BCH210 Section 2 Lecture Notes PDF
Document Details
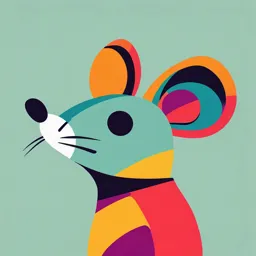
Uploaded by PremierForethought3427
University of Toronto
Sian Patterson
Tags
Summary
These lecture notes cover the structure and function of biological membranes, focusing on the roles of lipids and proteins. They include details on membrane properties like fluidity and asymmetry, as well as discussions on membrane protein function and different types of transport mechanisms. The notes also include sections on lipid metabolism and cholesterol.
Full Transcript
Glossary: https://utoronto-my.sharepoint.com/:x:/g/personal/sian_patterson_utoronto_ca/EVWadRQ UvKVKlVxzX8kGkhUBhHyLwrUe8ksZLLB12nTY-A?e=5kTcfV Metabolism Map: Metabolism Map.pdf 2023 Final: BCH210H.Fall.2023.Final.Exam.pdf 2014 Final: BCH210H.Fall.2023.Final.Exam.pdf DO ALL REVIEW SHEETS Look at l...
Glossary: https://utoronto-my.sharepoint.com/:x:/g/personal/sian_patterson_utoronto_ca/EVWadRQ UvKVKlVxzX8kGkhUBhHyLwrUe8ksZLLB12nTY-A?e=5kTcfV Metabolism Map: Metabolism Map.pdf 2023 Final: BCH210H.Fall.2023.Final.Exam.pdf 2014 Final: BCH210H.Fall.2023.Final.Exam.pdf DO ALL REVIEW SHEETS Look at learning goals Look at tutorials Membrane protein structure and function: done intro to metabolism: done carb metabolism: done mitochondrial bioenergetics: fat metabolism: metabolic conditions: Lecture 13: Membrane Structure - Essential membrane functions - Act as a barrier regulating the import and export of essential molecules - Compartementalization of specialized processes increase cellular efficiency - The cell membrane modulates cell-cell recognition due to the presence of glycoproteins and glycolipids - Signaling across the membrane is mediated by proteins and lipids - Structure is key for function - What is responsible for membrane formation - A decrease in ionic interactions with water - Membrane properties - Lipid bilayers that are impermeable to polar or charged molecules - Hydrophobic effect drives membrane formation of amphipathic molecules that assemble due to non-covalent interactions - Membranes are asymmetric and composed of lipids and proteins, which math or may not have carbohydrates covalently bound (PTM) - Membrane fluidity is important - Signals may also be transmitted across a membrane (hormones bind, conformational change) - Lipids and cholesterol also play a role in membrane fluidity - Measuring lipid dynamics in membranes (how fast do lipids and proteins diffuse laterally in a membrane) - FRAP: bleach fluorophore in membrane and measure mobility into affected region (rate=flourescence/time) - Lipid raft: molecules hang out there longer than usual - Single molecule tracking: microscope tracks molecule, if a protein is in the way it has to go around - Biological membranes are asymmetric - The two leaflets of the membrane have very different lipid and protein compositions - The addition of sugars to lipids and proteins is a form of PTM mediated by enzymes and is important for membrane insertion and cell recognition - The movement of lipids fro one leaflet to the other is very slow - Flip flop diffusion of polar/charged groups across the hydrophobic membrane interior is energetically unfavourable - Enzymes mediate membrane asymmetry - Flippase (ATP dependent): moves into the cell, moves PE and PS from outer to cytosolic leaflet, P-type ATPase - Floppase (ATP dependent): moves out of cell, moves phospholipids from cytosolic to outer leaflet, ABC transporter - Scramblase (ATP independent): moves lipids in either direction towards equilibrium, move al lipid down their concentration gradients to produce a symmetrical membrane - Lipids - Physical properties: low solubility in water, high solubility in non-polar solvents - Lipid self assembly and bilayer formation - Lipids spontaneously aggregate in water to bury their hydrophobic groups while polar groups interact with water - Non-covalent forces drive assembly - Fatty acids - Chains are either saturated (single bonds) or unsaturated (doouble bonds) - Double bonds are usually numbered relative to the carboxylic acid but can also be numbered relative to the terminal methyl group for omega fatty acids - They may either be free fatty acids or bound to a head group or backbone via ester bonds - Omega fatty acids in human health - Omega-3 and omega-6 fatty acids are polyunsaturated fatty acids and must be obatined from diet - Phase transitions - The melting of membrane lipids is a phase transition from a gel-like sloid phase to the liquid crystalline phase - The melting temperature (™) is an index of membrane fluidity - Triglycerides - 3 fatty acids tails bound to glycerol backbone through ester linkages - Very hydrophobic - For storing carbons to be used for energy - Membrane lipid diversity - In the membrane, amphipathic molecules are made by attaching fatty acyl chains to polar head groups - Glycero-phospho-lipids - Sphingolipids - Cholesterol is the most common steroid found in membranes - Cholesterol - Can be metabolized to other hormones and bile salts needed for dietary lipid absorption - Can form complexes with sphingolipids, glycolipids and some lipid-lipid anchored proteins to form lipid rafts - Lipid rafts help moderate membrane fluidity and play a role in signal transduction - Peripheral membrane proteins - Adhere to the surface of lipid membranes or integral membrane proteins through non-covalent interactions - Can be removed using milder conditions - Integral membrane proteins - Completely span the membrane - Require hash conditions for purification - Lipid anchored proteins - Also require harsh detergents or solvents for membrane removal - Lipid chains are covalently attached to amino acid functional groups and side chains - GPI-anchored proteins are covalently linked through a sugar chain and lipid anchor (usually at cell surface) - Studying membrane proteins - Detergents help form micelles around the hydrophobic regions of a membrane protein - The critical micelle concentration (CMC) is the concentration ay which the detergent spontaneously forms stable micelle structures Lecture 14: Membrane protein function - Cellular signaling - The binding of signaling molecules to their receptors initiate processes such as metabolic pathways and gene expression - Proteins are essential for carrying out this response but must be regulated - Signal