Chemical Bonding and Structure 4 PDF
Document Details
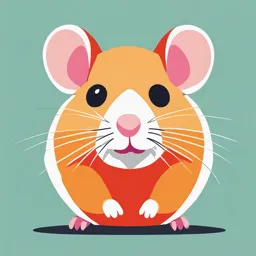
Uploaded by VirtuousAwareness1626
Tags
Summary
This document provides an overview of chemical bonding and structure, particularly focusing on ionic and covalent bonding. It outlines the formation of ions, types of compounds, and relationships in the periodic table regarding bonding properties. The structure includes learning objectives for the topic.
Full Transcript
Chemical bonding and structure 4 Bonding Compounds can be divided into two main classes according to the bonding in them – they are either ionic or covalent. The type of bonding present can usually be deduced by looking at the formula of the compound. Covalent compounds are those formed between t...
Chemical bonding and structure 4 Bonding Compounds can be divided into two main classes according to the bonding in them – they are either ionic or covalent. The type of bonding present can usually be deduced by looking at the formula of the compound. Covalent compounds are those formed between two or more non-metallic elements, whereas ionic compounds are usually formed between a metallic element and a non-metallic one. For example, NaCl is an ionic compound but CH4 is covalent. There are some compounds for which the distinction is not so clear. For instance, ammonium chloride does not contain any metallic elements but has ionic bonding between the ammonium ions (NH4+) and the chloride ions (Cl−). In addition to this, within the NH4+ ion there is covalent bonding. Generally, as a rough rule of thumb, elements that are close together in Ionic compound: metal and the periodic table form covalent compounds but elements that are far apart non-metal in the periodic table form ionic compounds. Thus elements from groups Covalent compound: two or more 1 and 17 combine to form ionic compounds (CsF being the most ionic) non-metals but elements from groups 14, 15, 16 and 17 combine to form covalent compounds. This is discussed in terms of electronegativity on page 129. 4.1 Ionic bonding and structure Learning objectives Positive ions are usually formed by metallic elements by the loss of valence (outer shell) electrons. For example, magnesium loses the two Recognise the formulas of ionic compounds electrons in its highest energy level (outer shell) to form a 2+ ion: Understand how ions are Mg → Mg2+ + 2e− formed and recall the formulas of some common ions Negative ions are usually formed by non-metallic elements by the gain of Work out the formulas of ionic electrons. For example, oxygen gains two electrons to fill up its outer shell compounds from the charges on (highest occupied energy level): the ions O + 2e− → O2− Describe the structure of sodium chloride as an example of an At the simplest level, when elements in the main groups of the periodic ionic lattice table (groups 1, 2, 13 (to a certain extent), 15, 16 and 17) form ions, Explain the physical properties electrons are gained or lost to achieve the electron configuration of the of ionic compounds in terms of nearest noble gas. That is, electrons are gained or lost to make an ion that structure and bonding is isoelectronic (same number of electrons) with the nearest noble gas. Another way of saying this is that electrons are lost or gained to achieve a full outer shell of electrons. Although this is true for the first 20 elements, it is not generally true after that because of the existence of transition metals (and d orbitals). 4 CHEMICAL BONDING AND STRUCTURE 119 The transition metals can form more than one ion. For instance, iron can form iron(II), Fe2+, and iron(III), Fe3+. An Fe2+ ion has 24 electrons, an Fe3+ ion has 23 electrons, and neither is isoelectronic with a noble gas. The 4s electrons are lost first when a transition metal atom forms an ion; therefore the electron configurations of Fe2+ and Fe3+ are: These do not have noble gas Fe2+ 1s22s22p63s23p63d6 electron configurations. Fe3+ 1s22s22p63s23p63d5 The number of electrons lost or gained is determined by the electron configuration of an atom. The number of electrons lost by elements in groups 1 and 2 when they form ions is given by the group number. For instance, magnesium, in group 2 of the periodic table, has two outer shell electrons, and therefore forms a 2+ ion (Figure 4.1). The number of electrons lost by elements in group 13 is given by the group number –10. For example, aluminium forms a 3+ ion. The number of electrons gained by elements in groups 15 to 17 when they form ions is given by 18 minus the group number. For instance oxygen, in group 16 of the periodic table, has six outer shell electrons and gains (18 − 16) = 2 electrons to form a 2− ion (Figure 4.2). 