Biochem 4.4 Enzyme Activity PDF
Document Details
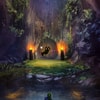
Uploaded by iiScholar
Arizona State University
Tags
Summary
This document discusses enzyme activity, its measurement, and calculating specific activity. It describes protein purification methods as well as factors that influence enzyme activity. The text explains how enzyme activity can be measured and calculated.
Full Transcript
erent compartments within a cell or organism can have slightly different environments. These environments have important implications for enzyme activity. Pathological conditions may also alter an enzyme\'s environment and therefore its activity.4.4.01 Measuring Enzyme ActivityEnzymes are biological...
erent compartments within a cell or organism can have slightly different environments. These environments have important implications for enzyme activity. Pathological conditions may also alter an enzyme\'s environment and therefore its activity.4.4.01 Measuring Enzyme ActivityEnzymes are biological catalysts that accelerate reactions to a rate necessary to sustain life. Therefore, the measurement of an enzyme\'s activity is, in theory, straightforward. An enzyme\'s activity can be measured as the amount of product produced per unit time. Alternatively, activity can be defined as the amount of substrate consumed per unit time. This measurement is often given in units of μmol/min, where 1 μmol/min = 1 enzyme unit (U), but the definition of an enzyme unit may vary depending on the system being studied.One potential hurdle in the measurement of enzyme activity is that the rate depends on the amount of enzyme in the sample. In other words, enzyme activity is an and varies with enzyme concentration. If not accounted for, this may result in misinterpretation of data.For example, consider an experiment in which samples of two different isoforms of an enzyme are prepared. The experimenter measures their activity and tries to compare the enzymes\' catalytic abilities. Even if equal volumes are tested, a higher concentration of the slower enzyme may cause it to appear to have a higher activity than the faster enzyme, leading to an incorrect conclusion (Figure 4.58). Chapter 4: Enzyme Activity188Figure 4.58 Enzyme activity calculations for samples of different enzymes with different concentrations.Differences in enzyme concentration can come from many sources. These differences may be due to differences in expression level, cell or tissue viability, sample preparation, sample collection, or other factors. To account for enzyme amount, the specific activity is typically reported.Specific activity is calculated by dividing the enzyme activity by the mass of protein in the sample, and it is commonly reported in units of μmol∙min−1∙mg protein−1, or simply U/mg protein. This calculation makes specific activity an of the protein mixture.Specific activity=Enzyme activityMass of protein=μmol/minmg proteinNote that \"mg protein\" appears in the denominator of the specific activity equation rather than mol protein. Most protein quantitation assays report mass of protein. Therefore, dividing by \"mg\" simplifies the calculation and avoids any discrepancies between expected and actual molar mass (eg, due to , , or formation of protein complexes).In addition, the conversion of mass to moles would only be appropriate if the entire protein mass in the sample were pure enzyme. It is not appropriate if there are any proteins other than the enzyme of interest, and samples usually contain other proteins, even after purification. By dividing by total protein mass, specific activity measurements can be calculated independent of enzyme purity. Furthermore, once calculated, specific activity can also be used to track increasing purity during a purification procedure.Protein purification techniques are covered in detail in Chapter 14. As a brief overview, protein purification begins with the creation of a crude lysate, which is a sample of tissue or cells that have been lysed (ie, ruptured). In the crude lysate, all soluble proteins from the cytosol have been released to Chapter 4: Enzyme Activity189diffuse freely in the solution. The crude lysate contains not only the protein or enzyme of interest but also many other proteins and enzymes that the cells use to maintain life. The goal of a purification project is to remove unwanted, contaminating proteins while retaining as much of the protein of interest as possible (Figure 4.59).Figure 4.59 Overview of a protein purification process.Within a purification procedure, the crude lysate contains the maximum amount of the enzyme of interest. Therefore, the crude lysate typically has the highest total activity of any purification step. Because some enzyme of interest is inevitably removed by purification steps, the total activity for each subsequent step typically decreases. However, crude lysate also contains the maximum number of contaminants. At this stage, the denominator of the activity equation (mg protein) is large, but the contaminating protein does not contribute to enzyme activity. Therefore, dividing by a large total amount of protein yields a low specific activity.The contaminants are removed in subsequent purification steps while most of the enzyme of interest remains. The result is that the enzyme of interest forms a higher percentage of the total protein mass. Therefore, purification results in an increased specific activity.Table 4.6 shows example measurements from a protein purification procedure. The values in white boxes can be measured directly by the