transduction - Binding specifically to a signalling molecule in response to a physiological stimulus - Reception of the message by the receptor, usually an integral membrane protein - Relay of the primary message to the cell interior by the generation of an intracellular secondary messenger - Amplification and transduction of the signal - Response then termination of the signal (regulation) - G Protein Coupled Receptors - Contain 7 ™ segments - Conformational changes release the G proteins - Can bind many different ligands - Natural: serotonin, epinephrine, prostaglandins, dopamine - Characterizing binding interactions - Non-covalent interactions between the amino acid side chains and molecules functional groups influences the binding affinity - Binding affinities can be used to characterize and compare the non-covalent interactions between 2 biomolecules - Kd values are dissociation constants, a lower Kd equals stronger binding - Binding is saturable based on stoicheometry and reversible for non-covalent interactions - Beta 2 Adregergic receptor - Ligand = epinephrine - Ligand binding induces small changes in TM5 on the extracellular side - A 14 A (10^-10m) movement in TM6 transmits the signal inside - Major conformational changes in TM6 promote the release of Ga-GTp which activates adenyl cyclase to produce the secondary messenger cAMP - cAMP can activate PKA - Insulin (fat storage) and epiniephrine (fat breakdown) are competing hormones - Phosphorylation of the insulin receptor substrate (IRS)-1 and activation of the pathway also leads to phosphorylation of the B-adrenergic receptor by PKB - PTM leads to internalization and degradation, terminating GPCR signalling - Ras proteins - GTPases that bind and hydrolyze GTP (turns off signal) - Enzyme linked receptors - Usually contain a single transmembrane segment that may be homodimers or dimerize upon ligand binding - Activation leads to auto phosphorylation or phosphorylation by tyrosine kinases (PTM) - Phospholipid mediated signalling - Phospholipases hydrolyze phospolipids to produce other 2nd messengers like DAG or IP3 leading to the release of calcium from ER - Membrane transport - Integral membrane proteins are important for transport via facilitated diffusion and active transport with or without ATP - Facilitated diffusion is saturable - Facilitated transport is dependent on the presence of binding sites on membrane proteins - The rate of transport (v) is saturable at high substrate concentration - A hyperbolic curve is similar to what is seen for simple catalytic enzymes - Channel proteins - Membrane transporters that facilitate diffusion are also known as ion channel proteins - The structure of the membran protein is key for its function - Important features: - Selectivity, rapid conductance of ions, can be gated due to stimuli - Potassium ion channel - Each subunit contributes a selectivity filter of 5 amino acids that contribute to K+ binding Lecture 15: Intro to Metabolism - Bioenergetics and metabolism - Metabolism involves the catabolism of compounds, releasing free energy which can be used to generate ATP - Energy is used in anabolic reactions to synthesize larger macromolecules using simple building blocks - The complex metabolic map can be broken down into linear, cyclic or even branched pathways - Gibbs free energy changes (and enzymes) influence the metabolic flux or the conversion of metaboliytes through the pathway - Thermodynamics and biochemical reactions - The Gibbs free energy under standard conditions depends on the nature of the reactants and their concentrations at equilibrium - The cellular Gibbs free energy is dependent on the cellular concentrations of substrate and product and standard free energy - An enzyme can couple a favourable and unfavourable reaction to produce an overall favourable reaction Lecture 16: carbohydrate structure and transport - The simplest monosaccharides - Trioses (3 carbons) - Aldehyde -> aldose - Ketone -> ketose - Carbohydrate numbering - Triose, tetrose, pentose, hexose - C double bonded to O is always C1 - Carbohydrate configuration - L or D assigned to chiral carbon furthest away to the carbonyl - 2^n , n=# of chiral centers = total isomers - Sugar structures are very diverse - Sugars all have the same chemical formula (CH2O)n - Ring structure = most stable, low E form - Carbohydrate cyclization - Aldehydes, ketones, and hydroxyl groups are very reactive functional groups (fold in and interact) - The aldehyde/ketone carbonyl undergo nucleophilic attack by hydroxyl groups - Intramolecular reactions for a 5 member furan ring or a 5 member pyran ring - The new bond may be a - Hemiacetal: aldehyde derivative - Hemiketal: ketone derivative - Alpha: on top of OH - Beta: under OH - Anomeric carbons are chiral - Intramolecular cyclization is reversible in solution through the linear chain - The chirality of cyclic structures can be detected by mutarotation; a change in the optical rotation of polarized light, following the interconversion between the alpha and beta anomer - The reactivity of aldehydes (and ketones) also means sugars can react with other molecules (intermolecular reaction) but only in the linear form - Carbohydrate modification - Sugars can be phosphorylated, methylated, or N-containing functional groups may be added - Hydroxyls (or even the carbonyl) may be removed - This increases the complexity of carbohydrate structure - Carbohydrate terminology - Isomers: same molecular formula, different structure - Constitutional isomers: different order of functional group bonding, same formula - Stereoisomers: same formula and order but - Enantiomers: non-superimposable mirror images - Diastereoisomers: not mirror images (different at 1 or more chiral centers) - Epimers: differ at 1 chiral center - Anomers: differ at a newly formed chiral center in the ring structure - Summary of simple monosaccharides - Carbohydrates are aldoses (C1-aldehyde) or ketoses (C2-ketone) - Chiral carbons (x) lead to structural diversity where 2^x determines the number of linear stereoisomers - D or L is decided by orientation around chiral carbon furthest from aldehyde/ketone - Cyclization results in the formation of 2 additional structures - Reducing sugars - Cyclic sugars need a free OH to convert back to a Cdouble bonded O - Glycosidic bonds - Condensation