2 electrons Note: metal atoms do not ‘want’ removed to form ions with noble gas electron configurations – for 2+ instance, it takes the input of over 2000 kJ mol−1 of energy to remove Mg Mg two electrons from a magnesium atom to form an Mg2+ ion. Figure 4.1 The Mg2+ ion is isoelectronic with the noble gas atom neon. 2 electrons gained 2– O O Figure 4.2 The O2− ion is isoelectronic with the noble gas atom neon. 120 2 electrons transferred Mg O 2+ 2– Mg O 2+ Figure 4.3 Electron transfer in ionic bonding. Mg When an ionic compound is formed, electrons are transferred from one atom to another to form positive and negative ions. Electrons cannot – – be created or destroyed; therefore, the total number of electrons lost must always equal the total number gained.You can see this in Figure 4.3. F F In the formation of magnesium fluoride (Figure 4.4), one magnesium atom must combine with two fluorine atoms, because magnesium loses two electrons when it forms an ion but each fluorine atom can gain only one electron. So the formula of magnesium fluoride is MgF2 (Figure 4.4). Figure 4.4 The ions in magnesium fluoride. The formulas of ions The formulas of commonly encountered positive ions are given in Table 4.1 and of some negative ions in Table 4.2. Ion Symbol Ion Symbol Ion Symbol + 2+ lithium Li magnesium Mg iron(III) Fe3+ sodium Na+ calcium Ca2+ aluminium Al3+ potassium K+ barium Ba2+ rubidium Rb+ iron(II) Fe2+ caesium Cs+ copper(II) Cu2+ silver Ag+ zinc Zn2+ ammonium NH4+ nickel(II) Ni2+ hydrogen H+ Table 4.1 Positive ions. 4 CHEMICAL BONDING AND STRUCTURE 121 Ion Symbol Ion Symbol Ion Symbol fluoride F− oxide O2− nitride N3− chloride Cl− sulfide S2− phosphate(V) PO43− bromide Br− carbonate CO32− iodide I− sulfate(VI) SO42− hydroxide OH− hydrogencarbonate HCO3− nitrate(V) NO3− Table 4.2 Negative ions. Ions such as NO3– are most properly named including the oxidation number (i.e. nitrate(V)) but this is often omitted. Working out the formulas of ionic compounds Exam tip To work out the formula of aluminium fluoride, we need to consider The formulas and charges the number of electrons lost by aluminium and gained by fluorine. of ions such as sulfate and Aluminium is in group 13 of the periodic table and so forms a 3+ ion nitrate must be learnt, but the by the loss of three electrons, whereas fluorine, in group 17, gains one formulas of ions formed by electron to generate a full outer shell and a 1− ion. The three electrons elements in groups 1, 2, 13, 15, transferred from the aluminium must be gained by three separate fluorine 16 and 17 can be worked out. atoms, therefore the formula of aluminium fluoride is AlF3. Another way to look at this is to consider that the overall charge on the compound is zero, so the 3+ charge on the Al3+ ion must be cancelled out by the 3 × 1− charge on 3F− ions, i.e. Al3+(F−)3. Similarly, the formula of magnesium hydroxide is Mg(OH)2, where the The brackets around the OH are 2+ charge on the Mg2+ ion is cancelled out by the 2 × 1− charge on two essential because, otherwise the OH− ions, i.e. Mg2+(OH−)2. formula would read MgOH2, and A shortcut to working out formulas is to switch over the charges on would indicate the presence of the ions, for example: only one oxygen atom. 3+ 2– Al O Al2O3 Rubies and sapphires are mainly Transition metal ions can form more than one ion, so the oxidation aluminium oxide. number of the ion is usually given with the name. For example, iron can form iron(II) sulfate or iron(III) sulfate. The Roman numeral in brackets indicates the oxidation number of the ion, which is the same as its charge. Iron(III) sulfate, therefore, contains the Fe3+ ion and has the formula Fe2(SO4)3. Iron(II) sulfate contains the Fe2+ ion and has the formula FeSO4. The ionic bond and ionic crystals Electrostatic attraction: positive An ionic bond is an electrostatic attraction between oppositely charges attract negative charges and charged