AMR Summary PDF
Document Details
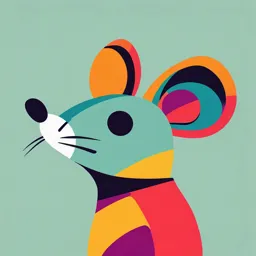
Uploaded by EvocativeHope
Imperial College London
Tags
Summary
This document provides a summary of microbial diversity and bacterial anatomy. It covers various structures, processes, and factors related to bacteria, such as outer and inner structures, growth phases, and quorum sensing. It also touches on bacterial communication and interactions.
Full Transcript
AMR Summary Microbial diversity and bacterial anatomy: - Microbial diversity is vast -- bacteria are most diverse of all organisms - Eukaryotic pathogens a small fraction of microbial diversity but often similar to other eukaryotic organisms -- difficult to target pathogens withou...
AMR Summary Microbial diversity and bacterial anatomy: - Microbial diversity is vast -- bacteria are most diverse of all organisms - Eukaryotic pathogens a small fraction of microbial diversity but often similar to other eukaryotic organisms -- difficult to target pathogens without targeting eukaryotic processes - Bacteria have varied structures, including cocci, bacilli, and other non-classified forms, but all share some common features. - Prokaryotic cells divided into outer and inner structures separated by cytoplasmic membrane - Outer Structures: - Glycocalyx (Capsule): A polysaccharide layer that can be rigid (capsule) or deformable (slime) -- aids in pathogenicity and immune invasion - Flagella: Protein structures enabling motility, attached to the cytoplasmic membrane and capable of complex movement -- distribution varied - Pili/Fimbriae: Shorter appendages used for attachment to surfaces -- involved in DNA transfer between bacteria - Inner Structures: - Endospores: Resistant cells formed under environmental stress -- can survive extreme conditions and revert to vegetative cells under favourable conditions (mostly gram pos) - Ribosomes: Used for protein synthesis, with a 70S structure made of 30S and 50S subunits, each associated w RNA molecules - Nucleoid: contains bacterial DNA, which is supercoiled for efficient packing since no nuclear membrane -- coiled via topoisomerase and gyrase enzymes - Plasmids: confer resistance mechanisms, separate from chromosomal DNA + autonomously replicate -- transfer usually requires cell-cell contact - Cell Wall: - Gram-Positive Bacteria: thick peptidoglycan layer, teichoic acid, and anchor proteins - Gram-Negative Bacteria: more complex, multi-layered cell wall with -- outer membrane contains lipopolysaccharide (LPS). - Gram Staining: crystal violet bonds to peptidoglycan -- gram pos purple, gram neg red - Cytoplasmic Membrane: - A selective permeability barrier (phospholipid bilayer) -- nutrient transport and energy conservation - Population Growth: - Growth: an increase in cell number or mass over time - Exponential Growth: Bacteria double in number every generation -- tracked via a growth curve - Bacterial Growth Phases: - Lag Phase: Cells adapt to the new environment without immediate growth (gene expression, molecule synthesis) - Exponential (Log) Phase: optimal growth -- mass and number increase exponentially - Stationary Phase: growth + death rate at equilibrium because less resources, more waste - Death Phase: death due to no resources + toxic waste build up - Growth phases often reflect stages of infection -- diff toxins/enzymes/adhesins produced can indicate stage - Factors Affecting Growth: - Influenced by temperature, medium composition, and other environmental conditions - Bacteria may exhibit different metabolic behaviours based on nutrient availability (e.g., diauxic growth -- preferentially consume one nutrient before switching to another) Quorum sensing (bacterial communication): - Bacteria use autoinducers to detect local population density - Gram pos produce small peptides (AIPs); gram neg produce N-acyl homoserine lactones - Quorum sensing regulates bacterial biology, including virulence factors -- optimise population growth Biofilms: - Bacteria can grow in 3D biofilms, often on surfaces - Biofilms can be single-species (lab) or multispecies (natural environments) - Biofilms start with colonizer bacteria that associate on a surface, then proliferate, form external matrix, and develop channels for nutrient flow