reaction where water is lost - Cleaving glycosodic bonds requires water (hydrolysis) - Monosaccharides are joined by glycosidic linkages to form disaccharides and more comlpex structures - Other types of glycosidic bonds - Intermolecular glycosidic bonds are formed between a hydroxyl or amine and a reactive anomeric carbon on another molecule - Glycoproteins may be O-linked (ser/thr) or N-linked (asn) - Complex carbohydrates - Amylose: unbranched glucose, alpha(1-4) - Amylopectin: built of amylose, linear glucose chains joined by an alpha (1-4) - Alpha 91-6) at branch points once every 30 glucose units - Alpha-amylase is secreted by the salivary glands and pancrea and cleaves at random locations along the chains to give maltose and maltotriose (breaks down amylose) - Cellulose - Unbranched chains of glucose joined by beta(1-4) with many hydrogen bonds - Cellobiose is a disaccharide of glucose linked by beta (1-4) - Negative iodone test due to a different macromolecular structure - Humans dont produce cellulase - Glycogen - Contains branch points every 8-12 glucose units - Contains a dimer of glycogenin at the center - Glucose units are added and removed from the non-reducing ends (releases glucose for energy) - Found in the liver and muscle - Cleavage to monosaccharides - Cells can only transport and use monosaccharides for fuel - disaccharides/polysaccharides must be broken down to monosaccharides for enzymes to use - Lactase, maltase, and sucrase help cleave associated disaccharides - These enzymes are found in the microvilli to help with monosaccharide absorption - Glucose transport - Glucose is a very polar, hydrophilic molecules and cannot diffuse through the lipid bilayer - Transporters help move glucose from one area to another based on the concentration gradient - Sodium-glucose Cotransporters (secondary active transport) - Glucose transporters (faciltated diffusion) - Fructose transporters (facilitated diffusion) Lecture 17: Carbohydrate Metabolism - In the anabolic state insulin signaling increases glucose utilization - The glycolytic pathways is essential for ATP production from simple monosacharides in all cells - Excess glucose is stores as glycogen in muscle or liover by glycogen synthase when ATP levels rise - In the catabolic state epinephrine and glucagon signal for glycogen breakdown leading to either ATP production or the release of glucose from the liver - Glycolysis summary - 10 step catabolic pathway found in the cytoplasm that uses glucose and other simple monosaccharides - Starts with glucose - Stage 1: preparation (steps 1-5) - 2 ATP are used to prepare this glucose molecule for breakdown generation 2 GAPs - Stage 2: (steps 6-10) - Produces 2 pyruvates, 2NADHs and 4ATPs, resulting in a net 2ATP - ATP is produced during glycolysis by substrate level phosphorylation - Pyruvate can be oxidied in the mitochondria generatiing even more NADH (if oxygen available) - Overall the glycolytic pathway is exergonnic under standard and cellular conditions - Regulation of glycolysis - Step 1: hexokinase can be inhibited by the build up of its product (glucose 6 phosphate) - Step 3: phosphofructokinase 1 (PFK-1) is inhibited by the amount of ATP or citrate present, and activated by the amount of AMP, ADP, or fructose 2, 6 biphosphate - Step 10: pyruvate kinase is inhibited by phosphorylation and other allosteric regulators - Step 1: hexokinase - Glucose is phosphorylated using ATP - Hexokinase - hexoses; glucokinase - glucose - Traps glucose in the cell - GLUTs cant bind to glucose 6 p - Only liver cells have an enzyme that can reverse this step - Glucose 6 p will be used by the cells to - Generate energy (glycolysis) - Stored for later (as glycogen in liver or muscle cells) - Used to make new molecules - Hexokinase binding - ATP and Mg2+ bind to large lobe away from glucose binding in the active sites - Substrates bind in the active site via charged and polar residues - Results in a conformational change where the enzyme closes around the substrates - induced fit model of binding - C6 hydroxyl attack the gamma phospahte producing glucose 6-phosphate - Step 3: (PFK-1) - 2nd ATP requiring step pf glycolysis - PFK-1 transfers a phosphate group from ATP to the C1 hydroxyl of F-6P - PFK-1 is inhibited allostericaly by ATP and citrate and activated by AMP or ADP and fructose 2,6 biphosphate - Allosteric regulation of PFK-1 - PFK-1 has 2 ATP binding sites, the active site and an allosteric site - ATP binding to the allosteric site inhibits PFK-1 - AMP or ADP binding to the allosteric site stimulates PFK-1 - Step 10: pyruvate kinase - Inhibited by phosphorylation and other allosteric regulators - Regulation of glycolysis - Glycolysis - Convert monosacchardies to energy - Favourable, catabolic pathway - What if the cell has enough energy - Glycolysis will slow down - What if the cell doesnt have enough energy - Glycolysis is stimulated - Feedback inhibition, allosteric regulation and phosphorylation can regulate the spontaneous reactions in glycolysis - When glycolysis is inhibited, exces glucose can be stores as glycogen in liver and muscle cells - Glycogen synthesis - Excess glucose can be stores as glycogen by glycogen synthase (anabolic) - Glycolysis slows down when ATP levels rise - Glucose is still phosphorylated by hexokinase but isomerized by phosphoglucomutase to form glucose 1 phosphate - UDP-glucose pyrophosphorylase uses UTP to activate glucose 1 phosphate to make UDP-glucose - Glycogen synthase requires UDP-glucose, the activated form o glucose to create new alpha(1-4) glycosidic bonds on the non-reducing ends of a glycogen - Insulin signaling activates glycogen synthase via dephosphorylation - Phosphoglucomutase reaction - Phosphate from 6 to 1 - Insulin signaling -> glucose uptake and phosphorylation - Delta G=0, reversible - Activation step Anabolism vs catabolism - Regulation between the two - Insulin signaling drives anabolic processes - Epinephrine and glucagon signaling (counterregulatory hormones) drive catabolic processes - Glycogenolysis - In response to catabolic state - Driven by blood-glucose levels - Epinephrine and glucagon signaling leads to the phosphorylation and activation of glycogen phosphorylase and inactivation of glycogen synthase via phosphorylation - Break down glycogen - Phosphorolysis reaction, using Pi to