Biochem Exam 2 Lecture Notes 2024 PDF
Document Details
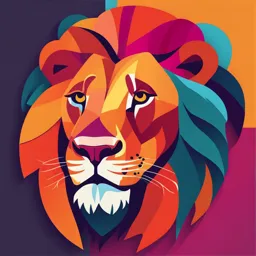
Uploaded by WieldyEpic
Dr. William M. Scholl College of Podiatric Medicine
2024
Tags
Summary
These lecture notes cover carbohydrate introduction, digestion, absorption, and glycolysis. They detail various enzymes and transport mechanisms involved in these processes.
Full Transcript
PBBS508 Biochemistry and Genetics Lecture note 2024 Lecture 12: Carbohydrate introduction Glucose Homeostasis Glucose is the common carbohydrate currency of the body. Blood glucose level should remain relatively constant to make sure that important organs receive enough glucose (and therefore ene...
PBBS508 Biochemistry and Genetics Lecture note 2024 Lecture 12: Carbohydrate introduction Glucose Homeostasis Glucose is the common carbohydrate currency of the body. Blood glucose level should remain relatively constant to make sure that important organs receive enough glucose (and therefore energy, ATP). Blood glucose concentration is the result of a balance between input and output, synthetic and catabolism. Dietary carbohydrate is digested in the gastrointestinal tract to simple monosaccharides, which are then absorbed into the enterocytes of the small intestine and systemic circulation. Carbohydrate Simple carbohydrate includes monosaccharides (glucose, fructose, galactose) and disaccharides (sucrose, lactose, maltose). Complex carbohydrate includes polysaccharide, e.g. starches, glycogen and fibers. Polysaccharide (except fibers) are digested to short oligosaccharides (dextrins) -> disaccharide -> monosaccharide. Then the monosaccharides are absorbed. Fibers (e.g. cellulose, pectin, beta-glucan, inulin) cannot be digested and therefore cannot be absorbed. Carbohydrate digestion Small intestine can absorb only monosaccharides. Therefore, all carbohydrate must be digested to monosaccharides before absorption. Carbohydrate digestion starts in our mouth by enzyme in saliva called salivary amylase that digest starch and glycogen to dextrin (short oligosaccharide). Then, the carbohydrates pass through stomach, where gastric acid destroys the salivary amylase (no digestion here). In small intestine, there are intraluminal digestion by pancreatic amylase, which further digest oligosaccharide providing disaccharide. Then all of disaccharides will be further digested by membrane digestion by enzymes disaccharidases on the mucosal cell membrane bound enzyme or brush border enzyme of enterocytes (small intestinal epithelial cells) monosaccharide. Disaccharidases include maltase (cleave maltose to 2 glucoses), lactase (cleave lactose to glucose + galactose) and sucrase (cleave sucrose to glucose and fructose). Monosaccharides are then are absorbed by enterocytes and circulation using different transporters and further used for metabolism. Some carbohydrates that are not hydrolyzed by these enzymes including some di-, oligo-, and polysaccharides will go to large intestine and will be digested by bacteria. After digestion by bacteria, CO2, methane, hydrogen gases, and short chain fatty acids are produced which can cause some bloating and gases. Lactose intolerance is one of the examples of effects from undigested molecules in people with PBBS508 Biochemistry and Genetics Lecture note 2024 lactase deficiency. Lactose cannot be digested therefore remain in the large intestine causing osmotic diarrhea. Lactose will pass to large intestine and digested (fermented) by bacteria producing 2 or 3 carbon metabolites, which worsen the diarrhea, as well as CO2, methane and hydrogen gases (H2), which causes cramping and bloating. H2 can be measured in the breath. Carbohydrate absorption There are 2 transporters (SGLT1 and GLUT5 (Glucose Transporter type 5) for monosaccharide absorption INTO enterocytes and GLUT2 to transport all monosaccharides OUT of enterocytes - Sodium/Glucose cotransporter-1 (SGLT-1): the secondary active transport mechanism using Na+ gradient established from NA+/K+ ATPase to transport glucose (and galactose) INTO enterocytes. - GLUT-5: facilitated diffusion of fructose into the enterocyte with no energy requirement (driven by fructose concentration). - GLUT-2 (at contraluminal membrane of enterocytes): facilitated diffusion which can transport all monosaccharides OUT of enterocytes into the blood stream. Glucose uptake in tissues After glucose enter to blood stream, there are several facilitated diffusion mechanisms (which are transporters constitutively located at the membrane) helping tissues to uptake glucose. GLUT-1, GLUT-2, GLUT-3, GLUT-5 are insulin independent. GLUT4 is insulin dependent (need insulin in order to be inserted in the membrane and uptake glucose. GLUT4 are found mainly in muscle, adipose tissues (heart expresses both GLUT 1 and some GLUT4). GLUT2 is expressed in liver, kidney, pancreas and small intestine. In liver and kidney, it works bidirectionally to uptake and release glucose into blood. In intestine, it’s located at the contraluminal membrane of enterocytes transport all monosaccharides out of enterocytes into the blood stream. In beta cells of the pancreas, GLUT2 is a major glucose transporter that is important for insulin secretion. PBBS508 Biochemistry and Genetics Lecture note 2024 Lecture 13 Glycolysis Glycolysis is the metabolic pathway that converts glucose into pyruvate and generate ATP. If the cells have mitochondria and oxygen the glycolysis is aerobic. If either mitochondria or oxygen is lacking, glycolysis is anaerobic. The needed of glycolysis are varied among tissues, the major consumer of glucose via glycolysis is the brain (under aerobic condition) and muscles (especially during anaerobic condition- strenuous exercise). Glycolysis