ions. vice versa. A crystal of sodium chloride consists of a giant lattice of Na+ and Cl− ions (Figure 4.5). All the Na+ ions in the crystal attract all the Cl− ions, 122 CI– Na+ a b c Figure 4.5 a A space-filling diagram of the NaCl lattice. The lattice keeps on going in three dimensions – only a tiny part of the structure is shown. b An expanded view of the NaCl lattice. c Each Na+ ion is surrounded by six Cl− ions in an octahedral array and vice versa. The coordination number (number of nearest neighbours) of each ion is 6. and vice versa, and it is this attraction between oppositely charged ions Extension that holds the crystal together. These electrostatic forces are strong, so it is This is only one possible lattice for difficult to break apart the lattice structure. ionic crystals. The type of lattice This is a giant structure – there are no individual molecules adopted depends on the formula of the of sodium chloride. Instead, the bonding extends fairly uniformly crystal and the ratio of the radii of the throughout the whole structure. positive and negative ions. It is often better to avoid the term ‘ionic bond’ and talk about ‘ionic bonding’ as a whole – the dotted lines in the diagrams of the lattice structures are not ionic bonds, they are simply there to give a better idea Examples of physical properties are of the shape. Ionic bonding (electrostatic attractions between oppositely melting point, solubility, electrical charged ions) extends throughout the whole structure in all directions – conductivity, etc. Chemical there are no individual ionic bonds. properties are how a substance reacts. Physical properties of ionic compounds The word ‘usually’ is used in the Melting points and boiling points sentence ‘ionic substances are usually solids at room temperature’ Ionic compounds usually have high melting points and boiling points. because there are some low- For instance, sodium chloride has a melting point of 801 °C and a boiling melting-point ionic compounds, point of over 1400 °C, while magnesium oxide has a melting point of such as ethylammonium over 2800 °C and a boiling point of about 3600 °C. This means that ionic nitrate, that are liquids at room substances are usually solids at room temperature. temperature (ionic liquids). The high melting and boiling points of ionic solids are due to the strong electrostatic forces between the oppositely charged ions. When an ionic solid is melted, the electrostatic forces throughout the giant lattice must be Extension broken and, because these are so strong, a lot of energy is required. Coulomb’s law – the force between Magnesium oxide has a much higher melting point than sodium two charges – is given by: chloride because the electrostatic attractions between the 2+ and 2− ions 1 QQ in the magnesium oxide lattice are much stronger than those between the F= × 12 2 4πεo r 1+ and 1− ions in sodium chloride. A higher temperature is required to provide sufficient energy to separate the ions in magnesium oxide. The where Q1 and Q2 are charges, r is the force between ions is proportional to the product of the charges (all other distance between the charges and εo is the electric permittivity of a vacuum. things being equal). 4 CHEMICAL BONDING AND STRUCTURE 123 Volatility Ionic solids have low volatility (refers to how readily a substance evaporates). The volatility of ionic substances is low because the electrostatic forces between the ions are strong. Electrical conductivity of ionic compounds Ionic substances do not conduct electricity when solid. In the solid state, Exam tip the ions are held tightly in position in the lattice structure so that they are It is important to be clear that not free to move around (other than vibrate). ions are moving, not electrons, When an ionic substance is melted the ions are able to move freely when an ionic substance throughout the liquid. Positive ions can move towards a negative electrode conducts electricity. and negative ions towards a positive electrode, so allowing the conduction of electricity. δ– Solubility in water δ+ O δ+ Ionic substances are often soluble in water. Water is a polar solvent, and a H H energy is released when the ions are hydrated by being surrounded (ion- dipole attractions) by water molecules (Figure 4.6). This energy pays back the energy