experimenter. From these data, the values in shaded boxes can be calculated. Chapter 4: Enzyme Activity190Table 4.6 Purification table showing values collected and calculated for a sample purification.Total protein mass is the mass of all proteins in the whole volume collected. It is calculated by multiplying the volume by the measured protein concentration in mg/mL. Similarly, total activity is calculated by multiplying the volume by the measured activity per mL. Note that the units for volume in the numerator and denominator must cancel to provide a correct value.Total protein mass (mg)=Volume (mL)×Concentration mgmLTotal activity (U)=Volume (mL)×Activity per mL UmLThe example data for total protein mass in Table 4.6 show how total protein mass decreases after each step of the purification.Specific activity is calculated by dividing enzyme activity by protein mass. From the example data shown in Table 4.6, specific activity can be calculated in two ways. The first way is to divide total activity by the total protein mass.Specific activity Umg=Total activity (U) Total protein mass (mg)The second way is to divide the activity per volume by the concentration. Again, the units for volume must cancel out to provide a correct answer.Specific activity Umg=Activity per mL UmL Protein concentration mgmL Chapter 4: Enzyme Activity191The example data also demonstrate how specific activity increases with each purification step. To track purity over a purification procedure, the fold-increase in purity is calculated by dividing the specific activity of a given step by the specific activity of the crude lysate.Fold-increase in purity=Specific activity UmgSpecific activity of crude lysate UmgFor example, Table 4.6 shows that size-exclusion chromatography increased the purity 11-fold 760 Umg71 Umg.The yield of a protein purification can be thought of as the amount of enzyme recovered divided by the total amount of enzyme originally present.% Yield=Total activityTotal activity of crude lysateTotal activity represents the amount of the enzyme of interest. The total activity of the crude lysate is therefore used as the reference for enzyme originally present. Because there is typically at least some loss of correctly folded, functional enzyme at each purification step, each purification step typically increases purity and specific activity but decreases yield.Concept Check 4.14A researcher induced expression of the enzyme glycogen synthase in two separate cultures: one culture of Escherichia coli and one culture of Saccharomyces cerevisiae. Each culture was lysed, and the lysates were each purified by identical methods. Each lysate yielded 5 mL of purified enzyme, and enzyme activity in each was immediately measured by monitoring UDP-glucose consumption.Which sample shows higher activity? What can be said about each enzyme\'s specific activity? Chapter 4: Enzyme Activity192Concept Check 4.15Information about the purification of glycogen synthase from E. coli is given in the following table.Purification stepVolume collected (mL)Protein concentration (mg/mL)Activity per mL (U/mL)Crude lysate500.1.020.After affinity chromatography10.5.0750What is the fold-increase in purity of glycogen synthase? What is the percent yield of glycogen synthase?4.4.02 Environmental Conditions for Enzyme ActivityThe activity of an enzyme sample depends not only on the enzyme\'s purity but also on its environmental conditions. For example, altering the pH, salt concentration, or temperature of an enzyme preparation can have drastic effects on the enzyme\'s specific activity. Lesson 2.3 discussed how these conditions can induce protein. Like all proteins, enzymes can denature, and they are also affected by their environment in additional ways. The result is that each enzyme shows peak activity within a narrow range of pH, salt concentration, and temperature. The specific values of this range depend on the identity of each enzyme and constitute that enzyme\'s optimal conditions.pH and Salt ConcentrationAs discussed in Concepts 2.2.03 and 2.2.04, salt bridges play an important role in stabilizing a protein\'s tertiary and (if present) quaternary structure. Figure 4.60 shows how alteration of pH can affect the protonation state of the residues forming those salt bridges, which disrupts them and can denature the protein. In addition, changes in salt concentration can disrupt salt bridges because the ionized residues may interact with dissolved salt instead of with their appropriate partner residue. Chapter 4: Enzyme Activity193Figure 4.60 pH and salt concentration may lower enzyme activity through disruption of salt bridges, resulting in enzyme denaturation.In addition to inducing denaturation, altering the pH or salt concentration can also directly affect the enzyme\'s catalytic mechanism. As discussed in Concept 4.3.05, several modes of catalysis depend on the ionization of active site residues (eg, acid-base catalysis, covalent catalysis, general enzyme-substrate interactions). Although the enzyme active site can alter amino acid pKa values, the residues are still affected by the surrounding solution\'s proton and salt concentrations. Changes in these conditions may adversely affect acid-base equilibrium, nucleophilic ability, or substrate binding in the active site.Because of the effects of pH and salt concentration on enzyme stability, denaturation, and catalytic mechanism, enzymes have evolved to show optimal activity within only a small range. Changes toward either side (too low or too high) can disrupt residue ionization state or correct salt bridge