is very important for some organs that have a few mitochondria or blood supply. For example, red blood cells (RBCs) don’t have mitochondria and therefore rely on anaerobic glycolysis for the energy source. Stages of glycolysis Stage I (investment phase): utilize 2 ATP molecules ***Reaction 1. Glucose à Glucose-6-Phosphate: this step uses 1 ATP to add Pi into C atom number 6 of glucose (phosphorylate), yielding glucose-6-phosphate. This step drives the glucose from outside the cells to flow in since glucose inside is converted to glucose-6-phosphate and is trapped inside the cells. This step is catalyzed by enzyme Hexokinase (low Km (high affinity) and low Vmax) in muscles and most of tissues, or Glucokinase (specific form of hexokinase found in liver and pancreatic b cells). This process is irreversible. Reaction 2. Glucose-6-phosphate à fructose-6-phosphate by enzyme phosphoglucose isomerase that isomerizes Glucose-6-phosphate to fructose-6-phosphate (glucose and fructose are isomers). ***Reaction 3: Fructose-6-phosphate à fructose 1,6- bisphosphate: the second step that uses ATP to add Pi into C atom number 1 resulting in fructose-1,6 biphosphate (F-1,6-BP), by phosphofructokinase 1 (PFK1, irreversible). PFK1 is the “rate limiting enzyme”*** for glycolysis. Reaction 4: Fructose 1,6 biphosphate à glyceraldehyde-3-phosphate (GAP) & dihydroxyacetone- phosphate (DHAP): this step splits the 6C molecules into 2 molecules of 3C (DHAP and GAP), by Aldolase. Reaction 5: DHAP can be reversibly converted to GAP by triose phosphate isomerase enzyme. However, The glyceraldehyde 3-phosphate (GAP) is the only product that further be used for the next steps of glycolysis. Therefore, the concentration of the GAP is decreased (because it’s pulled to be used in the further steps) and drive the reaction towards GAP production. At the end all DHAP is converted to GAP resulting in 2GAP molecules from 1 glucose. Stage II (Payoff phase): generate ATPs, NADH, and final product either pyruvate or lactate. The processes in this step occur twice (because of 2 GAP molecules/1 glucose). (Step 6-9 are reversible and therefore are not targets of regulations) PBBS508 Biochemistry and Genetics Lecture note 2024 Reaction 6: Glyceraldehyde-3-phosphateà 1,3-biphosphoglycerate: this step oxidizes glyceraldehyde-3- phosphate and phosphorylate at the same time. Glyceraldehyde-3-phosphate gives electron to NAD+, yielding NADH and Pi is added to glyceraldehyde-3-phhosphate yielding 1,3-biphosphoglycerate. Reaction 7: 1,3-bisphosphoglycerate à 3-phosphoglycerate: this step removes Pi from the 1,3- bisphosphoglycerate by phosphoglycerate kinase, yielding 3-phosphoglycerate, which generating the first ATP (substrate level phosphorylation). Reaction 8: 3-phosphoglycerate à 2-phosphoglycerate: the shifting of Pi to carbon atom number 2, by phosphoglycerate mutase. Reaction 9: 2-phosphoglycerate à phosphoenolpyruvate (PEP): this dehydrating step removes water from the molecule by enzyme enolase, yielding phosphoenolpyruvate (PEP) ***Reaction 10: Phosphoenolpyruvate (PEP) à pyruvate: this step removes Pi from PEP to ADP, yielding ATP and pyruvate as a final product, by pyruvate kinase, another key enzyme in glycolysis (irreversible). There are 2 conditions of glycolysis - Under aerobic condition (when mitochondria and O2 is present): 10 reactions Reaction: Glucose + 2 Pi + 2 ADP + 2 NAD+ à 2 pyruvate + 2 H+ + 2 ATP + 2 NADH + 2 H2O - Under anaerobic condition (without mitochondria and/or absent of O2): 11 reactions Reaction: Glucose + 2 Pi + 2 ADP à lactate + 2 H+ + 2 ATP + 2 H2O Anaerobic condition After pyruvates are generated, the pyruvates can be used to generate lactate under anaerobic condition. In this step, lactate dehydrogenase change pyruvate to lactate, and NADH is required in this reaction. Once NADH is used in this step, the NAD+ will be regenerated and used in glycolysis again. So, this conversion of pyruvate à lactate (anaerobic) is important in order to regenerating NAD+ for glycolysis to be continued (in order to keep providing ATP to the cells/tissues). Glycolysis regulation Major 3 enzymes in glycolysis are the targets of regulation including hexokinase/glucokinase, PFK-1, and pyruvate kinase. The major hormones controlling these 3 enzymes are insulin and glucagon, working in opposite way. Insulin will increase the synthesis of the enzymes (stimulate glycolysis), while glucagon will decrease the synthesis of enzyme (shut down glycolysis – in order to maintain glucose in blood). 1. Hexokinase/Glucokinase regulation: PBBS508 Biochemistry and Genetics Lecture note 2024 Hexokinase (found in muscles and most tissues) has low Km (high affinity) and can reach Vmax quickly (low Vmax). Therefore, it can function with the low level of glucose. Hexokinase can be inhibited by its product, glucose-6-phosphate. Glucokinase (another isoform of hexokinase found in liver and pancreatic beta cells): it has higher Km (low affinity) than hexokinase. After hexokinase reach its maximum and the glucose concentration is really high (higher than 5 mM), the glucokinase in the liver will be turned on. Glucokinase is also found in pancreatic beta cells which is used as a mechanism to sense increased glucose and stimulate insulin secretion. Glucokinase can be inhibited by fructose-6-phosphate. (Detail: it does so by inducing the binding of glucokinase with its regulatory protein (GKRP) and sequestering it in the nucleus -> decrease liver glycolysis. In contrast, increased glucose concentration will trigger dissociation of glucokinase from the GKRP, activating glycolysis in the liver). 