecule. The enzyme, phosphoglycerate mutase accomplishes this reaction by transferring a phosphate group from a side chain of the enzyme to the "vacant" -OH group of either 2- or 3-phosphoglycerate, producing the Kimberly Shane Villa 6 BIOCHEMISTRY LECTURE MIDTERMS PYRUVATE DEHYDROGENASE Citrate Is Kreb's Starting Substrate For COMPLEX (PDH) Making Oxaloacetate This enzyme occupies a key position Krebs cycle in metabolism: it is the link between Also known as the citric acid cycle glycolysis and the citric acid cycle. or tricarboxylic acid It converts pyruvate (the end product The Krebs cycle takes place in the of glycolysis) into acetyl CoA, the mitochondrial matrix, the first metabolite of the citric acid innermost compartment of cycle, condensing with OAA to mitochondria. make citrate. The Krebs cycle involves a series of PDH occurs in the mitochondrial eight enzymatic reactions that matrix and catalyzes an irreversible break down acetyl-CoA, releasing oxidative decarboxylation. high-energy electrons and carbon Pyruvate dehydrogenase complex dioxide as a byproduct. consists of three different enzymes It acts as a central hub for the and utilizes 5 different cofactors; breakdown of carbohydrates, lipids, four of which are derived from B and proteins. The products of their vitamins. initial breakdown (pyruvate, acetyl-CoA, and certain amino acids) all converge on the Krebs cycle. All the enzymes of the TCA cycle are in the mitochondrial matrix except succinate dehydrogenase, which is in the inner mitochondrial KREBS CYCLE membrane. The Krebs cycle is tightly regulated by various enzymes and feedback mechanisms to ensure efficient energy production and respond to the cell's energy needs. The Krebs cycle is a cyclic process, meaning that the starting molecule, oxaloacetate, is regenerated at the end of each cycle. ✔️ Products: The Krebs cycle produces: NADH and FADH2: These molecules are electron carriers that will be used in the ✔️ electron transport chain to generate ATP. ATP: The Krebs cycle directly produces one molecule of ATP per turn. Kimberly Shane Villa 7 BIOCHEMISTRY LECTURE MIDTERMS ✔️Carbon Dioxide: Carbon dioxide is STEP 3: Oxidative decarboxylation of released as a waste product. isocitrate to alpha-ketoglutarate and COz. We will follow the two carbons of acetyl The isocitrate undergoes oxidation group through each step in the Krebs cycle: and decarboxylation at the same STEP 1: Condensation of oxaloacetate with time. acetyl-CoA, producing citrate. This reaction involves the oxidation Acetyl coenzyme A combines with of the alcohol group of isocitrate to a oxaloacetate to form citrate. v The ketone through the transfer of a process is catalyzed by the enzyme hydride from the C-H of the alcohol citrate synthase and involves the to NAD+ followed by the loss of the addition of the acetyl group to the -COO group as CO2 (oxidative carbonyl group of oxaloacetate. decarboxylation). The reaction also results in the The oxidizing agent is NAD+, which release of coenzyme A. is reduced to NADH in the process. This step is considered a building-up This step is important because it process, as it adds carbons to the generates NADH, an electron carrier cycle, rather than breaking them that will be used in the electron down. transport chain to produce ATP. Enzyme: Citrate synthase Enzyme: Isocitrate dehydrogenase STEP 4: Oxidative decarboxylation of STEP 2: Isomerization of isocitrate from alpha-ketoglutarate to succinyl-CoA and citrate. COz Citrate is isomerized to isocitrate by a-Ketoglutarate is converted to a rearrangement of the molecule. succinyl-CoA in an oxidative i. Enzyme: aconitase decarboxylation reaction, ii. Aconitate serves as an enzyme-bound mechanistically the same as the intermediate. pyruvate dehydrogenase reaction. iii. Under physiologic conditions, this is an CO2 is released, and succinyl-CoA, unfavorable reaction, favoring citrate NADH, and H+ are produced. formation. This step captures another two electrons from the carbons of acetyl-CoA. Enzyme: a-ketoglutarate dehydrogenase. Kimberly Shane Villa 8 BIOCHEMISTRY LECTURE MIDTERMS This enzyme requires five cofactors: This enzyme is present in the inner thiamin pyrophosphate, lipoic acid, mitochondrial membrane. The other CoASH, FAD, and NAD+ enzymes of the cycle are in the