Prokaryotic Nutrition: - Prokaryotes need chemical tools to produce building blocks (macromolecules). - Macronutrients (carbon, nitrogen, phosphorous, sulphur) are required in large amounts. - Micronutrients (metal ions) are needed in small amounts for enzyme function. Macronutrients - Carbon: Needed for new cell material, obtained from CO2 (autotrophs) or organic compounds (chemotrophs). - Nitrogen: Needed for DNA and proteins, typically from NH3 or nitrogen-fixing bacteria. - Other Macronutrients: Phosphorous, sulphur, magnesium, and iron play roles in DNA, amino acid, cell wall, and respiration processes Micronutrients - Required in trace amounts, metal ions, for enzymes. - Important for cell metabolism and AMR targeting Catabolism & Anabolism - Catabolism: Breakdown of molecules to produce ATP, often via redox reactions. - Anabolism: Biosynthesis of complex molecules (proteins, DNA) from precursors, powered by ATP or proton motive force. - Link: Anabolism and catabolism are tightly linked in metabolism. Bactericidal vs Bacteriostatic Antibiotics - Bactericidal: Kill bacteria; reduce bacterial population. - Bacteriostatic: Prevent bacteria from proliferating; population remains steady. - In vitro vs In vivo: The effect can vary depending on dosage. Antibiotic Targets in Bacteria - Cell Wall Synthesis Inhibition: Penicillins, cephalosporins, vancomycin. - Protein Synthesis Inhibition: Chloramphenicol, tetracyclines, macrolides. - Nucleic Acid Synthesis: Quinolones, rifampicin. - Plasma Membrane Injury: Polymyxin B. - Metabolite Synthesis Inhibition: Sulphonamides, trimethoprim. Specific Antibiotics - Sulphonamides & Trimethoprim: Bacteriostatic, target folate synthesis, used in treating infections like UTIs and RTIs. - Quinolones: Bactericidal, target DNA gyrase/topoisomerase. - Tetracyclines: Bacteriostatic, prevent protein synthesis by binding to the 30S ribosomal subunit. - Chloramphenicol: Bacteriostatic, inhibits protein elongation by binding to the 50S ribosomal subunit. - Macrolides: Bacteriostatic, used in Gram-positive infections, targets the 50S ribosomal subunit. - Aminoglycosides: Bactericidal, target protein synthesis and cause membrane damage (e.g., gentamicin, streptomycin). - Vancomycin: Bactericidal, inhibits peptidoglycan synthesis in cell walls. - Beta Lactams: Bactericidal, inhibit peptidoglycan synthesis (e.g., penicillin, cephalosporins). - Carbapenems & Colistin: Last-resort antibiotics, used for resistant bacteria, carbapenems are beta-lactams, and colistin targets Gram-negative outer membranes. Why So Few Targets? - There are fewer targets for antibiotics because of the significant differences between mammalian and bacterial biology, with fewer distinct bacterial structures or pathways to target effectively. Fundamentals in antibiotic resistance: Antibiotics and Antimicrobials - Antibiotics: Substances derived from microorganisms that kill/inhibit other microbes. Categories: Natural: Directly sourced from microbes (e.g., Penicillium) Semi-synthetic: Modified versions of natural antibiotics Synthetic: Entirely lab-produced - Antimicrobials: Broader category targeting bacteria, fungi, or viruses (not always biologically derived) Bactericidal: Kill bacteria Bacteriostatic: Inhibit bacterial growth Antiseptics: Applied topically for microbial control Resistance basics: - Resistance occurs when the required antibiotic concentration to inhibit bacterial growth exceeds the effectiveness threshold (breakpoint) - Minimal Inhibitory Concentration (MIC): Lowest concentration needed to stop bacterial growth Applications of antibiotics: - Prophylaxis in surgeries and organ transplants - Cancer chemotherapy and trauma care - Veterinary medicine and agricultural use History and emergence of resistance: - Salvarsan: First targeted antimicrobial treatment for syphilis - Prontosil: Early sulphonamide antibiotic - Penicillin: Revolutionized treatment but foresaw resistance (Fleming, 1945) - Misuse (under-dosage or inappropriate application) predicted to fuel resistance Antibiotic Resistance: The Growing Threat - Global Problem Increasing resistance in pathogens like Staphylococcus aureus, E. coli, and Acinetobacter Example: Norway's public health measures successfully