2. Phosphofructokinase (PFK-1)***: is the rate limiting enzyme*** and the major regulation point of glycolysis AMP is an allosteric activator of PFK-1 -> enhance glycolysis in the low energy stage. ATP and Citrate (from citric acid cycle) is an allosteric inhibitor of PFK-1 (suggesting energy is already enough, no more glycolysis required). Note: High AMP or ADP levels (or high AMP/ATP or ADP/ATP ratio) are indicators for low energy. High ATP is an indicator for high energy in the cells*. Lactic acid from the utilization of glucose during anaerobic condition also can inhibit PFK-1 activity, inhibit glycolysis (prevent further decrease in intracellular pH). The most potent activator of PFK-1 is Fructose 2,6-bisphosphate, which is also produced from fructose- 6-phosphate (F6P) by enzyme PFK-2. Abundance of F6P results in a higher concentration of Fructose 2,6- bisphosphate (F-2,6-BP). The binding of F-2,6-BP increases the affinity of PFK1 for F6P and diminishes the inhibitory effect of ATP. Insulin activates PFK-2 increasing F2,6-BP, and therefore activates PFK1 and glycolysis. Glucagon has the opposite effect. 3. Pyruvate kinase (PK): the last step of regulation in glycolysis ATP is an allosteric inhibitor of PK. High ATP -> PK activity is inhibited. Fructose 1,6-biphosphate is an activator of PK, this is a feed forward effect to push intermediates through glycolysis. ** Pyruvate kinase (PK) deficiency is a rare genetic defect. The patients usually have only 5-25% of pyruvate kinase in their RBC, resulting in low ATP production (because RBCs do not have mitochondria and therefore only get ATP from glycolysis. Low ATP à RBC cannot maintain its shape à hemolytic anemia (also presented with common lower extremity non-healing ulcer). PBBS508 Biochemistry and Genetics Lecture Note 2024 Lecture 14-15: Energy Pathways I-II Pyruvate dehydrogenase (PDH) and Krebs cycle The hub of metabolism is mitochondria. The first step of converting pyruvate to Acetyl-CoA by enzyme “Pyruvate dehydrogenase complex (PDH)”, located in mitochondria matrix. This is an irreversible reaction using major 3 enzymes in the complex [pyruvate dehydrogenase, dihydrolipoyl transacetylase, dihydrolipoyl dehydrogenase] and 5 cofactors [thiamine pyrophosphate (from Vit B1), lipoic acid, CoA (from Vit B5), FAD (from Vit B2) and NAD+ (from Vit B3). The regulation of PDH PDH is regulated by the negative feedback mechanism of NADH and acetyl CoA, the inhibition can be enhanced in the catabolism of long-chain fatty acids (provide high acetyl CoA), which will be discussed later in lipid metabolism. High energy (ATP), NADH, Acetyl CoA -> inhibit PDH reaction Pyruvate and Ca++ -> activate PDH reaction Additional note: The PDH is active in “non-phosphorylated form”(via phosphatase enzyme) and inactive when in “phosphorylated form” (via kinase enzyme). Those phosphatase enzymes are regulated by calcium, while the kinase enzymes are activated by ATP, NADH, acetyl CoA (meaning the energy is already high, the further production of energy can be decreased). Defect in PDH reaction The deficiencies in PDH is very rare, however, if the deficiencies is occurred, it would mostly affect the tissue where with highly metabolism including brain, skeletal muscle, and heart. Particularly for the PDH deficiency in the brain, it can cause cerebral lactic acidosis and encephalopathies. Vit B1 (thiamine) is an important coenzyme for PDH. Deficiency in vit B1 (from malnutrition or alcoholic) can cause Beriberi disease (neurological or cardiovascular disease). Patients with this disease cannot oxidize pyruvate normally and elevated pyruvate in blood could be found. Severe B1 deficiency can cause Wernicke-Korsakoff syndrome (WKS), which cause damage to the brain. The citric acid cycle/TCA/Krebs cycle TCA occurs in mitochondria, it’s the oxidation of Acetyl CoA (2C) to 2CO2 (2 carbons enter, 2 leave the cycle). The purpose of Krebs cycle is to produce NADH*, FADH2*, as electron carriers, in order to be used in the electron transport chain (ETC). Net reaction: Acetyl CoA + 3NAD+ + FAD + GDP+Pi à 2CO2 + 3NADH + FADH2 + GTP PBBS508 Biochemistry and Genetics Lecture Note 2024 There are 4 redox reactions occurred in the cycle to produce high energy molecule; NADH, FADH2 and GTP (ATP equivalent). The important enzymes in this cycle are the first enzyme Citrate synthase* and dehydrogenase enzymes*, which are in several part of the cycle generating NADH and FADH2. These enzymes are targets of the regulation. TCA regulation: Similar ideas with the glycolysis; energy levels regulate the reaction*** Citrate synthase* (Acetyl CoA + Oxaloacetate à Citrate), the first step of the cycle (inhibited by high level of citrate) ATP, NADH, GTP (signal for high energy) -> inhibit TCA (no more energy is needed) Isocitrate dehydrogenase*** (isocitrate àa-ketoglutarate) is RATE-LIMITING ENZYME of the TCA cycle. It is activated by low energy (ADP) and Ca++ Alpha-ketoglutarate dehydrogenase (a-ketoglutarate à succinyl CoA) is inhibited by its product, succinyl CoA and NADH. Arsenic poisoning: arsenic binds to lipoic acid, a cofactor of PDH and dehydrogenase enzymes, therefore inhibit TCA cycle. Anabolic functions of the TCA cycle TCA cycle intermediates serve as junction points in metabolism. When needed, intermediates can be channeled to synthesize biomolecules (fatty acids, steroids, glucose, heme, neurotransmotters and amino acids). - Citrate is use as carbon source for fatty acid and steroid synthesis - Alpha-ketoglutarate is used as an intermediate for synthesis some amino acids - Succinyl CoA: essential for heme synthesis - Oxaloacetate and malate: are used as a major precursor for gluconeogenesis. As these intermediates are taken out from the cycle, the replenishment is done by anaplerotic reactions. These intermediates include