release glucose 1-phosphate from non reducing ends - Glucose-1 phosphate cannot be used directly in glycolysis and must be first converted to glucose 6-phosphate by phosphoglucomutase - Glycogen phosphorylase is also allosterically inhibited by ATP and glucose 6-phosphate and stimulated by AMP - Muscle vs liber glycogen - Muscle: - Glycogen - Glucose 1-phosphate - Glucose 6 phosphate - Glycolysis: ATP, lactate, CO2 - Liver: - Glycogen - Glucose 1-phosphate - Glucose 6 phosphate *glucose 6 phosphatase* - Glucose - Blood glucose Lecture 18: Mitochondrial Bioenergetics - Look at this one cause theres a lot of pics - Pyruvate dehydrogenase complex (PDC): connecting glycolysis and the TCA cycle - Links the production in cytoplasm with the TCA cycle in the mitochondrial matrix - It is a large complex of multiple copies of 3 different subunits - The complex requires 5 cofactors for catalysis - Prosthetic group (covalently bound to enzyme): - Thiamine pyrophosphate (TPP) derived from vitamin B1 - Lipoamide - FAD derived from vitamin B2 - Coenzyme (donate functional groups): - Coenzyme A derived from vitamin B5 - NAD+ derived from vitamins B3 - PDC reaction - Products (CO2) more stable that substrates - Pyruvate is decarboxylated into CO2 - Ends with acetyl CoA - 5 step reaction PDC cpmplex - A souluble, complex of 3 enzymatic subunits and 5 different cofactors that can be found in the mitochondrial matrix - Pyruvate (from breakdown of carbs) is oxidized and activated forming acetyl CoA - This reaction is a redox decarboxylation reaction producing CO2, and NADH - The large negative standard free energy change indicates that it is favourable as written and essentially irreversible - Therefore regulation will be important to control this step Pathway integration and regulation - Control by feedback inhibition - Acetyl CoA increase inhibits E2 of PDC - NADH increase inhibits E3 of PDC - PDC PTMs (regulation) - PD kinase inactivates PDC (E1) by phosphorylation - Hydrolysis reaction by PD phosphatase to activate PDC (E1) Regulation of PDC 1. Product inhibition a. Feedback inhibition by Acetyl CoA and NADH on E2 and E3 2. Covalent modification (PTM) is mediated by PD kinase and PD phosphatases: a. The phosphorylation of E1 by PD kinase leads to complex activation b. Dephosphorylation by PD phosphatase restores activity 3. Allosteric control: a. Allosteric control of PD kinase by acetyl CoA, NADH, pyruvate and ADP b. Ca2+ stimulates PD phosphatase (and PDC activity) Acetyl-CoA: at the center of metabolism - All different metabolic fuels can generate acetyl-CoA - This places it at the center of energy metabolism The TCA cycle: oxidizing fuel - The TCA cycle links the breakdown of fuel molecules to ATP production in oxidative phosphorylation Overview of the TCA cycle - Stage 1: oxidative decarboxylation of citrate - acetyl-CoA (2C) combines with oxaloacetate (4C) to produce citrate (6C) (this starts at the TCA cycle) - During the cycle, 2Cs are oxidized to CO2 regenerating oxaloacetate in the process - **all dehydrogenases produce oxidizing agents - Stage 2: regenerate oxaloacetate - The GTP is made by substrate level phosphorylation - 2H2O molecules are needed by reactions - 4 pairs of electrons are used to reduce and form 3 NADH and 1 FADH2 for oxidative phosphorylation - Succinate dehydrogenase is a membrane-bound protein linking the TCA cycle to oxidative phosphorylation Succinate Dehydrogenase (SDH; Complex II) Citric acid cycle thermodynamics - Lower delta Gs close to 0 means reversible - Higher negative ones need to be regulated - Pathways may couple unfavourable and favourable reactions to help drive product formation - Favourable reactions are the steps that are regulated Regulating the TCA cycle - Low energy and calcium stimulate the cycle - High energy, reduced coenzymes or products inhibits the cycle - Ratio of NAD:NAD+ determines if TCA is turned on or off Summary of the TCA cycle - Hub of mitochondrial oxidation and uses acetyl CoA supplied by breakdown of glucose, fatty acids and amino acids - The overall pathways is favourable despite the positive standard free energy of malate dehydrogenase (MDH) - Regulation occurs at favourable directions (negative delta G) to control the cycle - The TCA is considered an amphibolic pathways linking anabolic and catabolic pathways via Acetyl CoA and TCA intermediates Lecture 19: oxidative phosphorylation Mitochondrial oxidative phosphorylation - NADH and FADH2 carry electrons to the ECT - As electrons are passed from carrier to carrier, a change in redox potential generates free energy - The energy is used to power a conformational change in the protein complexes, pumping protons from the matrix into the innermembrane space - Some energy is lost as heat -> maintains body temp - This proton gradient is used by ATP synthase to make ATP in the matrix Electron transfer potential - How much energy is captured - Standard reduction potential (E’o): a molecules tendency to bve oxidized or reduced - Delta G^o’ = -n F deltaE’o - F = 96,485 J/Vmol - N = number of electrons Electron transfer chain - Good reducing agents give up electrons easily and have negative E’o values - Strong oxidizing agents have a greater affinity for electrons and have positive E’o values - Passage of electrons throug the chain (-ve to +ve) results in free energy change that drives conformational changes in the complexes, setting up a proton gradient for ATP synthase Oxidative phosphorylation inhibitors - Rotenone and amytal inhibit electron flow from complex I to CoQ - Antimycin A blocks complex III (cytochome Q builds up) - Cyanide, azide, and CO inhibit complex IV - Oligomycin inhibits ATP synthase (complex V) - Uncouplers can also disrupt the H+ gradient, affecting ATP synthase (uncouple ECT from ATP synthase) Uncouplers - Move electrons back into the matrix - Molecules that have hydrophobic groups that allow to them to cross the membrane - Acidic groups can bind protons and move them from high to low concentrstions ATP synthase - F1 carries out the catalytic synthesis of ATP in the matrix - Fo is the integral membrane protein unit and anchors the complex to the membrane (path for protons to enter matrix) 1. Binding of H+ in the rotor causing a rotation in the ring of c subunits of Fo 2. The rotation of the ring, rotates the gamma subunit, inducing a