Biochemistry Lesson 6.2: Enzyme Regulation PDF
Document Details
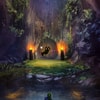
Uploaded by iiScholar
Arizona State University
Tags
Summary
This document details enzyme regulation, specifically focusing on covalent modifications. It explores zymogens and the dynamic process of phosphorylation as mechanisms to control enzyme activity.
Full Transcript
# Chapter 6: Enzyme Regulation ## Lesson 6.2: Regulation by Covalent Modifications ### Introduction In addition to allosteric regulation, enzymes are commonly regulated by covalent modifications. Lesson 2.4 discussed many post-translational modifications that a protein may undergo. This section d...
# Chapter 6: Enzyme Regulation ## Lesson 6.2: Regulation by Covalent Modifications ### Introduction In addition to allosteric regulation, enzymes are commonly regulated by covalent modifications. Lesson 2.4 discussed many post-translational modifications that a protein may undergo. This section discusses the effects of those modifications on enzyme activity specifically. ### 6.2.01 Zymogens Proproteins are proteins that are synthesized in an inactive form and then converted to an active form by proteolytic cleavage. For example, proinsulin is cleaved to form the active hormone insulin, and fibrinogen is cleaved to form the active clotting factor fibrin. Zymogens are proproteins that generate an enzyme upon cleavage. Zymogens are also known as proenzymes. Pepsinogen is an example of a subclass of a zymogen. Specifically, pepsinogen can proteolytically cleave itself under certain conditions, and this cleavage irreversibly converts it into its active form, pepsin. **Image Description**: A diagram showing inactive pepsinogen being cleaved into active pepsin. The cleavage happens between the "zymogen" portion and the "active enzyme" portion. Pepsinogen is shown as a folded structure with a "zymogen" label on the left hand side, and a "cleaved off peptide" label on the right. Pepsin is shown as a structure with an "exposed active site" at the right handside and a "active enzyme" label below it. An arrow points from pepsinogen to pepsin with the label "HCI or pepsin (proteolysis)" written on it. This diagram shows the irreversible conversion of a zymogen into its active form. ### 6.2.02 Dynamic Covalent Modifications Irreversible inhibitors can stop an enzyme's catalytic activity by covalently modifying an individual molecule, and most of these inhibitors are exogenous to the organism. Lesson 6.2.01 introduces endogenous covalent modification of zymogens through proteolysis, which irreversibly activates the molecule to form an active enzyme. Both irreversible inhibition and zymogen activation are examples of post-translational modifications - covalent modifications that occur on a peptide after the sequence has been accurately translated by a ribosome. Although many post-translational modifications (PTMs) are irreversible, this section focuses instead on covalent PTMs that are dynamic - these modifications can be added, but also removed, to dynamically regulate a target enzyme's activity. Note that sometimes dynamic covalent modification is referred to as reversible covalent modification. However, this does not refer to the thermodynamic reversibility of a single enzymatic reaction. Instead, it refers to the ability to undo a covalent modification through the action of a separate enzymatic reaction. **Image Description**: A diagram showing different types of post-translational modifications of a protein. The top of the diagram shows a protein molecule, and below it are several types of chemical groups that can be attached to the protein. The labels below the chemical groups identify each modification: Lipidation, Ubiquitination, Glycosylation, Phosphorylation, and Proteolysis. The right of the diagram includes a key that defines what each chemical group symbol represents. ### Phosphorylation Phosphorylation is one of the most common regulatory dynamic PTMs. As discussed in concept 2.4.02, phosphorylation is the addition of a phosphate group to a protein, typically through the side chain hydroxyl of a serine, threonine, or sometimes tyrosine residue. The addition of the phosphate group is most commonly mediated by a kinase enzyme through transfer of the γ-phosphate of ATP. The phosphate group is most commonly removed by hydrolysis using a phosphatase enzyme. Although both kinase and phosphatase reactions are considered thermodynamically irreversible when considered individually, they can work together to control the reversible phosphorylation status of a target protein. **Image Description**: A diagram showing the interaction of kinase and phosphatase to regulate the phosphorylation state of an enzyme. The diagram depicts a molecule of ATP, the molecule that provides the phosphate group to the enzyme, and a second molecule of ATP that is being created from ADP in the presence of a phosphate group. In between the two ATP