oxaloacetate and alpha-ketoglutarate from some amino acids, and Succinyl CoA (from fatty acids). For example, Oxaloacetate can be derived from Pyruvate using the major enzyme “pyruvate carboxylate”. In the excess of acetyl CoA, the production of oxaloacetate from pyruvate is occurred. PBBS508 Biochemistry and Genetics Lecture Note 2024 Electron transport chain and oxidative phosphorylation The electron transport chain and oxidative phosphorylation are terminal steps of oxidation occur in mitochondria. The electron received from Krebs cycle in form of NADH and FADH2 will be transferred through protein complexes in the inner membrane of mitochondria, and the final acceptor of electron will be oxygen (this is why O2 is necessary for life). During electron transport, the H+ will be pumped from the matrix to the intermembrane space of mitochondria by complex I, III, and IV to generate H+ gradient, which will drive ATP synthase to produce ATP. The components of electron transport chain (ETC) Electron transport chain complex includes 4 large multi-subunits of enzymes (complex I-IV), 2 mobile electron carriers, coenzyme Q and cytochrome C. In electron transport chain, vitamins (NAD+, FAD, FMN) and minerals (iron and copper) are important to maintain proper functions of the system. Complex I: NADH dehydrogenase (or NADH-Coenzyme Q reductase). The first reaction, NADH is used to reduce the complex. Once the complex receives the electron it can pump H+ from matrix to the intermembrane space. Complex II: Succinate dehydrogenase (or succinate CoQ reductase). The FADH2 from TCA cycle is used with this complex. No H+ pumping in this step. Coenzyme Q (ubiquinone) receives electrons from both complex I and complex II, then transfer them to complex III. Complex III: Cytochrome b-c1 or CoQ-cytochrome C oxidoreductase receives electron from Coenzyme Q and pump out H+. Cytochrome C, water soluble, mobile electron carrier. It transfers electron from complex III to complex IV. Complex IV (or cytochrome oxidase): receives electrons from cytochrome C and pass electron to the last electron acceptor (O2) to form H2O. It also pumps H+ out. *** O2 is important in this step, in the condition of absence O2, the whole system will back up and stop! Toxins affecting ETC Barbiturate and rotenone: inhibit the electron flow from complex I to Coenzyme Q Cyanide and CO prevent electron transferring from complex IV to oxygen. PBBS508 Biochemistry and Genetics Lecture Note 2024 The oxidative phosphorylation/ATP production: The last step, after electrons are transported through 4 complexes, the H+ that are pumped out to inner membrane space generated H+ gradient and membrane potential, which drive ATP synthesis. The H+ move back to inside matrix through ATP synthase (F1F0ATPase). F0 portion makes membrane permeable to protons and F1 contain ATPase enzyme that catalyze ATP synthesis” The ATP synthesized in matrix are then transported out from matrix by ATP/ADP translocase, which is antiport system (move ATP out of the matrix with ADP in). Heat generation: Besides using H+ for ATP synthesis, H+ in the inner membrane space can be used to generate heat by passing through the uncoupling protein UCP-1 (thermogenin) instead of ATP synthase (generate heat instead of generating ATP). This process is found mostly in hibernating animals, newborn animals, and brown adipose tissue in human (high in infant). Some chemical compounds behave as uncouplers (eg. 2,4-DNP and high dose aspirin) which eventually disrupt the ETC. Respiratory control: The regulator of electron transport chain is ATP/ADP ratio. In the condition of energy needed (ATP is needed), the level of ADP is high (as an indicator of ATP break down), electron transport chain and oxidative phosphorylation is increased. When the ATP/ADP ratio is high, ATP can inhibit dehydrogenase enzymes in TCA cycle. The NADH and FADH2 are not consumed by electron transport chain and therefore are accumulated and inhibit TCA cycle (NAD+ and FAD levels are also low -> so the TCA cycle will slow down). With the limited amount of Oxygen, rate of oxidative phosphorylation is decreased, increasing NADH and FADH2 (and decrease NAD+ and FAD), so the TCA cycle will also slow down. Ischemia*: refers to the restriction in blood supply, causing the cell to unable to utilize oxygen for respiration through ETC, then the cells will die. Once the cells unable to utilize O2, they will compensate by increasing anaerobic glycolysis which can be characterized by decreased blood pH (from lactic acid). PBBS508 Biochemistry and Genetics Lecture Note 2024 Lecture 16-17: Gluconeogenesis I-II Blood glucose: Blood glucose level needs to be maintained in a normal range (Fasting ~ 70-100 mg/dl), which is necessary to maintain normal function of several organs: Brain, RBC, Kidney medulla, lens, cornea, and testis. Once blood glucose drops below 70 mg/ml our body will start release glucagon, epinephrine and cortisol. However, if the hormone releasing is not function properly, our body is in hypoglycemic condition. Some symptoms will occur including sweating, trembling, convulsion, eventually permanent brain damage or even death. Fasting blood glucose higher than 100 mg/dl is considered hyperglycemic condition. If blood glucose is constantly higher than 125 mg/dl, it is the considered diabetes mellitus. Glucose homeostasis: 3 processes are working in cycle to keep blood glucose level constant. 1. Dietary intake causes high blood glucose for a while, which trigger secretion of insulin. Insulin starts to uptake the glucose into muscles or adipose for glycolysis. After that around 2 hours, blood glucose will drop (between meals). 