conformational change in the beta subunits. Protons are released into the matrix 3. Conformational changes in the F1 beta subunits are responsible for ATP synthesis - Each beta subunit functions independently and alternate between 3 states: 1. Open or empty/exit (ATP leaves) 2. Loose - ADP and Pi bound 3. Tight - ATP bound # of H+ needed for ATP synthesis - 3H+ are transported for the production of 1ATP (polar) - 1 extra H+ is needed for ATP export and ADP and Pi import - ATP-ADP translocase and Pi carrier protein - Maintains the charge across the inner mitochondrial membrane - 4H+ required/ATP made in the matrix How many ATPs are made - NADH and FADH2 each donate a pair of electrons to the ETC, resulting H+ being pumped into a pool of protons used by ATP synthase - The P/O ratio tells you how many ATPs (P) are made per oxygen reduced to water (2 electrons from donor) - NADH = 10H+/(4H+/ATP)=2.5 - FADH2 = 6H+/4H+/ATP)=1.5 P/O ratio exceptions - NADH2 from cytosol from glycolysis can not be imported across the inner mitochondrial membrane for use in the ETC - The glycerophosphate shuttle passes electrons from NADH (cyt) to FADH2 in the mitochondria - P/O for NADH(cyt)=1.5(=FADH2) - The P/O ratio also differs depending on the ATP synthase present (different isoforms of ATPase) Water formation in oxidative phosphorylation - In complex IV - NADH + G+ + ½ O2 -> NAD+ + H2O - FADH2 + ½ O2 -> FAD + H2O - By ATPase - ADP+Pi-> ATP+H2O (condensation rxn) - 2.5 H2O made when 2.5ATP are made from NADH 1.5 H2O made when 1.5ATP are made from FADH2 - Water ratio: 3.5 for NADH, 2.5 for FADH2 (or NADHcyt) Lecture 20: Fat metabolism TAGs - Extra carbons are stored in triacylglyercide molecules, primarily in fat cells but also in muscle and other tissues - Acyl chains are connected to a glycerol backbone via ester bonds - Fatty acyl chains are saturated to allow for close packing - Chains must be hydrolyzed off for use - Epinephrine and glucagon signal via GPCRs to activate lipases - Lipases hydrolyze ester bonds to produce free fatty acids and glyercol - Free fatty acids are transported in the blood via albumin for cellular use Fat mobilization via GPCR signaling - Epinephrine and gluagon bind to GPCRs on adipose cell - The signalling response involves the release of Galpha-GTP, activation of adenyl cyclase and cAMP production, leading to PKA activation - PKA phosphorylates and activates TAG lipase, also known as hormone sensitive lipase (HSL) - TAGs are hydrolyzed tp 3 fatty acid (+glyercol) that are released in the blood and transported via albumin Fatty acid activation in the cytoplasm - Acyl chains are trapped in the cell by the covalent addition of CoA - Acyl CoA synthetase catalyzes a reversible reaction uses ATP->AMP to form the fatty acyl CoA molecule - PPi hydrolysis to 2Pi is favourable and drives the reaction in the forward direction ATP->AMO us energetically inefficient - In order for ATP to be made from AMP, AMP must be converted to ADP by sacrificing a 2nd ATP - ATP synthase only binds to ADP - Fatty acid activation by CoA requires 2 ATP (1 for activation and 1 for the production of ADP) and 1 H2O to hydroylze PPi Acyl CoA and beta-oxidation - Acyl CoA is made in the cytoplasm, but b-oxidation occurs inside the matrix - Acyl CoA can pass through outer membrane into the intermembrane space via porins, but it cannot cross the inner membrane since there is no transport mechanism - CoA is too large and water soluble making it unable to cross membrane - What the point of making fatty acyl CoA outside the matrix if its only used in the matrix? -> compartmelinization (regulation) Carnitine shuttle - The carnitine shuttle regulates what comes into the mitochondria - Carnitine acyltransferase I (CAT I) moves te acyl chain onto carnitine from CoA - Acyl carnitine is translocated across the inner membrane in exchange for carnitine - Carnitine acyltransferase II (CAT II) moves the acyl chain back onto CoA - Acyl CoA can then be catabolized in b-oxidation Overall b-oxidation of 16:0 CoA in the mitochondria - 16:0 CoA + 7NAD+ + 7FAD + 7H2O + 7CoA -> - 8 acetyl CoA + NAD + 7FADH2 + 7H+ Grand total for the complete oxidation of 16:0 CoA in the mitochondrial matrix - 16:0 CoA + 108ADP + 108PPi + 23O2 -> - 16CO2 + 108ATP +123H2O +CoA Summary of fatty acid breakdown - Epinephrine and/or glucagon are required for TAG breakdown - Fatty acids travel in blood stream bound to serum albumin and enter the tissues - Fatty acids are activated in the cytoplasm by acyl CoA synthetase, requiring 2 ATP and H2O - The carnitine shuttle controls fatty acid entry into the matrix (CAT I, translocase, CAT II) in exchange for free carnitine - Acyl chains are borken down in b-oxidation oroducing acetyl CoA, NADH, and FADH2 - B-oxidiation can produce a huge amount of ATP using the citric acid cycle and oxidative phosphorylation Using fats as fuel - In order to generate ATP in the mitochondria, acetyl CoA from fat degradation needs to be processed via the citric acid cycle - The CAC is dependent on the amount of OAA present - In order to efficiently make ATP from fat, cells need OAA - OAA can be made from amino acids or pyruvate by an enzyme called pyruvate carboxylase - You need a blend of fuels, both glucose, amino acids, and fats for efficient energy production Hormonal response and fat synthesis - As glucose levels rise, insulin signals for glucose uptake and catabolism in glycolysis. Fats and amio acids will also be imported for use in the cell - As ATP levels rise, glycolysis slows down and excess glucose is stored as glycogen in muscle and liver cells - As ATP and NADH levels rise in the mitochondria, the CAC slows down, allowing for the synthesis of fat from acetyl CoA - Excess macromolecules will first be broken down to smaller precursors and energy that can be used to make fats, but acetyl CoA is made in the mitochondria Steps in fatty acid synthesis 1. Export of mitochondrial acetyl CoA to the cytoplasm for fat synthesis 2. Carboxylation of acetyl CoA to malonyl CoA by acetyl CoA carboxylase (ACC) 3. Use of malonyl CoA to form 16:) fatty acid chains by fatty acid synthase (FAS) Step 1. Acetyl CoA is exported as citrate - Recall coA cannot cross the inner mitochondrial membrane - Citrate synthase makes citrate in the CAC, which can cross the inner membrane - Citrate is broken down by