reduced resistance - Selective Pressure Antibiotic use selects for resistant bacteria Resistant bacteria can spread even among untreated individuals Mechanisms of antibiotic resistance: - Altered Target Site Bacteria acquire genes or enzymes that modify antibiotic targets (e.g., MRSA) Example: Streptococcus pneumoniae modifies ribosomal targets via the erm gene - Antibiotic Inactivation Enzymes degrade antibiotics (e.g., beta-lactamases, NDM-1) - Altered Metabolism Overproduction of substrates to outcompete antibiotics (e.g., sulphonamide resistance) Switching metabolic pathways to avoid targeted processes - Decreased Drug Accumulation Reduced membrane permeability (e.g., Gram-negative bacteria) Increased efflux pumps expel antibiotics Sources of resistance genes: - Plasmids: Extra-chromosomal DNA with multiple resistance genes - Transposons: Mobile genetic elements transferring genes between chromosomes and plasmids - Naked DNA: Free DNA from lysed bacteria, available for uptake Spread of resistance: - Horizontal gene transfer: Conjugation: DNA transfer through direct bacterial contact Transformation: Uptake of environmental DNA Transduction: Gene transfer via bacteriophages Key pathogens of concern: - ESKAPE Pathogens: Enterococcus faecium, Staphylococcus aureus, Klebsiella pneumoniae, Acinetobacter baumannii, Pseudomonas aeruginosa, Enterobacter spp Cause hospital-acquired infections and exhibit high multidrug resistance - Neisseria gonorrhoeae: Sexually transmitted pathogen with increasing untreatable cases - Mycobacterium tuberculosis: Infects 25% of the global population; dormant infections can reactivate Extensively drug-resistant strains pose a critical threat - Plasmodium falciparum: Malaria parasite resistant to all current antimalarial drugs - Fungi: Growing resistance with limited and often toxic treatment options Ecological complexity: - Efficient Gene Transfer: Particularly prevalent in pathogens like ESKAPE and N. gonorrhoeae - High Global Prevalence: Diseases like tuberculosis and malaria impact billions - Fungal Challenges: Limited drug availability and inherent resistance mechanisms Clinical and societal implications: - Patient Outcomes: Increased mortality, longer hospital stays, and higher treatment costs Reliance on toxic or less effective drugs (e.g., outbreak in Europe costing €11M in 10 months) - Microbiome Disruption: Antibiotics disrupt commensal bacteria, reducing competition and enabling resistant strains to thrive Biofilms and phenotypic resistance: - Growth arrest in deeper layers - Cooperative antibiotic degradation - Physical barriers slowing drug penetration Evolution of resistance: - Resistance determinants predate human antibiotic use (e.g., ancient tetracycline resistance genes) - Natural selection within microbial ecosystems promotes resistance Potential solutions: - New Antibiotics Develop drugs targeting novel mechanisms - Policy Measures: Reduce misuse and overprescription - Chemical Inhibitors: Block mechanisms like horizontal gene transfer - Diagnostics: Rapid and accurate diagnostics for targeted treatments - Alternative Therapies: Explore non-antibiotic treatment options Persister cells: - Some bacteria form "persisters" to evade antibiotics (e.g., Mycobacterium tuberculosis, Salmonella typhi) - Growth arrest often due to microbial signalling, including toxin-antitoxin systems - Persisters make bactericidal antibiotics bacteriostatic Toxin-antitoxin systems: - Involves toxins (cause growth arrest) and antitoxins (inhibit toxins) - Stress causes degradation of antitoxins, allowing toxins to act Reversible intoxication and persister formation: - Toxins like TacT from Salmonella arrest growth, promoting persister formation MRSA and antibiotic resistance: - S. aureus can acquire resistance via horizontal gene transfer (HGT) or mutation - Methicillin: a penicillin derivative resistant to some beta-lactamases - Beta-lactam resistance routes: Beta-lactamases degrade beta-lactams Pbp2a (penicillin-binding protein) resists beta-lactam inhibition Methicillin-Resistant S. aureus (MRSA): - Acquires alternative Pbp2a through SCCmec element (transposase) - Vancomycin-resistant S. aureus (VRSA) can acquire vancomycin resistance from Enterococcus faecalis - Emerged in hospitals due to high antibiotic use, large immunocompromised population, and indwelling devices - Community-acquired MRSA (CA-MRSA) is more virulent and affects healthy individuals Differences Between HA-MRSA & CA-MRSA: - HA-MRSA: More resistant to antibiotics, typically infects immunocompromised individuals - CA-MRSA: More virulent, infects healthy individuals, smaller SCCmec elements Livestock-Associated MRSA (LA-MRSA): - Resistant due to beta-lactam use in cattle, variant mecA gene - Found in pets and human patients Spontaneous Resistance to Other Antibiotics: - Resistance to daptomycin, ciprofloxacin, rifampicin via mutation - Vancomycin resistance in S. aureus via point mutations (VISA, VRSA) Vancomycin resistance: - Progressive resistance involves cell wall modifications (thickening, reduced crosslinking, and charge changes) Antibiotic Resistance and Spread: - Resistance to new antibiotics emerges quickly and spreads - Vancomycin introduced in 1954, widespread use in 1990s New antibiotic discovery: - Phenotypic Screens: Test compound libraries for biological activity - Targeted Screens: Test compounds for specific chemical activities - Rational Design: Identify compounds that bind to specific targets Challenges in rational drug design: - Chemistry, off-target effects, on-target toxicity - Antibodies and large proteins are promising but expensive for antimicrobials Sources of antimicrobial compounds: - Natural: From soil bacteria (Streptomyces) and fungi - Synthetic: Created via small-molecule screening libraries - Semisynthetic: Modified natural products for efficiency or spectrum Antimicrobial compound development: - Cephalosporins developed from fungus-derived beta-lactams: 1st gen: Active against Gram-positives, limited Gram-negative coverage 2nd gen: Broader Gram-negative coverage, less beta-lactamase sensitive 3rd gen: Improved against Gram-negatives, CNS penetration 4th gen: Broader spectrum, better resistance to beta-lactamase Progression in antimicrobial synthesis: - Modifying natural products through successive generations to overcome resistance AMR strategies: - Broad vs. narrow-spectrum antibiotics - Resistance inhibition, disease-specific treatments, host-directed therapies, biological control (e.g., phage therapy) Antimicrobial Drug Discovery: An Overview Importance of antimicrobials: - Historical significance: Discovery of antibiotics in the 1930s led to the \"Golden Age of Antibiotics\" Increased life expectancy by 8 years (1944--1972) Overconfidence in their impact led to claims like \"close the books on infectious diseases\" (US Surgeon General, 1969) - Antimicrobial resistance (AMR): Resistance emerged as early as four years after penicillin\'s introduction 1950s--1960s: Resistance was minor due to continuous discovery of new antimicrobials By the 1970s, widespread resistance in common pathogens emerged Today, resistance has been reported for all antimicrobials on the market Drug discovery process: - Traditional drug discovery cycle: Primary screen: High-throughput and in vitro assays to test for basic properties like safety Secondary screen: Assesses bioavailability, toxicity, metabolism, and more DBT cycles: Iterative design-build-test cycles to optimize lead compounds Outcome: A clinical candidate may result after several cycles and screens Screening methods: target-based screens - Features: Focus on a specific target with known disease association Clear, fast, and scalable with simple, interpretable data Requires purified targets and assumes prior knowledge of target-disease links - Readouts: Colorimetric, fluorescence, radioactivity, microscopy-based methods High-performance computing may be needed for data analysis Phenotypic screens: - Features: Focus on biological outcomes rather than specific targets Strengths: Good in vivo translation, potential to discover novel biology Weaknesses: Slow, unclear mode of action, requires phenotype-disease association Screen quality assessment: - Z factor: Statistical measure of assay quality (ideal Z′ = 1) Used to ensure controls can reliably identify hits Desirable features of screen hits: - Effect matches drug concentration (dose-dependent) - Low EC50 for effective ADME properties - Non-toxicity