2. Between meals, glycogenolysis will take place by utilizing glycogen storage (liver) releasing glucose into the blood. Then, the next meal comes and spike the level of blood glucose again. 3. During fasting period or during sleep (no dietary intake for several hours), body will start to synthesize new glucose (gluconeogenesis) to maintain blood glucose level. Gluconeogenesis is the process of synthesis new glucose (gluco-sugar, neo–new, genesis-make/synthesize) from non-carbohydrate sources. Mostly, gluconeogenesis occurs during period of fasting, low-carbohydrate diets, or intense exercise (when glycogen is running out). Around 90% of gluconeogenesis takes place in liver and 10% in kidney. During prolong starvation (>48 hours) the kidney can account for up to 40% of gluconeogenesis. Gluconeogenesis is the opposite of glycolysis. There are 3 irreversible reactions in glycolysis that are need to be bypassed for gluconeogenesis. Therefore, the bypass steps with some additional enzymes (and energy) are required. The reactions require both cytosolic and mitochondrial enzymes. Three irreversible reactions in glycolysis that are need to be bypassed for gluconeogenesis. è Pyruvate kinase is bypassed into 2 steps è PFK-1 is bypassed by using phosphatase reactions è Glucokinase is bypassed by using phosphatase reactions PBBS508 Biochemistry and Genetics Lecture Note 2024 ***4 Gluconeogenesis bypass steps 1. Converting pyruvate into PEP: by using coupling two reactions, pyruvate will be converted to Oxaloacetate by Pyruvate Carboxylase*** (found only in mitochondria), which requires biotin (Vit B7) as a coenzyme and need ATP and CO2. Then, oxaloacetate must be converted to malate in order to be transported out to the cytoplasm. The transported malate is then converted back to oxaloacetate, in cytoplasm. Oxaloacetate is then decarboxylated and phosphorylated in the cytosol by phosphoenolpyruvate carboxykinase (PEPCK) to yield Phosphoenolpyruvate (PEP). The GTP is used as a phosphoryl donor. Phosphoenolpyruvate (PEP) is then further gone through reversible glycolysis reactions until giving rise fructose 1,6 bisphosphate. 2. Fructose 1,6-bisphosphate dephosphorylation to become fructose-6 phosphate (bypassing the phosphofructokinase step) by a rate-limiting enzyme, Fructose-1,6-biphosphatase***. Then, fructose 6- phosphate is converted to glucose 6-phosphate by the reversible reaction using phosphoglucose isomerase. 3. Converting glucose 6-phosphate into glucose: by Glucose 6-phosphatase*** (bypassing glucokinase step). *Glucose-6-phosphatase is presented in the ER of the glucogenic tissues (liver and kidney). The glucose 6-Phosphate must be translocated from cytosol to the ER by Glucose 6-P translocase. After glucose is produced, the glucose can be released to the blood stream. Without Glucose 6-phosphatase and Glucose 6-P translocase glucose cannot be produced (G-6P cannot be dephosphorylated), leading to severe, fasting hypoglycemia. Overall reaction (note that the reaction use ATP and GTP: consume energy) 2 pyruvate + 4 ATP + 2 GTP + 2 NADH + 6 H2O à Glucose + 4 ADP + 2GDP + 6Pi + 2 NAD + 2H+ ***Substrates for gluconeogenesis Major substrates for gluconeogenesis are glycerol from adipose tissue and amino acids and lactate from muscles. The prolonged fasting/malnutrition/starvation can cause the loss of both adipose and muscle mass. 1. Lactate is one of the substrates for gluconeogenesis. Lactate molecules from blood (RBC) and from anaerobic glycolysis in muscle cells enter blood stream and go to liver. Lactate molecules are used to generate pyruvates, which will then enter to gluconeogenesis pathway producing glucose. Glucose then enter the muscles and RBC for glycolysis. This cycle of lactate-glucose between muscle/RBCs and liver is called “The Cori cycle”. 2. Amino acids produced by hydrolysis of tissue proteins generate pyruvate, which can enter gluconeogenesis. Some amino acid metabolisms generate alpha-ketoglutarate which can enter the TCA PBBS508 Biochemistry and Genetics Lecture Note 2024 cycle and form Oxaloacetate (OAA), a direct precursor of PEP. All of amino acids EXCEPT leucine and lysine can be catabolized to be used in gluconeogenesis. 3. Glycerol from breakdown of triacylglycerols (TAGs): The glycerol backbone from the breakdown of lipid can be used as a substrate for gluconeogenesis by the enzyme in the liver (glycerol kinase). Glycerol kinase phosphorylate glycerol to glycerol-3-phosphate. Then it is dehydrogenated to become dihydroxyacetone phosphate (DHAP), which can enter to gluconeogenesis pathway. *Fatty acid cannot provide substrate for gluconeogenesis since it provides Acetyl CoA which cannot be converted back to pyruvate. ***The regulation of gluconeogenesis: Gluconeogenesis and glycolysis are reciprocally regulated. Allosteric effectors ***High AMP (low energy) inhibit gluconeogenesis/activate glycolysis (preserve energy) è inhibit Fructose 1,6 bisphosphatase in gluconeogenesis (inhibit the energy-requiring reaction) è stimulate PFK-1 in glycolysis (stimulate energy-producing pathways). ***High level of Citrate and ATP (high energy) activate gluconeogenesis/ inhibits glycolysis è stimulate Fructose 1,6 bisphosphatase in gluconeogenesis è inhibit PFK-1 in glycolysis ***Acetyl CoA The 2 major mitochondrial enzymes that use pyruvate, pyruvate carboxylase (PC) and pyruvate dehydrogenase (PDH) are both regulated by Acetyl-CoA. Between meals, when fatty acids are oxidized in the liver for energy, high amount of Acetyl-CoA is produced. Acetyl-CoA activates pyruvate carboxylase to converting of pyruvate à oxaloacetate (facilitate gluconeogenesis). It also inhibits PDH, preventing conversion of pyruvate to acetyl-CoA for TCA (because the cells already have a lot of Acetyl CoA, no more conversion is needed). Alcoholism: Ethanol can inhibit gluconeogenesis due to the excessive production of NADH. Effects of high NADH 1. Push pyruvate à lactate reaction, decreasing pyruvate as a substrate for gluconeogenesis. 