different enzymes to recreate acetyl CoA, regenerating a pyruvate molecule - The pyruvate can then return to the mitochondria while acetyl CoA is used for the synthesis of fatty acyl chains in the cytoplasm Fatty acid synthesis is an anabolic process - Acyl chain synthesis occurs in the cytoplasm - 2 enzymes are needed - acetyl CoA carboxylase and fatty acid synthase - ACC converts acetyl CoA to malonyl CoA, an activated 2-carbon carrying precursor for fatty acid synthesis - Acetyl CoA carboxylase is the committed and regulated step for fatty acid syntheis - Acetyl CoA carboxylase uses ATP, while FAS uses NADPH as reducing power Step 2. Acetyl CoA carboxylase (ACC) - Acetyl CoA is carboxylated using ATP to produce malonyl CoA - The presence of the CO2 group on malonyl CoA will be used to drive fatty acid synthesus by fatty acid synthase Reciprocal regulation for fats - When fat synthesis is on, fat degradation is off - This prevenrs the futile cycle of synthesizin chains, then breaking them down - The product of acetyl CoA carboxylase (ACC) - malonyl CoA, inhibits CATI and shuts down the carnitine shuttle for the import of chains - ACC is also regulated for fat metabolism ACC regulation via phosphorylation Regulation of fat metabolism - Acteyl CoA carboxylase is the committed step for FA synthesis - ACC is inhibited by phosphorylation with rising glucagon/epinephrine and AMP - Insulin and protein phosphatase activate ACC via dephosphorylation - Citrate can also stimulate ACC while palmitoyl CoA inhibits active ACC - The product, malonyl CoA shits down fat breakdown by inhibiting carnitine acyltransferase 1 Step 3. Use of malynol CoA to form fatty acid chains by FAS Fatty acid synthase - Step 1: a condensation reaction (KS) occurs with the release of CO2, producing a chain that is 2 carbons longer - Step 2: redox reaction requiring NADPH(KR) - Step 3: dehydration and H2O is released (DH) - Step 4: 2nd redox reaction usin NADPH producing a saturated chain (ER) - Repeat : 2 carbons from another malonyl CoA can then be used for the next round of reactions Fat synthesis - The synthesis of fatty acids requires the actions of both acetyl CoA carboxylase (ACC) and (FAS) - 7 cycles of FAS are required to generate 16:). This involves 1 acetyl CoA and 1 malonyl CoA in the first cycle, followed by 6 malonyl CoA molecules for the next 6 runds of reactions - Lots of energy is required for this anabolic pathway to proceed (ACC needs 7ATPs to make 7 malonyl CoAs anbd FAS needs 14NADPHs) - Elongation of the 16:0 chain occurs in the ER also using malonyl CoA and NADPH - Desaturases can introduce cis bonds, also requiring NADPH and oxygen Lecture 21: Lipoproteins - TAG formation - Triglycerides are made with phosphatidate (phospholipid) - The phosphate is first removed by a phosphatase producing DAG - DAG acyltransferase adds the 3rd fatty acyl tail to make TAG - Elevated insulin promotes the activation of all the enzymes involved - ACC, FAS, and acyltransferase - TAGs produced in the liver can be released in the blood stream as lipoproteins for storage or use - Lipoproteins - Transport molecules for hydrophobic TAGs and cholesterol esters throughout the body - They have a thin monolayer of phospholipids and cholesterol - The polar portions of the molecules face the aqueous exterior, while the hydrophobic ones face the hydrophobic interior - Apoproteins are on the surface of the lipoproteins and are oriented with their hydrophilic ones facing the hydrophobic interior - Apoproteins are on the surface of the lipoproteimns are are oriented with their hydrophilic side chains outward and hydrophobic side chains facing inwards - Cholesterol metabolism - Cholesterol is crucial for membranes and is metabolized to produce hormones, bile salts and vitamin D3 - Cholesterol is made from acetyl CoA in the cytoplasm of liver cells using carbons from fat/protein/carb catabolism - Cholesterol can be modified to a hydrophobic, acyl-carrying molecule called a cholesterol ester (CE) by acyltransferase - The production of cholesterol requires NADPH and ATP and is regulated by inhibiting an enzyme called HMG-CoA reducatse - Lipid transport via lipoproteins - Lipoproteins are made in the liver or intestine - As they travel through the body, fatty acids and cholesterol are transferred to the tissues to produce energy, build membranes, or to produce other molecules - Whatever doesnt get used, returns to the liver as lipoprotein remnants for recycling. Additional cholesterol and/or TAGs are synthesized for the production of new lipoproteins - Excess synthesis of fats, defects in the receptors that recycle lipoproteins, or problems with the expression and/or activity of HMG-CoA reductase can disrupt the balance of lipid metabolism and lead to high cholesterol and CVD - Lipo-proteins - The different lipoproteins can be differentiated by their individual components (apoproteins %, TAGs%, cholesterol) - Chylomicrons are made in the small intestine from the diet and are the largest and contain and apoprotein known as apoprotein 48 - Very low density lipoprotein (VLDL) is made by the liver and contains apoB100 protein - Lipoprotein lipase attacks VLDL, hydrolyzing TAGs to produce intermediate density lipoprotein (IDL) and then low density lipoprotein (LDL)->bad cholesterol - High density lipoprotein (HDL) is the good cholesterol that picks up cholesterol and CEs and transports them back to the liver for breakdown and recycling - Cholesterol and CVD - Cholesterol is found in the diet but it is also synthesized in the liver (800mg/day) - The liver is responsible for monitoring cholesterol levels and for lipoprotein recycling and the synthesis of new cholesterol - Increased cholesterol intake, synthesis in the liver or decreased lipid turnover and use can lead to CVD - Oxidixed LDL particles have been found to be the principal components in arterial plaques resulting in heart attack and stroke Lecture 22: gluconeogenesis - Anaerobic metabolism - During periods of limited oxygen, the ECT is inhibited, and anaerobic metabolism occurs - All energy production in oxidative phosphorylation is inhibitedm and the PDC and CAC slow down - Glycolysis is the only means of generating ATP - Lactate dehydrogenase converts NADH back to NAD+ so that glycolysis (step 6) can continue - Lactate is a biochemical marker of