formation, leading to decreased enzyme activity (Figure 4.61). Chapter 4: Enzyme Activity194Figure 4.61 The effects of pH (A) and salt level (B) on enzyme activity.TemperatureEnzymes also have an optimal temperature at which they are most active, as shown in Figure 4.62. Concept 2.3.04 discusses how high temperatures cause proteins to denature and lose their function. Therefore, temperatures above the optimal temperature cause enzyme activity to decrease. Chapter 4: Enzyme Activity195Lower temperatures tend to stabilize proteins and enzymes in their native conformations. However, below-optimal temperatures decrease enzyme activity by decreasing the average energy of the molecules. At , fewer molecules can reach the activation energy Ea.Figure 4.62 The effect of temperature on enzyme activity.Different Enzymes Have Different Optimal ConditionsAll enzymes have their own optimal pH, salt concentration, and temperature. The optimal conditions for any given enzyme will likely differ from those for another enzyme, even if they are from the same organism. Although the term \"physiological conditions\" typically refers to a well-controlled set of standard biochemical conditions (eg, pH 7.4, 37 °C), organisms often have subcompartments in which conditions differ (Figure 4.63).For example, the cells that line the stomach have a cytosolic pH of 7.4, typical of most mammalian cells. Consequently, their cytosolic proteins likely have an optimal pH at or near 7.4. Conversely, the interior of the stomach is highly acidic. Therefore, the proteins and enzymes that are secreted into the stomach have optimal pH values around 2.0.Similarly, different intracellular organelles have pH values that differ from the cytosol. For example, lysosomes are acidic whereas the mitochondrial matrix is basic. Consequently, the proteins and enzymes found in those spaces have evolved an optimal pH to match their environment. Chapter 4: Enzyme Activity196Figure 4.63 Bodies and cells contain subcompartments that maintain differing environmental conditions.Concept Check 4.16Thermophiles are organisms that thrive at extreme temperatures (ie, 110--121 °C). In contrast, mesophiles thrive at moderate temperatures (ie, 20--45 °C).DNA polymerase samples from a thermophile and from a mesophile were purified and tested for activity at various temperatures, yielding the following graph. Which sample was isolated from the mesophile, and which was isolated from the thermophile? Chapter 4: Enzyme Activity197Pathological Conditions Adversely Affect Enzyme FunctionPathological conditions (ie, conditions resulting from an illness or other affliction) also affect conditions within the body and therefore affect enzyme function. For example, alkalosis and acidosis occur when pH values throughout the body become too basic or acidic, respectively. The fever response results in an increased body temperature above baseline (37 °C).These pathological conditions typically affect enzyme activity and create suboptimal conditions for enzyme function. Sometimes these condition changes may have beneficial side effects; however, large changes are generally harmful. For example, mild fevers may stress the germs infecting a host and assist the host\'s immune response. Prolonged or elevated fevers, however, adversely affect the host\'s enzymes and proteins, resulting in increased risk of damage.4.4.03 Cofactors and CoenzymesIn addition to being affected by environmental conditions such as pH, salt, and temperature, enzyme activity can also be affected by the presence or absence of specific molecules. The effect of substrate concentration on enzyme activity and enzyme rate is discussed in detail in Chapter 5. This concept focuses on the effects of cofactors and coenzymes.Concept 2.4.03 introduces cofactors as components that are not amino acids (eg, metal ions) but are required for protein function. Coenzymes are simply organic cofactors. Prosthetic groups are cofactors that are tightly joined to the protein, often by a covalent bond (Figure 4.64).Figure 4.64 Cofactors, coenzymes, and prosthetic groups are components of proteins that are not amino acids but are required for protein function.Cofactors, coenzymes, and prosthetic groups all play similar roles in enzymes. Cofactors can serve structural purposes and help maintain the enzyme\'s native conformation, or they can act directly in the catalytic mechanism. For example, metal ions such as Mg2+ can help orient substrates in the correct position.By convention, some molecules are commonly called coenzymes but behave more like cosubstrates. For example, oxidoreductases can convert redox coenzymes such as NAD+, NADP+, and FAD from their oxidized forms to their reduced forms (Concept 4.2.02). Despite existing in a changed form at the end of the reaction, these molecules are often called coenzymes because they are typically regenerated to their original form by other enzymes. Chapter 4: Enzyme Activity198Dietary Vitamins and Minerals Are a Source of Enzyme Cofactors and CoenzymesThe human body cannot synthesize most coenzymes and cofactors from a diet of just carbohydrates, protein, and fat. Therefore, cofactors and coenzyme precursors must be included in the diet as well. Dietary cofactors and coenzyme precursors are known as minerals and vitamins, respectively. An inability to consume enough dietary vitamins and minerals can lead to a loss of enzyme function and adverse health outcomes (Figure 4.65).Figure 4.65 Minerals and vitamins are essential to the diet as they are used as cofactors and coenzyme precursors, respectively.