2. Push DHAP à glycerol-3-phosphate reaction, decreasing DHAP for gluconeogenesis. 3. Push OAA à Malate reaction, decreasing OAA as a substrate for gluconeogenesis. Glycerol-3-phosphate accumulation also contribute to lipid accumulation in alcoholic liver disease. PBBS508 Biochemistry and Genetics Lecture Note 2024 Other carbohydrates (Fructose and Galactose) Similar to glucose, fructose and galactose are phosphorylated after entering the cell to be trapped inside. Fructose metabolism: fructose comes from sucrose, which is cleaved by an enzyme sucrase in the intestine to become glucose + fructose. Fructose can also be found in many fruits and honey. Fructose is transported into the cells is insulin independent glucose transporter, GLUT-5. Many tissues in the body uptake fructose, including the liver, kidney, intestine, adipose tissue, muscle, sperm cells but liver is the major tissue that catabolize fructose. In liver and kidney, fructose is phosphorylated by enzyme fructokinase* to become fructose-1-phosphate (Fructose 1-P). Hexokinase in other tissues can also slowly phosphorylates fructose if the intracellular fructose concentration is unusually high (Hexokinase has low affinity (high Km) for fructose). Fructose 1-P is then cleaved by Aldolase B*** enzyme, providing DHAP and GAP, which can be used in glycolysis and gluconeogenesis pathway. Disorder of fructose metabolism 1. The deficiency of fructokinase can cause fructosuria, high urinary fructose (benign). 2. *Hereditary fructose intolerance (HFI) is an autosomal recessive disorder from the deficiency of Aldolase B enzyme. Lacking aldolase B causes the accumulation of F-1-P in liver, kidney, and small intestine, which is then inhibit glycogen breakdown and gluconeogenesis (because the Pi and ATP are depleted). This can induce severe hypoglycemia following ingestion of fructose. Symptoms are reversed after removing fructose and sucrose from the diet. Sorbitol synthesis: Aldose reductase enzyme found in many tissues (retina, lens, kidneys, peripheral nerves, ovaries, and seminal vesicles) reduces glucose, producing sorbitol (when glucose is high). Sorbitol dehydrogenase then oxidizes sorbitol to fructose. Sorbitol dehydrogenase is only expressed in some tissues e.g. liver, ovaries, and seminal vesicles. The two-reaction pathway from (glucose -> sorbitol -> fructose) benefits sperm cells which use fructose as a major carbohydrate energy source and liver (glycolysis). In hyperglycemia (e.g. in uncontrolled diabetes), large amounts of glucose enter retina, lens, kidneys, and peripheral nerves. High level of glucose can bind to aldose reductase and are converted sorbitol. In the cells that lack of sorbitol dehydrogenase e.g. the retina, lens, kidneys, and peripheral nerves sorbitol cannot be converted to fructose and therefore is trapped inside the cells. Sorbitol accumulation in these cells causes strong osmotic effects and cell swelling due to water influx and retention. Some of the pathologic alterations associated with diabetes can be partly attributed to this osmotic stress, including cataract formation, peripheral neuropathy, nephropathy and retinopathy. PBBS508 Biochemistry and Genetics Lecture Note 2024 Galactose metabolism (majorly from milk): Galactose uptake is insulin-independent (by SGLT1). Once absorbed from the small intestine, galactose travels through the portal vein and is primarily taken up by the liver cells. The small amount can be metabolized in brain, muscles and other tissues. Galactose is phosphorylated by galactokinase, yielding Gal 1-P. Gal 1-P can be converted to UDP-galactose (activated form) by the enzyme Gal 1-P uridyltransferase and produce Glucose 1-P, which can be used in glycogenesis or further converted to glucose-6P for glycolysis or gluconeogenesis. Disorder of galactose metabolism *Classic galactosemia is an autosomal recessive disorder caused by deficiency of galactose-1-P uridyl- transferase which cause the severe disease. Patients often have cataract formation (because accumulation of galactose enter the lens and is converted to galactitol). Accumulation of galactose-1-P also causes growth failure, mental retardation, and liver damage. PBBS508 Biochemistry and Genetics Lecture note 2024 Lecture 18: Glycogen Glycogen is one form of glucose storage found in liver and skeletal muscle. The glycogen in liver is mainly used for maintaining blood glucose***, while glycogen in skeletal muscle is used for energy production***. Glycogen is stored in the cytoplasm as single granules (skeletal muscle) or as clusters of granules (liver). The granule has a central protein core with poly-glucose chains radiating outward to form a sphere. Glycogen is a branch chain containing many D-glucose molecules linked together. Primary linkage is alpha 1,4 linkage (linear chain) and in every 8-10 residues the branches will be made by alpha 1-6 linkage. The branches structures of glycogen facilitate the rapid degradation and synthesis. Glycogen synthesis (glycogenesis) 1. Uridine diphosphate glucose (UDP-Glucose) synthesis: glucose is phosphorylated by enzyme Glucokinase (liver) or hexokinase (muscle) to become Glucose 6-P. Glucose 6-P is then converted to Glucose 1-P by enzyme phosphoglucomutase. UDP is added to Glucose 1-P yielding UDP-glucose. This step is done by “UDP-glucose pyrophosphorylase”, releasing 2 Pi molecules (which equivalent to the consumption of 2 ATP). 