anaerobic metabolism - Cori cycle - Red blood cells dont have mitochondria, always in anaerobic metabolism - Produce lactate that goes to liver for GNG - GNG - In addition to glycogen breakdown, the liver and kidney can make glucose via GNG - This is important because your brain cant use fats for energy, it needs glucose to generate ATP - Key substrates include lactate, glycerol, and certain amino acids that can be turned into glucose - These carbon containing molecules are readily available in large quantities from the bloodstream - GNG is not simply the reverse of glycolysis - Glycolysis is exergonic and would require lots of energy (standard=84kJ/mol) for the 10 reactions to go in reverse to make glucose - GNG is an anabolic pathway that uses high energy intermediates and 4 different enzymes to bypass the 3 steps in glycolysis that have negative free standard G and are essentially irreversible - Fructose-6-phosphate formation - 2 PEP goes through the reverse sequence of enzymes in glycolysis to form a DHAP and GAP which joins to form fructose1,6-bisphosphate - A phosphatase reverses step 3 of glycolysis (PFK-1) - Fru 6-P is isomerized to Glc 6-P - Glucose formation (only in liver) - Glucose 6-phosphatase hydrolyzes off the phosphate to produce free glucose that can exit the cell - This enzyme acts on Glc 6-P made from glycogen breakdown (glycogen phosphorylase + phosphoglucomutase) - Glucose production from (2) pyruvate - Glycerol to glucose production - Fatty acids cannot be used to generate glucose because the production of pyruvate from acetyl CoA is unfavourable - The glycerol that is released during fat metabolization in fat cells can be used and is released in large amounts into the blood - Glycerol has 3 carbons; 2 glycerol molecules can be used to make a 6 carbon glucose entering the pathways as dihydroxyacetone phosphate (DHAP) - 2 ATPS are needed to drive this anabolic pathway but 2 NADHs are also produced. 2 waters help remove phosphates to make 1 glucose molecule Lecture 23: ketogenesis - Acetyl CoA and ketogenesis - Fat reserves are a huge source of acetyl CoA and generate more ATP via beta oxidation than glucose alone - The catabolsim of amino acids can produce glucose or acetyl CoA in the liver - Fat and amino acid catabolism occurs when epinephrine and glycagon (GPCR signalling) dominate - Acetyl CoA enters the CAC but is limited by the amount of OAA present - OAA may be depleted when glucose levels drop - Ketogenesis occurs when acetyl CoA levels rise so that ketone bodies can be used as an alternate fuel source to glucose - Metabolism of amino acids - In addition to their roles in protein structure, amino acids can also enter in the metabolic pathways at various points - The alpha amino group is first removed and metabolized in the urea cycle for excretion as ammonia - The carbon backbone is used to make ATP, glucose, or ketone bodies - Non essential amino acids can also be made from intermediate metabolites - Amino acid metabolism - Excess amino acids are degraded in the liver - Amino acid carbon skeletons are used to generate energy entering as pyruvate, acetyl CoA or CAC intermediates - Essential amino acids must be obtained from the diet due to a more complex (6-15 steps) biosynthetic pathways - Glucogenic amino acids can be used to make glucose, while ketogenic amino acids produce acetyl CoA and ketone bodies - 3 ketone bodes are made in the liver - Beta-hydroxybutyrate - Acetoacetate - Acetone - Use of ketone bodies as fuel by the brain or other tissues - Ketone bodies leave the liver and enter the bloodstream - Ketone bodies can cross the BBB - Tissues can convert them back to acetyl CoA to generate energy - What molecule is needed to produce energy from acetyl CoA?: OAA - Where does it come from?: GNG->Glc->pyruvate - Ketogenesis - The processing of acetyl CoA in the CAC is limited by the amount of OAA present - When glucose levels drop and acteyl CoA levels rise, ketone bodies are instead made in the liver for energy production - Acetoacetate and beta-hydroxybutyrate can be used as fuel by the brain, heart, muscle, and kidney cells - Acetone is a waste product and gives characteristic fruity breath symptom - The over production of the acidic ketone bodies can, however, contribute to a drop in blood pH and acidosis - keto(acido)sis - Occurs when ketone levels rise in the body due to depleted liver glycogen stores and increased acetyl CoA (starvation, low carb diets, diabetes) - The blood buffering system initially compenesates along with H+ absorption by bone and tissue, as well as renal secretion - Acidosis can occur when bicarbonate is depleted and the blood pH drops below 7.35 - Liver metabolism - The liver is the main supplier of glucose for the rest of the body releasing glucoe from glycogen breakdown and producing it in GNG - Cholesterol and fatty acid synthesis also take place in the liver, releasing them as lipoprotein complexes in the bloodstream - Fatty acids cant be used by the brain for energy, but the liver can provide ketone bodies that get converted back to Acetyl CoA - Acetyl CoA from fat breakdown and ketogenic amino acids can be used to make ketone bodies, while glucogenic amino acids can be used to make glucose - The liver helps supply important fuel molecules for the rest of the body - Ketoacidosis in the absence of insulin - Without insulin, glucose transporters wont be recruited to the cell surface to help with glucose import - Since glucose cant be broken down to make energy, fat catabolism can help - CAC in liver slows because cycle intermediates are not replenished in the absence of pyruvate/OAA, leading to excess acetyl CoA boeing converted to ketone bodies - The production of ketone bodies is associated with a drop in blood pH, leading to ketoacidosis - Deep respiration removes CO2 from blood, which causes a loss of bicarbonate and a lack of buffering capability - Acidosis affects protein structure and/or function, and can lead to unconsciousness, coma or death - Effects of insulin administration - Reduced hyperglycemia and ATP levels rise - Polydipsia and polyuria are eliminated due to a drop in blood glucose and ketone body formation - Blood tonicity normalizes, along with water balance - Glycolysis is stimulated while GNG/glycogenolysis is inhibited - Fat breakdown is also inhibited while