required to break apart the ionic lattice. Electrical conductivity in ionic solutions Aqueous solutions (solutions made with water) of ionic substances conduct electricity. This is because the ions are free to move around (Figure 4.7). b Na+(aq) Solubility in non-polar solvents Ionic solids are not usually soluble in non-polar solvents such as hexane. This is because a great deal of energy is required to break apart the ionic lattice and this is not paid back by the energy released when the non- polar solvent forms interactions with the ions (London forces). This will be considered in more detail on page 157. c Cl–(aq) – + Figure 4.6 a A water molecule is polar. b A hydrated sodium ion. c A hydrated chloride ion. Cl– Cl– Na+ Na+ Na+ Cl– Cl– Cl– Na+ Na+ Na+ Na + Na+ Cl– Cl– Cl– + Na+ Na Na+ Na+ Cl– Cl– – Na+ Cl– Na+ Cl– Cl Figure 4.7 Ions move towards the oppositely charged electrode. 124 Nature of science Scientists use theories to explain and predict the properties of substances. We can use our theories of the bonding in ionic compounds to explain their properties, for instance, the facts that they have high melting points, conduct electricity when molten etc. Our theory of bonding in ionic substances predicts that all ionic substances have a common set of properties. Therefore we can predict the properties of any new ionic compound and use experimental observations to test the theory. ? Test yourself 1 State whether the following compounds have ionic or covalent bonding: a LiF b CF4 c CaO d NH3 e PCl3 f CuCl2 2 Write the formulas of the following compounds: a magnesium oxide g lithium nitride l silver sulfide b barium sulfate h magnesium phosphate m silver nitrate c calcium hydroxide i magnesium fluoride n ammonium chloride d sodium oxide j potassium sulfate o copper(II) nitrate e strontium sulfide k ammonium carbonate p rubidium carbonate f aluminium oxide 4.2 Covalent bonding Learning objectives Single covalent bonds Understand that a covalent bond is formed when electrons are shared Covalent bonding occurs when atoms share electrons, and a Understand the relationship covalent bond is the electrostatic attraction between a shared pair between bond strength and of electrons and the nuclei of the atoms that are bonded. bond length Understand what is meant by At the simplest level, electrons are shared to allow the atoms being bonded electronegativity to achieve a full outer shell of electrons (noble gas electron configuration). Predict whether a bond will be One example is the formation of methane (CH4). polar or not A carbon atom has four electrons in its outer shell (highest occupied energy level) (Figure 4.8). It will share four electrons so that the number of electrons in its outer shell is eight. H C + 4 H H C H hydrogen atom H carbon atom methane molecule Figure 4.8 The covalent bonding in CH4. 4 CHEMICAL BONDING AND STRUCTURE 125 A hydrogen atom has one electron in its outer shell and will share one electron with another atom (carbon in this case) to generate an outer shell containing two electrons, i.e. a full outer shell (the same number of electrons as helium). H In methane, the shared electrons are in the outer shell of both atoms H making up the bond and so each atom has a full outer shell of electrons H C H (Figure 4.8). H C H Two alternative ways of representing the covalent bonding in methane H H are shown in Figure 4.9. These are Lewis structures. Figure 4.9 Two different types of Lewis Consider the bonding in water (H2O) – an oxygen atom has six structure for methane. In the second one, the electrons in its outer shell and so will share two electrons to generate a full line between the atoms represents a shared outer shell (eight electrons) or an octet of electrons. The covalent bonding pair of electrons: that is, a covalent bond. in water is shown in Figure 4.10. lone pair lone pair 2 H + O O H hydrogen atom oxygen atom H O H O H H H Figure 4.10 Covalent bonding in water. All atoms have full outer shells of electrons. Figure 4.11 Lewis structures for water. The pairs of electrons in the outer shell of atoms that are not involved in the covalent bonds are called lone pairs of electrons. There are two lone pairs in the outer shell of the oxygen atom in a molecule of water. Alternative representations of the covalent bonding in water