2. Primer synthesis: addition of UDP-glucose (activated) molecules to the glycogen is performed by glycogen synthase. However, Glycogen synthase cannot initiate chain synthesis using free glucose as an acceptor molecule. It can only add the glucose to the existing glycogen fragments. Therefore, for de novo synthesis, the primer is needed. Glycogenin is a protein that can accept glucose from the UDP- glucose. Glucose is attached to the hydroxyl group (OH) of tyrosine residue. Glycogenin catalyzes the transfer of at least 4 molecules of glucose from UDP-glucose, producing a short, alpha 1-4 link glucosyl chain, which serves as a primer for elongation. 3. Chain elongation: the new glucose from UDP-glucose will be added to the chain by alpha 1-4 glycosidic linkage, continuously, growing the linear chain. This step is done by Glycogen Synthase* enzyme. 4. Branch formation: once glucoses are added to the chain, forming the long chain of glucose, the branching enzyme (amylo alpha(1-4)->alpha(1-6)-transglycosylase) will recognize this long chain and break the alpha 1-4 linkage and transfer the chain of ~5-8 glucosyl residues to form alpha 1-6 linkage. PBBS508 Biochemistry and Genetics Lecture note 2024 Glycogen degradation (glycogenolysis) Chain shortening 1. The cleavage of alpha 1-4 glycosidic bond is occurred in the free ends of glycogen branches releasing Glucose 1-P. This is done by glycogen phosphorylase*** enzyme, which is a rate limiting enzyme for glycogenolysis. This enzyme can cleave the chain until only 4 glucose residues left on that branch (limit dextrin). Branch removal 2. The 3 outer residues on limit dextrin are then removed, and put back to the non-reducing end (free end) of the long chain, by the transferase activity of debranching enzyme (oligo-alpha(1- 4)->alpha(1-4)glucan transferase). 3. The last one residue is then removed by glucosidase activity of debranching enzyme (amylo- alpha(1-6)-glucosidase) releasing free glucose. Free glucose can either enter to blood stream for maintain blood glucose or be used as an energy for muscle. The Glucose 1-P released from the process is then converted to Glucose 6-P by phosphoglucomutase. Glucose 6-P will be further processed differently in liver and muscles. - In liver; Glucose 6-P will be translocated to ER by Glucose 6-P translocase and converted to glucose, via Glucose 6-P phosphatase***, releasing glucose to the blood. - In muscle; muscles do not have enzyme Glucose 6-P phosphatase therefore, Glucose 6-P will enter glycolysis pathway and produce ATP for muscle contraction. Lysosomal degradation: The small amount of glycogen are continuously degraded by lysosomal enzyme. The purpose of this is still unknown but deficiency in this enzyme can cause Pompe disease (lysosomal storage disease)*. Regulation of glycogen synthesis and degradation The controlling of glycogen synthesis and degradation are occurred in reciprocal fashion by both allosteric and hormonal regulation, through the regulation of enzymes glycogen synthase*** and glycogen phosphorylase***. Hormonal regulation Glucagon/epinephrine: in the condition of energy needed state (eg. during fasting or exercise) causes activation of glycogen phosphorylase, which promote glycogenolysis and causes inhibition of glycogen synthase leading to inhibition of glycogenesis. PBBS508 Biochemistry and Genetics Lecture note 2024 Insulin: released in the condition of well-fed state, oppose the action of glucagon. Insulin leads to activation of glycogen synthase, promoting glycogenesis and inhibits glycogen phosphorylase, decreasing glycogenolysis. Detail mechanism of insulin and glucagon Glucagon and epinephrine bind to G-protein couple receptor, activates adenylyl cyclase à produce cAMP à activate PKA à activate glycogen phosphorylase kinase à phosphorylation of glycogen phosphorylase (active form), which will then degrade glycogen (promote glycogenolysis). Insulin can activate protein phosphatase (PP1) à dephosphorylates glycogen phosphorylase and glycogen phosphorylase, which shut down the glycogen breakdown. It can also activate phosphodiesterase enzyme which breakdown cAMP in the cells. Therefore, oppose the actions of glucagon. Allosteric regulation - Glycogen synthesis: both in liver and muscle, G-6-P in well fed state can allosterically activates glycogen synthase, increasing glycogen synthesis. - Glycogen degradation: both in liver and muscle, G-6-P and ATP* in high energy state can allosterically inhibit glycogen phosphorylase, decreasing glycogen degradation. Additionally in muscle, when muscle is in the immediate need state, Ca++ and AMP* also can activate muscle glycogen phosphorylase (myophosphorylase) to breakdown the glycogen (need more energy (ATP) -> time to break-down more glycogen!). In liver, high amount of free glucose can inhibit glycogen phosphorylase and therefore inhibit glycogenolysis (enough glucose, no more glycogen break-down). Glycogen storage disease (GSDs) 1. ***Von Gierke disease (GSD I): the deficiency of glucose-6-phosphatase in liver and kidney (convert G 6-P to glucose), which cause the patients fail to utilize G-6-P from glycogen breakdown for blood glucose maintaining. This can cause severe fasting hypoglycemia and lactic acidosis (*Note that glucose-6-phosphatase is also required for the last step of gluconeogenesis). 2. *Pompe’s disease (GSD II): the deficiency in alpha 1-4 glucosidase (lysosomal degradation) leading to massive increase in amount of glycogen and develop cardiorespiratory failure. 