Carbohydrate Metabolism Lecture 1 PDF
Document Details
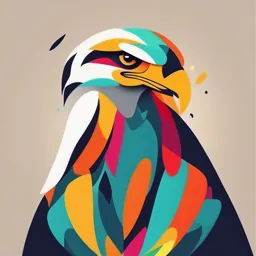
Uploaded by ConsistentIvory7857
Minia University
Dr. Asmaa Mostafa Bayoumi
Tags
Related
- Carbohydrate Metabolism (Lecture 3) PDF
- Lecture #1 Carbohydrates Metabolism (Glycolysis) PDF
- Lecture Notes on Metabolism of Carbohydrates (MED 302) PDF
- PC30103 Biochemistry of Sports Lecture 3 - Carbohydrate Metabolism II PDF
- Carbohydrate Metabolism PDF
- NFNF1613 Carbohydrate Metabolism I 2024-2025 PDF
Summary
This document is a lecture presentation on carbohydrate metabolism, specifically focusing on glycolysis. The lecture, delivered by Dr. Asmaa Mostafa Bayoumi at Minia University, provides a detailed explanation of the topic including different types of biomolecules, their roles, and processes. The lecture material explores the chemical reactions and various aspects of the topic.
Full Transcript
Carbohydrate Metabolism Lecture 1 GLYCOLYSIS By: Dr. Asmaa Mostafa Bayoumi Associate Professor, Department of Biochemistry, Faculty of Pharmacy, Minia University Reference Book: Biochemistry: The Molecular Basis of Life, (McKee&McK...
Carbohydrate Metabolism Lecture 1 GLYCOLYSIS By: Dr. Asmaa Mostafa Bayoumi Associate Professor, Department of Biochemistry, Faculty of Pharmacy, Minia University Reference Book: Biochemistry: The Molecular Basis of Life, (McKee&McKee) Third Edition What is life? Life is usually described in operational terms, as movement, reproduction, adaptation, and responsiveness to external stimuli. Since the end of the nineteenth century, however, the science of Biochemistry has provided new insights. Biochemists have investigated living organisms with a unique experimental approach. Their work has revealed that despite the rich diversity of living organisms from the blue whale to the smallest of microorganisms, all obey the same chemical and physical laws that rule the universe. Among the most important insights gained from the work of biochemists are the following: 1. Life is complex and dynamic. 2. Life is organized and self-sustaining. Living organisms are hierarchically organized systems. Each level is based on the one below. Certain biomolecules become linked to form polymers called macromolecules. Examples include nucleic acids, proteins, and polysaccharides, which are formed from nucleotides, amino acids, and sugars, respectively. Various combinations of biomolecules and macromolecules form more complex supramolecular structures that together make up cells. In multicellular organisms other levels of organization include tissues, organs, and organ systems. 3. Life is cellular. 4. Life is information-based. Biological information is in the form of coded messages that are inherent in the unique three-dimensional structure of biomolecules. Genetic information, which is stored in the DNA, called genes, in turn specifies the linear sequence of amino acids in proteins, and how and when those proteins are synthesized. The unique three dimensional structure of each type of protein allows it to bind to and interact with a specific type of molecule with a precise complementary shape. Information is transferred during the binding process. 5. Life adapts and evolves. Stress-induced DNA modifications and errors that occur when DNA molecules are copied can result in mutations. Living organisms are hierarchically organized systems Major Classes of Biomolecules 1. AMINO ACIDS AND PROTEINS. 2. SUGARS AND CARBOHYDRATES. 3. FATTY ACIDS. 4. NUCLEOTIOES AND NUCLEIC ACIDS. AMINO ACIDS AND PROTEINS. Amino acids are classified α, β, or γ according to the location of the amino group in reference to the carboxyl group. In α-amino acids, the amino group is attached to the α-carbon immediately adjacent to the carboxyl group. Also attached to the α-carbon is another group, referred to as the side chain or R group. The chemical properties of each amino acid are determined largely by the properties of its side chain. There are 20 standard α-amino acids that occur in proteins. Amino acid molecules are used primarily in the synthesis of long, complex polymers known as polypeptides. Short polypeptides, up to a length of 50 amino acids, are called peptides. Longer polypeptides are often referred to as proteins. Polypeptides play a variety of roles in living organisms. Examples of molecules composed of polypeptides include transport proteins, structural proteins, and the enzymes. SUGARS AND CARBOHYDRATES. Sugars that possess an aldehyde group are called aldoses and those that possess a ketone group are called ketoses. For example, glucose is an aldohexose. Fructose (fruit sugar) is a ketohexose. Carbohydrates range from the monosaccharides, such as glucose, to the polysaccharides, polymers that contain thousands of sugar units. Examples of the latter include starch and cellulose in plants and glycogen in animals. Glucose is the principal carbohydrate energy source in animals and plants. Sucrose is used by many plants as an efficient means of transporting energy throughout their tissues. Some carbohydrates serve as structural materials. Cellulose is the major structural component of wood and certain plant fibers. Chitin, another type of polysaccharide, is found in the protective outer coverings of insects. Some biomolecules contain carbohydrate components. Nucleotides, the