fat and glycogen synthesis rises (from excess carbon molecules) - Reciprocal regulation - The goal of reciprocal control in the liver is to allow either glycolysis or GNG to run, not both at the same time - Regulation is achieved by hormone signalling through enzyme (de)phosphorylation and the availability of energy - The tandem PFK-2/FBPase-2 enzyme controls the production and breakdown of fructose 2 6, bisphosphate for allosteric regulation - Energy charge also regulates these pathways: - Lower energy (AMP/ADP) stimulates glycolysis (PFK-1, pyrivate kinase) and slow down GNG (pyruvate carboxylase, PEP carboxykinase, F1, 6-bisPase) - Higher energy (ATP) slows down glycolysis (PFK-1, pyruvate kinase) and stimulates GNG (pyruvate carboxylase, and F1,6bisPase) - Hyperglycemia nad glycation - Glucose is highly reactive and can react with the N-terminal valine residues of the beta chains in hemoglobin - This forms hemoglobin A1c (HbA1c) is a marker for high blood over every 120 days - Other molecules in the body can by glycated (AGEs) resulting in their modification which may affect their function - Hormonal regulation and diabetes - People with type I diabetes need to balance their insulin injections with carbohydrate intake and exercise - Insulin injections are necessary for glucose uptake and can also inhibit glucogon secretion - A lack of insulin or insulin insensitivity (type 2) leads to poor cellular glucose uptake and hyperglychemia. Glucagon signalling can also turn on GNG, worsening hyperglycemia - When blood glucose levels drops in the presence of insulin, glycogenolysis and GNG cannot help maintain blood glucose levels - Low blood glucose = hypoglycemia - Fat breakdown and ketogenesis could assist with ATP production - Summary - Anaerobic conditions limits the amount of ATP produced in a cell and produces the waste product lactate - The Cori cycle allows for lactate to be used in GNG along with other non-carb precursors - GNG occurs in the liver (and kidney) supplying the body with glucose, in addition to glucose from glycogen breakdown - GNG is an anabolic pathway that requires energy and involves 4 different enzymes that bypass the 3 irreversible steps in glycolysis - Glycolysis and GNG are reciprocally regulated via allpsteric regulation and phosphorylation but is also dependent on energy and metabolite availability Learning Goals: Lecture 13: - Review the composition and roles of biological membranes and how the diverse chemical structures of molecules contribute to its formation - Explain how fluorescence microscopy can be used to visualize the movement of molecules in a membrane and why membrane fluidity is important for movement - Define the unnique properties of integral membrane proteins and predict the topology of a membrane protein based on the amino acids present - Describe the role of detergents and salt when purifying a membrane protein Lecture 14: - Explain how the structure of a membrane protein is crucial for its ability to move ions, molecules, or transmit signals across the membrane - Describe the essential features of the mian types of signalling pathways - Identify the role of lipids and enzymes play in transmittinig signals across the membrane and throughout the cell - Distinguish between passive and primary vs. secondary active transport Lecture 15: - Compare the features of anabolic and catabolic pathways - Explain the general organization and regulation of enzymes within pathways - Review the thermodynamics in the context o enzymes and pathways and predict favourable reactions - Describe the importance of phosphoryl transferase potential for high energy molecules Lecture 16: - Explain the importance of carbohydrate structure for biochemical processes - Describe the organizaton of functional groups and how they contribute to carbohydrate comlpexitiy (mono s. polysaccharides) - Differentiate between the enzymatic synthesis and breakdown of complex carbohydrates - Describe how carbohydrates are transported across the plasma membrane and into the cell Lecture 17: - Summarize how glycolysis breaks down glucose for energy production - Examine the role of thermodynamics as a driving force in glycolysis and glycogen synthesis - Describe how glycolysis can be regulated depending on metabolite concentrations and cellular ATP requirements - Examine how hormone signalling can lead to glucose uptake, use, storage, or production and compare the regulation and mechanisms of the enzymes involved Lecture 18: - Same as 17 Lecture 19: - Explain how electrons flow through the electron transport chain to generate a proton gradient - Examine the conformational changes needed for proton transport and ATP synthesis by ATP synthase - Define the P/O ratio for NADH and FADH2 - Calculate the number of ATPs and water molecules produced from glucose (and other molecules) under aerobic conditions Lecture 20: - Describe why, when, and where fats are made and broken down - Review how much ATP is made from the reduced coenzymes produced in beta oxidative phosphorylation - Describe the role of ACC and FAS for fat synthesis - Compare lipid synthesis to lipolysis (beta oxidation) - Contrast the reciprocal regulation of fat metabolism Lecture 21: - Describe the structure and function of lipoproteins - Summarize how lipoproteins are made and how they travel throughout the body - Contrast ‘good’ and ‘bad’ cholesterol and examine the role the lover plays in cholesterol levels - Devise a biochemical strategy to maintain good cardiovascular health Lecture 22: - Outline the role of gluconeogenesis plays in maintaining glucose homeostasis in the body - Summarize how various substrates can be used to generate glucose in the liver, and why glucose is important for the brain - Explain why lactate is considered both a waste product and substrate for gluconeogenesis - Review the role of thermodynamics as a driving force in glycolysis vs. gluconeogenesis - Compare the regulation of gluconeogenesis to glycolysis for determining which pathway is active in the liver Lecture 23: - Summarize the biochemical pathways leading to ketogenesis - Describe where and when ketone bodies are made - Discuss the physiological role of ketone bodies - Explain the impact of ketoacidosis and how it arises in a person with diabetes - Contrast the roles of glucogenic and ketogenic amono acids