3. *Cori’s disease (GSD III): the deficiency of debranching enzyme (amylo-1,6-glucosidase), and therefore cannot degrade branches of glycogen causing glycogen accumulation and obtain less glucose from glycogenolysis. The symptoms are similar to those found in GSD I, but much milder. PBBS508 Biochemistry and Genetics Lecture note 2024 4. ***McArdle’s disease (GSD V): the absence of muscle phosphorylase enzymes (myophosphorylase). Muscles cannot breakdown glycogen and therefore are unable to utilize glycogen stored for energy production. This can cause painful muscle cramps during strenuous exercises. 5. *Hers (GSD VI): the absence of liver phosphorylase enzymes, leading to defect in glycogenolysis. Clinical features are similar to those found in type I but milder. PBBS508 Biochemistry and Genetics Lecture Note 2024 Lecture 19: HMP/PPP NADPH & ROS The pentose phosphate pathway (PPP) or hexose monophosphate shunt (HMP) is the anabolic pathway. Key purposes of this pathway are. - Provide Ribose-5-Phosphate (R5P) for nucleotides and nucleic acids synthesis - Generate NADPH The tissues that have large amount of fatty acid biosynthesis, steroid biosynthesis will use lots of NADPH i.e., liver, adrenal cortex, testis, and lactating mammary gland. These tissues have high level of PPP enzymes. The rapidly proliferating cells also have high level of PPP enzymes since they require a lot of Ribose 5-P for nucleic acids synthesis. PPP pathway has 2 phases 1. Oxidative irreversible reactions*: the conversion of Glucose 6-Phosphate into 6- phosphogluconolactone by glucose-6-phosphate dehydrogenase (G6PD)***, this step release NADPH and generate ribulose-5-phosphate. The NADPH is potent negative regulator for this enzyme. High NADPH (product) inhibits G6PD. In contrast, high NADP+ stimulate the enzyme. 2. Non-oxidative reversible reactions: the conversion of ribulose-5-phosphate to either ribose-5- phosphate (R5P) for nucleotide synthesis or glyceraldehyde-3-phosphate (GAP) and fructose- 6-phosphate (F6-P) for glycolytic intermediates (depending on the needs of the cells), using multiple enzymes such as epimerase, isomerase, transaldolase, transketolase. Uses of NADPH*** NADPH is used in several aspects including reductive biosynthesis, anti-oxidation (removal of ROS), defense mechanisms (antibacterial) and synthesis of NO. NADPH is a high energy molecule in biosynthesis of fatty acids. It will incorporate into the molecule being synthesized i.e., palmitate (which use 14 NADPH). Reactive Oxygen Species (ROS) Reactive oxygen species (ROS) is one of the radicals (atom that has 1 or more unpaired electrons), most concerned in biological system due to its high chemical reactivity. In the last step of electron transport chain, O2 receive H+, electron (e–), becoming water (H2O) and generate ATP. However, sometime O2 receive only electron and become superoxide (O2-) à H2O2 à hydroxyl radical (OH-). These ROS molecules cause damage to the cells by damaging all macromolecules including lipid, proteins, and nucleic acids. Mechanism for protecting cells against radicals (anti-oxidant mechanism) - Superoxide dismutase (SOD) enzyme converts superoxide (O2-) into H2O2. Then, H2O2 is degraded to become H2O and oxygen by enzyme catalase (found in peroxisome). PBBS508 Biochemistry and Genetics Lecture Note 2024 - Glutathione peroxidase***, enzyme reduces H2O2 to become O2. This enzyme required reduced glutathione (G-SH) for the reaction. To produce the reduced form of glutathione (G-SH), the enzyme glutathione reductase is involved, which requires NADPH for the reaction. This mechanism is very important for the anti-oxidant mechanism in red blood cells (RBCs). Glucose-6-phosphate dehydrogenase (G6PD) deficiency*** is an X linked recessive hereditary disease, causing hemolytic anemia, because red blood cells cannot detoxify ROS. The deficiency in G6PD reduces capacity of NADPH production, which is essential for keep glutathione (G-SH) in the reduced form. In RBCs, the reduced glutathione (G-SH) is used to get rid of H2O2 by glutathione peroxidase. Therefore, H2O2 causes damages to RBCs (hemoglobin denaturation causes Heinz body and membrane damage leads to hemolytic anemia). Splenic macrophages try to remove this hemoglobin aggregation in the red blood cells producing “bite cells” in blood smear test. G6PD deficiency patients usually do not develop any symptoms unless they are exposed with the triggers because these triggers induce high levels of ROS. The most common triggers include severe infection* or receiving oxidant drugs* i.e., antibiotics (sulfa), antimalarials (quinone)*, and some antipyretics. Fava bean (favism) ingestion can also trigger the symptoms in some variants of G6PD deficiency. NADPH in defense against bacterial infections Neutrophils and monocytes (phagocytic cells) are working as a defense system to get rid of bacterial infections by using both oxygen independent and oxygen dependent systems. Once the bacteria bind to the IgG receptor on the cells, it will be phagocytosed and fused with lysosome. In the oxygen dependent systems, O2 will be changed to superoxide by NADPH oxidase using NADPH. Superoxide can then spontaneously, or use SOD enzyme to convert to H2O2 , both superoxide and H2O2. can kill bacteria. H2O2. can also be converted to hypochlorous acid (a major component of bleach), using myeloperoxidase (MPO) enzyme for fighting with those infected bacteria. The defect in NADPH production, NADPH oxidase* or MPO system* can cause chronic granulomatous disease (CGD)*, the failure of phagocytic cells to kill infected organisms. Patients will suffer from recurrent bouts of infection. Most of patients with CGD will be diagnosed usually before age 5 and antibiotics can be given to them, before the infection occur. NADPH in nitric oxide (NO) production NO is the molecule serve as neurotransmitter, blood coagulation, and vasodilator. NO is produced by nitric oxide synthase (NOS) enzyme, which is an NADPH dependent enzyme.