are shown in O O Figure 4.11. Multiple covalent bonds It is possible for more than one pair of electrons to be shared between two O O O O atoms. A double bond results from the sharing of two pairs of electrons, and Figure 4.12 Representations of the covalent a triple bond arises when three pairs of electrons are shared. For example, bonding in O2. the covalent bonding in an oxygen molecule, O2, is shown in Figure 4.12. Four electrons – that is, two pairs – are shared and so there is a double bond between oxygen atoms. The covalent bonding in the nitrogen molecule, N2, is shown in Figure 4.13. A nitrogen atom shares three electrons to generate a full outer shell, N N so six electrons are shared when two nitrogen atoms combine to form N2. Three shared pairs of electrons between two atoms form a triple bond. More examples of molecules with multiple bonding are shown in Figure 4.14. N N N N Figure 4.13 Representations of the covalent bonding in N2. 126 O C O O C O H C N H C N a b H H H H C C H C C H H C C H C C H H H H c d Figure 4.14 Covalent bonding in a CO2, b HCN, c C2H4 (ethene) and d C2H2 (ethyne). What holds the atoms together in a covalent bond? A covalent bond is the electrostatic interaction between the positively charged nuclei of both atoms and the shared pair of electrons. – The electrons are negatively charged and because the shared electrons are + + attracted to the nuclei (positively charged) of both atoms simultaneously, – this holds the atoms together (Figure 4.15). Figure 4.15 The positively charged nuclei As can be seen from Table 4.3, triple bonds are stronger than double attract the negatively charged electrons in the bond. bonds, which are stronger than a single bond. This is because the attraction of the two nuclei for three electron pairs (six electrons) in a triple bond is greater than the attraction for two electron pairs (four electrons) in a Strength double bond, which is greater than the attraction for one electron pair (two single bonds electrons) in a single bond (Figure 4.16). increasing double bonds It should also be noted that triple bonds are shorter than double strength triple bonds bonds, which are shorter than single bonds. This is, again, due to stronger attraction between the bonding electrons and the nuclei when there are more electrons in the bond. Bond Length / nm Bond energy / kJ mol−1 C–C 0.154 348 C=C 0.134 612 C C 0.120 837 C–O 0.143 360 C=O 0.122 743 Table 4.3 The relationship between number of bonds and bond length/strength. greatest attraction least attraction – electrons in bond – – – – – + – + > + – + > + – + – nucleus – nucleus – triple bond double bond single bond Figure 4.16 The more electrons that make up a covalent bond, the greater the attraction between the electrons and the nuclei, and therefore the stronger the bond. 4 CHEMICAL BONDING AND STRUCTURE 127 Group 14 Group 17 Bond Length / nm Energy / Bond Length / nm Energy / kJ mol−1 kJ mol−1 C–C 0.154 348 Cl–Cl 0.199 242 Si–Si 0.235 226 Br–Br 0.228 193 Ge–Ge 0.241 188 I–I 0.267 151 Table 4.4 The relationship between length of bonds and bond strength. In general, when we are comparing just single bonds, the longer the bond the weaker it is. Data for two groups in the periodic table are shown in Table 4.4. If we consider the data for group 14, it can be seen that the single bond between the elements gets weaker as the bond gets longer. This is because, as the atoms get bigger, the electron pair in the covalent bond is further away from the nuclei of the atoms making up the bond. If the electron pair Trends should really only be is further away from the nuclei, it is less strongly attracted and the covalent compared down a group because bond is weaker (Figure 4.17). A similar trend can be seen down group 17. elements in the same group have greater attraction the same number of outer shell electrons and therefore any effects – – due to effective nuclear charge or shielding are most similar. + + + + In general, comparisons such as – – this are most useful and valid a b less attraction when similar molecules, bonds or Figure 4.17 The bond in a is a shorter bond in which the bonding electrons are closer compounds are considered. to the nuclei than in b. Polarity Electronegativity In a covalent bond between two different atoms, the atoms do not attract the electron pair in the bond equally. How strongly the electrons are attracted depends on the size of the individual atoms and their nuclear charge. In an F2 molecule, the two fluorine atoms attract the electrons in the bond equally and so the electrons lie symmetrically (Figure 4.18). This molecule is non-polar. Electronegativity is a measure of the attraction of an atom in a F F F F + + electrons symmetrically molecule for the electron pair in distributed in covalent bond the covalent bond of which it is a b a part. Figure 4.18 a The electron density in F2; b F2 is a non-polar molecule. 