building block molecules of the nucleic acids, contain either of the sugars ribose or deoxyribose. Certain proteins and lipids also contain carbohydrate. Glycoproteins and glycolipids occur on the external surface of cell membranes in multicellular organisms, where they play critical roles in the interactions between cells. FATTY ACIDS. Fatty acids are monocarboxylic acids that usually contain an even number of carbon atoms. In some organisms they serve as energy sources. There are two types of fatty acids: saturated fatty acids, which contain no carbon-carbon double bonds, and unsaturated fatty acids, which have one or more double bonds. Although the charged carboxyl group has an affinity for water, the long nonpolar hydrocarbon chains render most fatty acids insoluble in water. Fatty acids occur as independent (free) molecules in only trace amounts in living organisms. Most often they are components of several types of lipid molecules. Lipids are a diverse group of substances that are soluble in organic solvents such as chloroform or acetone, but are not soluble in water. For example, triacylglycerols (fats and oils) are esters containing glycerol and three fatty acids. Certain lipid molecules that resemble triacylglycerols, called phosphoglycerides, contain two fatty acids. In these molecules the third hydroxyl group of glycerol is coupled with phosphate, which is in tum attached to small polar compounds such as choline. Phosphoglycerides are an important structural component of cell membranes. NUCLEOTIOES AND NUCLEIC ACIDS. The bases in nucleotides are heterocyclic aromatic rings with a variety of substituents. There are two classes of base: the bicyclic purines and the monocyclic pyrimidines. Each nucleotide contains three components: a five-carbon sugar (either ribose or deoxyribose), a nitrogenous base, and one or more phosphate groups. An example: adenosine triphosphate contains a nitrogenous base (adenine), a pentose sugar (ribose), and three phosphates. Nucleotides participate in a wide variety of biosynthetic and energy-generating reactions. For example, a substantial proportion of the energy obtained from food molecules is used to form the high-energy phosphate bonds of adenosine triphosphate (ATP). The most important role of nucleotides, however, is their role as the building block molecules of the nucleic acids. In a nucleic acid molecule, large numbers of nucleotides are linked by phosphodiester linkages to form long polynucleotide chains or strands. There are two types of nucleic acid: 1. DNA. DNA is the repository of genetic information. Its structure consists of two polynucleotide strands wound around each other to form a righthanded double helix. In addition to the pentose sugar deoxyribose and phosphate, DNA contains four types of base: the purines (adenine and guanine) and the pyrimidines (thymine and cytosine). The double helix forms because of complementary pairing between the bases made possible by the formation of hydrogen bonds. Adenine pairs with thymine and guanine pairs with cytosine. Each gene is composed of a specific and unique linear sequence of bases. Most genes code for the linear sequence of amino acids in proteins. 2. RNA. RNA differs from DNA in that it contains the sugar ribose instead of deoxyribose, and the base uracil instead of thymine. RNA is single stranded. RNA molecules fold into complex three-dimensional structures created by local regions of complementary base pairing. During a complex process the DNA double helix partially unwinds and RNA molecules are synthesized using one DNA strand as a template. There are three major types of RNA: messenger RNA (mRNA), ribosomal RNA (rRNA), and transfer RNA (tRNA). Each unique sequence or molecule of mRNA possesses the information that codes for the amino acid sequence in a specific polypeptide. Ribosomes, the large, complex, supramolecular structures composed of rRNA and protein molecules, convert the mRNA base sequence into the amino acid sequence of a polypeptide. Transfer RNA molecules function as adapters during protein synthesis. Each type of tRNA molecule bonds to a specific amino acid. Each polypeptide is manufactured as the base sequence information in mRNA is translated by a ribosome as base pairing occurs between the mRNA and tRNA molecules. As the amino acids are brought into close proximity, peptide bonds are formed. Carbohydrate Metabolism Glycolysis A series of reactions that occurs in almost every living cell. An anaerobic process. Referred to as the “Embden-Meyerhof-Parnas” pathway. Each glucose molecule is converted to two three-carbon units (pyruvate). The small amount of energy captured during glycolytic reactions is stored temporarily in two molecules each of ATP and NADH. In anaerobic organisms, pyruvate may be converted to waste products e.g. ethanol, lactic acid, acetic acid. Aerobic organisms such as animals and plants completely oxidize pyruvate to form CO2 and H2O in aerobic respiration. The glycolytic pathway can be summed up in the following equation: D-Glucose + 2 ADP + 2 Pi + 2 NAD+ → 2 pyruvate + 2 ATP + 2 NADH + 2 H+ + 2 H2O Glycolysis 1. Synthesis of glucose-6-phosphate. An irreversible reaction. One ATP molecule is consumed. The animal liver has four hexokinases: Three of these enzymes (hexokinases A, B, C) have