matrix. STEP 5: Conversion of succinyl-CoA to succinate STEP 7: Hydration of fumarate to malate. Succinyl-CoA is cleaved to Fumarate is converted to malate by succinate. the addition of water across the Cleavage of the high-energy double bond. thioester bond of succinyl-CoA Enzyme: fumarase provides energy for the substrate-level phosphorylation of GDP to GTP. Because this does not involve the electron transport chain, it is not an oxidative STEP 8: Oxidation of malate to form phosphorylation; however, if oxaloacetate. electron flow were to stop, this step Malate is oxidized, regenerating would also be blocked. Enzyme: oxaloacetate and thus completing the succinate thiokinase or cycle. succinyl-CoA synthetase. Two hydrogens along with their electrons are passed to NAD+, producing NADH and H+ Finishing the capture of the eight electrons from the carbons of acetyl-CoA. STEP 6: The oxidation of succinate to Enzyme: malate dehydrogenase. fumarate Succinate is oxidized to fumarate. Succinate transfers two hydrogens together with their electrons to FAD, forming FADH2 After this step, six of the eight Energy production by the TCA cycle electrons from the carbons in Krebs Cycle Output: One turn of the acetyl-CoA have been captured Krebs cycle produces: Enzyme: succinate dehydrogenase. - 3 moles of NADH - 1 mole of FADH2 Kimberly Shane Villa 9 BIOCHEMISTRY LECTURE MIDTERMS - 1 mole of GTP (which can be mitochondrial membrane to produce converted to ATP) ATP ✔️ ATP Yield: The electron transport ADP level: HIGH ✔️ chain is highly efficient, yielding The cells need energy approximately 2.5 moles of ATP for Reactions of electron transport chain ✔️ each mole of NADH and 1.5 moles is accelerated ✔️ of ATP for each mole of FADH2. NADH is rapidly oxidized Total ATP: Therefore, the total ATP ADP allosterically activates yield from one turn of the Krebs isocitrate dehydrogenate. cycle is approximately 10 moles of Concentration of ATP: HIGH ATP (3 NADH x 2.5 ATP/NADH + - Cells have adequate energy supply, 1 FADH2 x 1.5 ATP/FADH2 + 1 Electron transport slows down, GTP). NADH builds up, TCA cycle is FATE of NADH, FADH2 and GTP inhibited. The NADH and FADH2 molecules - This excerpt describes how high from the Krebs cycle enter the levels of NADH inhibit the TCA electron transport chain, where they cycle, leading to its slowdown. It donate electrons. This electron flow highlights two main mechanisms: drives the production of ATP through 1. Direct Inhibition of Isocitrate oxidative phosphorylation. Dehydrogenase: High NADH The NADH and FADH2 molecules allosterically inhibits isocitrate from the Krebs cycle enter the dehydrogenase, a key enzyme in the electron transport chain, where they TCA cycle. This leads to the donate electrons. This electron flow accumulation of isocitrate, which drives the production of ATP through then favors the formation of citrate. oxidative phosphorylation. Citrate further inhibits citrate The GTP produced directly in the synthase, the first enzyme of the Krebs cycle is quickly converted to cycle, further slowing down the ATP by the enzyme nucleoside process. diphosphate kinase, making the 2. Mass Action Effect: High NADH energy stored in GTP available for levels also influence the reactions cellular processes. that generate NADH, leading to a REGULATION OF THE KREBS CYCLE slowdown of the cycle due to the The Krebs cycle is regulated by the principle of mass action. This means Cell's need for energy in the form of that the high concentration of NADH ATP. pushes the equilibrium of these The Krebs cycle acts in concert with reactions towards the reactants, the electron transport chain and the slowing down the production of ATP synthase in the inner NADH and further inhibiting the TCA cycle. Kimberly Shane Villa 10 BIOCHEMISTRY LECTURE MIDTERMS Oxidative Phosphorylation: The Electron THE ELECTRON CARRIERS OF THE Transport Chain ELECTRON TRANSPORT CHAIN: Oxidative phosphorylation is the COENZYME Q (UBIQUINONE) final stage of cellular respiration, Coenzyme Q, also known as Oxidative phosphorylation takes ubiquinone, contains a quinone place in the mitochondria, group. specifically in the inner This group acts as an oxidizing mitochondrial membrane. agent, accepting electrons and Reduced cofactors such as NADH becoming reduced to ubiquinol and FADH2 are good reducing (QH2). agents. Oxygen is a very good This reduction process involves two oxidizing agent - one of the strongest electrons and two protons, and it known to chemistry. happens in two steps, with a free Three types of electron transfers radical intermediate called occur in the electron transport chain; semiquinone. 