molecules, a kinase enzyme is shown adding a phosphate group to a substrate. The presence of a phosphatase enzyme then results in the removal of the phosphate group, generating H2O as a byproduct. The arrows point to the direction of substrate conversion from dephosphorylated to phosphorylated and vice versa. ### Phosphorylation and Enzyme Activity Because enzymes are proteins, the phosphorylation status of an enzyme can also control its level of enzyme activity. The specific effect of a phosphorylation event depends on the enzyme being modified. Some enzymes are "switched on" when phosphorylated; others are "switched off" by phosphorylation. Some enzymes stay "on" regardless of phosphorylation status but are simply switched into a more active or less active state. Many enzymes have multiple phosphorylation sites, and phosphorylation at one site may have a different or opposing effect to phosphorylation at a different site. Mechanistically, phosphorylation affects an enzyme's activity because the modification induces a conformational change in the enzyme. This conformational change may affect the enzyme's turnover number, its ability to bind its substrate, or both. An enzyme is turned "on" if its catalytic efficiency rises, and an enzyme is turned "off" if its catalytic efficiency drops to nearly zero. **Image Description**: A diagram showing the regulation of glycogen metabolism via phosphorylation. The diagram shows two enzymes that control glycogen metabolism: glycogen phosphorylase and glycogen synthase. Phosphorylation activates glycogen phosphorylase, which breaks down glycogen into glucose. Phosphorylation inactivates glycogen synthase, which synthesizes glycogen from glucose molecules. The diagram shows the following: * Glycogen breakdown - when inactive glycogen phosphorylase is phosphorylated (by kinase) it becomes an active glycogen phosphorylase that breaks down glycogen. Dephosphorylation (by phosphatase) reverses this into an inactive form again. * Glycogen synthesis - when glycogen synthase is phosphorylated (by kinase) it becomes inactive. Dephosphorylation (by phosphatase) reverses this into an active form again. ### Advantages of Covalent Modification Covalent regulation of enzymes offers an alternate means of regulating enzymes that supplements feedback mechanisms and other allosteric mechanisms. The unique features of covalent modification allow this type of regulation to either enhance or override allosteric signaling to offer greater control over the metabolic activity of a cell or organism. Concept 3.2.02 explains that signal cascades can propagate a cellular response. Changes in phosphorylation status often play a role in the ability of these cascades to amplify the original signal. This is because each kinase or phosphatase involved can act on multiple target substrates. For example, suppose one molecule of Kinase A phosphorylates and activates 10 molecules of Phosphatase B. Each Phosphatase B can then dephosphorylate and activate 10 molecules of Kinase C, resulting in 100 molecules of active Kinase C from a single activated Kinase A. In contrast, a single feedback or allosteric modulator can affect only a single enzyme and only when the modulator is bound to the enzyme. **Image Description**: This diagram compares the signal amplification of a feedback/allosteric effector and covalent modification of a kinase and phosphatase signal cascade. The top of the diagram shows a feedback/allosteric effector binding to a single Kinase C and activating it. This represents a smaller cellular response as there is no signal amplification. The bottom of the diagram shows a more complex signal cascade: A single active Kinase A activates 10 molecules of Phosphatase B which in turn activates 10 molecules of Kinase C each, resulting in 100 total active Kinase C molecules. This represents a greater cellular response as the signal is amplified through the cascade. ### Overriding Feedback Control Covalent regulation of enzymes can be used to override feedback inhibition of enzymes. **Image Description**: This diagram shows the use of covalent regulation to override feedback inhibition of enzymes. The diagram shows the process of glycolysis where ATP is produced. Typically, high levels of ATP would inhibit the process via negative feedback regulation. When glucose levels are high, and cells are required to metabolize glucose quickly, phosphatases are activated to prevent the feedback inhibition. This allows for glycolysis to proceed at a greater rate and increase production of ATP. ### Other Dynamic Covalent Modifications Although this chapter has focused primarily on phosphorylation, many other types of dynamic covalent regulation of enzymes exist as discussed previously. These modifications work in a similar way as phosphorylation - they cause conformational changes that affect an enzyme’s catalytic efficiency to turn an enzyme "on" or "off" or to fine-tune their level of activity.