high affinities for glucose relative to its concentration in blood (i.e., they are half-saturated at concentrations of less than 0.1 mM, although blood glucose levels are approximately 4-5 mM). These enzymes are inhibited by glucose-6-phosphate, the product of the reaction. They are not affected by insulin. When blood glucose levels are low, these properties allow cells such as those in brain and muscle to obtain sufficient glucose. When blood glucose levels are high, cells do not phosphorylate more glucose molecules than required to meet their immediate needs. The fourth enzyme, called hexokinase D (or glucokinase), has different kinetic properties that permit the liver to divert glucose into storage as glycogen. Glucokinase requires much higher glucose concentrations for optimal activity (about 10 mM), and it is not inhibited by glucose-6-phosphate. It is stimulated by insulin. Consequently, after a carbohydrate meal the liver does not remove large quantities of glucose from the blood for glycogen synthesis until other tissues have satisfied their requirements for this molecule. 2. Conversion of glucose-6-phosphate to fructose-6-phosphate. 3. The phosphorylation of fructose-6-phosphate. An irreversible reaction. One ATP molecule is consumed. 4. Cleavage of fructose-1,6-bisphosphate. 5. Interconversion of glyceraldehyde-3-phosphate and dihydroxyacetone phosphate. After this reaction, the original molecule of glucose has been converted to two molecules of Glyceraldehyde-3-P. 6. Oxidation of glyceraldehyde-3-phosphate. Two NADH molecules are produced. 7. Phosphoryl group transfer. An example of a substrate-level phosphorylation. Two ATP molecules are produced because of the transfer of a phosphoryl group from a substrate with a high phosphoryl group transfer potential. The high-energy phosphoryl group is transferred from glycerate-1,3-bisphosphate to ADP. 8. The conversion of Glycerate-3-P to Glycerate-2-P. Glycerate-3-P Glycerate-2-P 9. Dehydration of Glycerate-2-P. 10. Synthesis of pyruvate. An irreversible reaction. Two ATP molecules are produced. Pyruvate Kinase Fates of Pyruvate In aerobic organisms, Acetyl-CoA is formed through oxidative decarboxylation. Acetyl-CoA is the entry-level substrate for the citric acid cycle. Citric acid cycle completely oxidizes two carbons to CO2 and NADH. The electrons of NADH and FADH2 produced in the citric acid cycle are delivered to oxygen via the electron transport chain (ETC) to produce water. Coupled to this process is the generation of a proton gradient that drives the synthesis of ATP. Under anaerobic conditions, Further oxidation of pyruvate is impeded. A number of cells and organisms compensate by converting pyruvate to a more reduced organic compound and regenerating the NAD+ required for glycolysis to continue. This process of NAD+ regeneration is referred to as fermentation. Muscle cells and certain bacterial species (e.g., Lactobacillus) produce NAD+ by transforming pyruvate into lactate: Fermentation In rapidly contracting muscle cells the demand for energy is high. After the O2 supply is depleted, lactic acid fermentation provides sufficient NAD+ to allow glycolysis (with its low level of ATP production) to continue for a short time. Microorganisms that use lactic acid fermentation to generate energy can be separated into two groups. Homolactic fermenters produce only lactate. For example, several species of lactic acid bacteria sour milk. Heterolactic or mixed acid fermenters produce several organic acids. Mixed acid fermentation, for example, occurs in the rumen of cattle. Symbiotic organisms, some of which digest cellulose, synthesize organic acids (e.g., lactic, acetic, propionic, and butyric acids). The organic acids are absorbed from the rumen and used as nutrients. Gases such as methane and carbon dioxide are also produced. Alcoholic fermentation occurs in yeast and several bacterial species. In yeast, pyruvate is decarboxylated to form acetaldehyde, which is then reduced by NADH to form ethanol. Fates of Pyruvate Regulation of Glycolysis The rate at which the glycolytic pathway operates is controlled primarily by allosteric regulation of three irreversible enzymes: hexokinase, PFK-1, and pyruvate kinase. Allosteric effectors are molecules whose cellular concentrations are sensitive indicators of a cell's metabolic state. Excess glucose-6-phospbate inhibits hexokinase. High AMP concentration activates PFK-1 and pyruvate kinase. While high ATP concentration inhibits both enzymes. Citrate and acetyl-CoA, which accumulate when ATP is in much supply, inhibit PFK-1 and pyruvate kinase, respectively. Fructose-2,6-bisphosphate, produced via hormone-induced covalent modification of PFK-2, is an indicator of high levels of available glucose and allosterically activates PFK-1. Fructose-1,6-bisphosphate activates pyruvate kinase, providing a feedforward mechanism of control. Glucagon, present when serum glucose is low, activates the phosphatase function of PFK-2, reducing the level of fructose-2,6-bisphosphate in the cell, thus, inhibits glycolysis. Insulin, present when serum glucose is high, activates the kinase function of PFK-2, increasing the level of fructose-2,6-bisphosphate in the cell, thus, activates glycolysis. The regulation of glycolysis is summarized in the following Table.