1. Direct transfer as in the reduction of It can freely move in the membrane Fe3+ to Fe2+ carrying electrons from one ETC 2. 2. Transfer of a hydrogen atom (H+ complex to another. + e-) 3. 3. Transfer of a hydride ion (:H-) bearing two electrons MITOCHONDRIAL ELECTRON TRANSPORT CHAIN In the electron transport chain, electrons from NADH and FADH2 are passed along a series of protein complexes. NADH starts at a higher energy level and fuels the chain earlier, while CYTOCHROME C FADH2 enters later due to its lower Cytochromes are a large class of energy level. heme proteins participating in redox Each complex acts like a big reactions. enzyme, facilitating the transfer of The heme group of cytochrome c is electrons. Ultimately, these electrons covalently linked to the protein via are passed to oxygen, the final cysteine residues electron acceptor. Cytochrome c is a soluble protein in the mitochondrial intermembrane space and shuttles electrons from complex Ill of the ETC to complex IV. The iron atom of heme acts as the Kimberly Shane Villa 11 BIOCHEMISTRY LECTURE MIDTERMS redox active component and carries one electron at a time. COMPLEXES (1,11, III, IV) The complexes are enzymes that catalyze individual electron-transfer steps in the chain. The complexes are integral membrane proteins; they are buried COMPLEX IV (CYTOCHROME deeply in the inner mitochondrial OXIDASE): membrane. Catalyzes the transfer of electrons COMPLEX I (NADH from reduced Cyt c to molecular 02, DEHYDROGENASE): the final electron acceptor. Complex I catalyzes two processes The energy released by the transfer which are obligately coupled to each of each 2 electrons is used to pump 2 other protons into the intermembrane Transfer of a hydride ion from space. NADH and a H+ from the matrix to coenzyme Q Transfer of four H+ from the matrix to the intermembrane space ATP Synthase and Oxidative COMPLEX II (SUCCINATE Phosphorylation: A Vital Partnership DEHYDROGENASE): As electrons move through the ETC, Complex Il is the TCA cycle enzyme they lose energy, which is used to succinate dehydrogenase. It transfers pump protons (H+) from the electrons from FADH, generated by mitochondrial matrix across the inner this reaction to coenzyme Q. membrane into the intermembrane It does NOT pump protons from the space. matrix to the intermembrane space. The pumping action in the mitochondria creates a proton gradient across the inner membrane, resulting in a higher concentration of protons in the intermembrane space and a lower concentration in the COMPLEX III (CYTOCHROME C mitochondrial matrix. REDUCTASE): ATP synthase is a molecular machine Complex II transfers electrons from in the inner mitochondrial membrane QH, to cytochrome c with the that connects the proton gradient to simultaneous transfer of four ATP production. It has two main protons from the matrix to the components: intermembrane space. Kimberly Shane Villa 12 BIOCHEMISTRY LECTURE MIDTERMS 1. FO component: Embedded in the membrane, it acts as a proton channel, enabling protons to move back into the mitochondrial matrix from the intermembrane space. 2. F1 component: Extending into the mitochondrial matrix, it houses the catalytic sites responsible for ATP synthesis. When protons move through the Fo component, they induce the rotation of a central stalk in the complex. This rotation leads to structural changes in the F1 component, enabling the binding of ADP and inorganic phosphate (Pi), their conversion into ATP, and the release of the newly formed ATP molecule. Inhibitors of Electron Transport Chain Inhibitors of the ETC interfere with this process, disrupting energy production and potentially causing cell damage. How ETC Inhibitors Work: Blocking Electron Flow: These inhibitors target specific components of the ETC, preventing the flow of OXYGEN TOXICITY AND electrons from one complex to the FREE-RADICAL INJURY next. This disrupts the chain Formation of Reactive Oxygen Species reaction, halting the pumping of (ROS): Oxygen can accept single electrons protons across the mitochondrial and form highly reactive oxygen radicals membrane. like superoxide (02), hydrogen peroxide Disrupting Proton Gradient: (H202), and the hydroxyl radical (HO.). Without the proper flow of electrons, ROS Generation: ROS can be produced in the proton gradient, which is a controlled (enzymatic) or uncontrolled essential for ATP synthesis, cannot (nonenzymatic) manner, contributing to be established. This means ATP oxidative stress and cell damage. synthase, the enzyme responsible for Cellular Damage: Oxygen radicals, ATP production, cannot function including ROS and reactive nitrogen-oxygen properly. species (RNOS), can initiate free-radical damage to lipids and proteins, leading to cell death. Kimberly Shane Villa 13 BIOCHEMISTRY LECTURE MIDTERMS Protection Mechanisms: Cells have plays a significant role in oxidative stress defense mechanisms to counteract ROS and and cellular damage. RNOS damage, including enzymes such as Hydrogen Peroxide (H202): superoxide dismutase (SOD) and glutathione While hydrogen peroxide is not a radical peroxidase. itself, it can easily generate hydroxyl Antioxidants: Vitamins C and E act as radicals through interactions with transition antioxidants, protecting cells from radical metals like iron (+2) and copper (+1). This damage and oxidative stress. ability to form hydroxyl radicals contributes Oxygen and the generation of ROS to its damaging effects in cells. 1. Oxygen as a Biradical: Reactive Nitrogen-Oxygen Species Oxygen (02) exists as a biradical, meaning it (RNOS): has two unpaired electrons in its outer RNOS are primarily derived from the orbitals. This makes it relatively unreactive free-radical nitric oxide (NO), which can in its ground state. combine with oxygen or superoxide to 2. Oxygen Reduction to Water: produce additional RNOS. This cascade of Oxygen can accept four electrons to become reactions involving nitrogen and oxygen fully reduced to water (H2O). This process radicals contributes to oxidative stress and occurs in stages, with each electron addition cellular injury. generating a different reactive species: ROS Reactions with Cellular Components 1. Lipid Peroxidation and Membrane Damage Chain Reactions: ROS, particularly the hydroxyl radical ( OH, can Characteristics of ROS initiate chain reactions in lipid Reactive Free Radicals: membranes. These reactions involve ROS are reactive free radicals that extract the abstraction of hydrogen atoms electrons from other compounds to complete from unsaturated fatty acids, forming their own orbitals. This initiates free-radical lipid free radicals. chain reactions, leading to cellular damage. Lipid Peroxide Formation: These Hydroxyl Radical ( OH): lipid free radicals readily react with The hydroxyl radical is the most potent oxygen, forming lipid peroxides. ROS, capable of initiating chain reactions Membrane Damage: The formation that result in the formation of lipid of lipid peroxides disrupts membrane peroxidation and organic radicals, structure and function, leading to contributing to cellular damage. increased permeability and loss of Superoxide (02°-): integrity. This damage can affect all Superoxide is highly reactive but has limited organelles, including mitochondria, solubility, restricting its range of action lysosomes, and the endoplasmic compared to other ROS. However, it still reticulum Kimberly Shane Villa 14 BIOCHEMISTRY LECTURE MIDTERMS Base Alterations: ROS can directly modify the chemical structure of DNA bases, leading to mutations and errors in genetic information. Strand Breaks: ROS can also cause single- and double-strand breaks in the DNA backbone, disrupting the integrity of the genetic code. Consequences: DNA damage caused by ROS can lead to: - Genetic Instability: Increased susceptibility to mutations and 2. Protein Oxidation and Dysfunction genomic rearrangements. Amino Acid Susceptibility: - Cancer Development: Mutations in Specific amino acids within proteins, critical genes involved in cell growth including proline, histidine, arginine, and proliferation can contribute to cysteine, and methionine, are the development of cancer. particularly vulnerable to attack by - Aging: Accumulated DNA damage hydroxyl radicals. is a hallmark of aging, contributing Oxidative