demonstrated in secondary screens - Analogues available for structure-activity relationship (SAR) studies Small molecule libraries: - Natural products: Source: Plants, marine organisms, microorganisms Strengths: High stability, selectivity, and bioavailability Weaknesses: Difficult purification and synthesis; unknown targets - Synthetic small molecules: Strengths: High diversity, ease of synthesis, rapid SAR optimization Weaknesses: Limited chemical diversity and anthropomorphic bias - Lipinski's rule of 5: Predicts drug-like properties for oral bioavailability: ≤5 H-bond donors, ≤10 H-bond acceptors MW ≤500 Daltons, log P ≤5 Target identification struggles: - Process: Identifying the target protein of a small molecule and understanding its mechanism of action (MOA) Methods: Genetic or chemoproteomic approaches - Genetic Approaches: Resistance selection: Identify mutations conferring resistance to the drug CRISPR KO: Identify genetic loci consistently altered in mutants - Chemical Proteomic Approaches: Direct methods: Use covalent binding for target identification Indirect methods: Use competition assays with reversible molecules - Biorthogonal Reactions and Probes: Probes enable visualization and retrieval of target interactions Goal: Develop probes for every known target by 2035 (Target 2035 initiative) Target validation: - Confirmation: Ensures the target engagement and MOA correspond to the observed phenotype - Methods: Genetic knock-out/knock-down to mimic or alter drug effects Biochemical studies to determine how the drug-target interaction produces phenotypic effects Case Study: Sotorasib (Lumakras) - Drug development: Targeted K-RAS oncoproteins, previously untargetable Screened disulfide-containing molecules to identify effective candidates Outcome: Effective treatment for non-small cell lung cancer Glossary: - Target: Protein targeted by a small molecule - Small molecule: Small chemical compounds HTS: High-throughput screening - Target ID: Identifying the target of a small molecule - Target Validation: Confirming the target is relevant and engaged - Engagement: Interaction of a small molecule with its target - Translation: Applying in vitro findings to clinical settings - Mechanism of Action (MOA): Chemical/molecular activity mechanism Target validation: - Purpose: Confirm target engagement and phenotypic relevance - Approaches: Genetic Knock-Out: Phenotype should mimic drug effect; drug loses efficacy Genetic Knock-Down: Reduces drug's EC50 Biochemical: Confirms MOA Key questions: - Is the target phenotypically relevant? - Does drug-target interaction cause the observed phenotype? Steps in target validation: - Confirm drug hits target - Demonstrate phenotypic relevance of the target - Determine how drug-target interaction affects target function - Check if antimicrobial disrupts a known function of the target Challenges: - Easier validation for certain protein classes - Requires genetic and biochemical confirmation Antimicrobial Resistance and Mycobacterial Disease: Global tuberculosis: - Active TB: 10M cases/year; 1.5M deaths annually (\~1 death/25 sec) - Latent TB: \~2B infected; 10% lifetime risk of active disease, rising to 10% annually in HIV-positive individuals due to reduced CD4+ cells - UK reports \~4,000 new cases annually - Infection can be established by a single bacterium - Viral infections (e.g., HIV) increase TB risk and incidence Effects of COVID-19 on TB: - Global TB deaths increased sharply post-2020, reversing 20 years of progress (500K fewer deaths pre-COVID) - Co-infections with COVID-19 exacerbated TB mortality - Diagnostic and treatment disruptions due to COVID-19 focus led to undiagnosed cases, increased transmission, and later deaths - TB deaths rose in all regions from 2020--2022; Africa and South/Southeast Asia hit hardest - TB diagnoses decreased during COVID-19, allowing undetected cases to spread - Temporary spike in mortality in 2020; recovery to pre-pandemic trends remains slow - Of 1.5M TB deaths annually, 100K--300K involve drug-resistant TB - Treatment duration: 6 months for drug-sensitive TB, up to 3 years for drug-resistant TB Laboratory features of mycobacteria: - Aerobic/microaerophilic, non-motile bacilli: Can survive in low oxygen (\