Damage: This attack to age-related diseases. leads to oxidative damage, altering Formation of Free Radicals During the structure and function of Phagocytosis and Inflammation proteins. During the immune response to Consequences: Protein oxidation infectious agents and other stimuli, can result in: phagocytic cells of the immune - Protein Fragmentation: The system undergo a process known as breakdown of proteins into smaller the respiratory burst, characterized fragments. by a rapid consumption of oxygen. - Cross-linking: The formation of This leads to the generation of abnormal bonds between protein reactive oxygen species (ROS), molecules. hypochlorous acid (HOCI), and - Aggregate Formation: The reactive nitrogen-oxygen species clumping of proteins, leading to (RNOS) to aid in the destruction of protein misfolding and aggregation. phagocytosed materials. - Loss of Protein Activity: The Generation of ROS and Other disruption of protein function, Species: rendering them unable to perform - NADPH Oxidase: NADPH oxidase their essential roles in cellular catalyzes the formation of processes. superoxide (02 -) from oxygen and 3. DNA Damage and Genetic Instability NADPH. This superoxide is released Kimberly Shane Villa 15 BIOCHEMISTRY LECTURE MIDTERMS into the phagolysosome, where it is role in energy production. SOD2, the converted into other reactive oxygen mitochondrial isoform of SOD, is essential species (ROS) that play a role in for protecting these organelles from destroying the engulfed materials. superoxide damage. - Myeloperoxidase: Myeloperoxidase is an enzyme that generates hypochlorous acid (HOCI), a potent oxidant. HOCl is highly effective in destroying the contents of the phagosome, contributing to the immune response against pathogens. Catalase: Function: Catalase is another key enzyme in the antioxidant defense system. It catalyzes the breakdown of hydrogen peroxide (H202) into water (H20) and oxygen (02). This reaction prevents the formation of highly reactive hydroxyl radicals ( OH) from hydrogen peroxide. Location: Catalase is primarily found in Cellular Defenses Against Oxygen peroxisomes, Toxicity: Antioxidant Scavenging Importance: Peroxisomes are sites of Enzymes significant ROS production, making catalase Superoxide Dismutase (SOD): crucial for protecting these organelles and Function: SOD is a crucial enzyme that the cell as a whole from oxidative damage. converts superoxide radicals (02°-) into Glutathione Peroxidase (GPx): hydrogen peroxide (H202) and molecular Function: GPx is a family of enzymes that oxygen (02). This reaction dismutates reduce hydrogen peroxide (H202) and lipid superoxide, effectively reducing its harmful hydroperoxides, using glutathione as a potential. reducing agent. This reaction detoxifies Location: SOD is found in various cellular these reactive species and prevents them compartments, including the cytoplasm, from damaging cellular components. mitochondria, and extracellular spaces. The Location: GPx is found in various cellular specific isoforms of SOD (SOD1, SOD2, compartments, including the cytoplasm, SOD3) are localized to these different mitochondria, and endoplasmic reticulum. compartments. Importance: GPx plays a critical role in Importance: Mitochondria are particularly protecting cells from lipid peroxidation, a vulnerable to oxidative stress due to their Kimberly Shane Villa 16 BIOCHEMISTRY LECTURE MIDTERMS major form of oxidative damage that can disrupt membrane integrity. Other Antioxidant Enzymes: Glutathione Reductase (GR): GR maintains a supply of reduced glutathione (GSH), which is essential for the activity of GPx and other antioxidant enzymes. Thioredoxin Reductase (TrR): TrxR reduces thioredoxin, another important antioxidant that participates in various cellular processes, including DNA repair and redox signaling. Vitamin E (Tocopherol): A fat-soluble antioxidant that protects cell membranes from lipid peroxidation, a major form of oxidative damage. Helps prevent damage to cell membranes, reduces inflammation, and supports immune function. Beta-Carotene: Beta-carotene is a precursor to vitamin A and acts as a potent antioxidant, particularly against singlet oxygen, a highly reactive form of oxygen. It also protects cell membranes from ROS-induced damage. Kimberly Shane Villa 17