128 δ− indicates a small negative H F H F H FH F charge. + + + + electrons lie, electrons on average, lie, on average, δ+ δ– closer to F closer to F H F a a b b c Figure 4.19 a The electron density in HF; b HF is a polar molecule; c usually represented like this However, in HF, fluorine is more electronegative than hydrogen and attracts the electrons in the H–F bond more strongly than the hydrogen atom does. The electrons in the bond lie closer to the fluorine than to the hydrogen (Figure 4.19) – H–F is a polar molecule. The unsymmetrical distribution of electron density results in small charges on the atoms. Fluorine is δ− because the electrons in the bond lie closer to F, whereas electron density has been pulled away from hydrogen, so it is δ+. Pauling electronegativities There are various scales of electronegativity and it is important to realise that, although they are derived from physical quantities (such as bond energies), the numbers themselves are not physical quantities – they have no units and must only be used in a comparative way. The most commonly used scale of electronegativity is that developed by Linus Pauling. The electronegativity values for some elements, worked out using the Pauling method, are shown in Table 4.5. Noble gases do not have electronegativity values because they do Non-metals have higher not form compounds. electronegativities than metals. H 2.1 Exam tip You do not need to learn Li Be B C N O F 1.0 1.5 2.0 2.5 3.0 3.5 4.0 these electronegativity values, but you should be aware of Na Mg Al Si P S Cl 0.9 1.2 1.5 1.8 2.1 2.5 3.0 trends and recognise highly electronegative atoms such as K Ca Ga Ge As Se Br 0.8 1.0 1.6 1.8 2.0 2.4 2.8 N, Cl, O and F. Rb Sr In Sn Sb Te I 0.8 1.0 1.7 1.8 1.9 2.1 2.5 Table 4.5 Pauling electronegativities for some elements. Exam tip To remember the trends in Electronegativity generally decreases down a group and increases across electronegativity, just remember a period. Trends in electronegativity were explained in Topic 3. that fluorine is the atom with the highest electronegativity. Atoms with similar electronegativities will form covalent bonds. Electronegativity must then Atoms with widely different electronegativities will form ionic increase across a period towards bonds. The difference in electronegativity can be taken as a guide fluorine and decrease down a to how ionic or how covalent the bond between two atoms is group from fluorine. likely to be. 4 CHEMICAL BONDING AND STRUCTURE 129 Linus Pauling related the electronegativity difference between two atoms to the ionic character of a bond. He suggested that an electronegativity Exam tip difference of 1.7 corresponded to 50% ionic character in a bond and If asked about this, assume reasoned that a higher electronegativity difference than this corresponded that the bonding between to a structure that was more ionic than covalent, whereas if the difference is two elements is ionic if the less than 1.7, the bonding is more covalent than ionic. This is a useful idea, difference is more than 1.7, but it must be used with great caution. For instance, KI (electronegativity and covalent if the difference is difference 1.7) would come out of this discussion as having 50% ionic and less than 1.7. 50% covalent character, and NaI (difference of 1.6) would appear to be mostly covalent, whereas both behave as predominantly ionic compounds. Pauling, in his original discussion of this, was actually referring to diatomic molecules and not to macroscopic compounds. ? Test yourself 3 Arrange the following atoms in order of electronegativity (lowest first): Cl O H Br Na Learning objectives 4.3 Covalent structures Understand what is meant by a The octet rule coordinate covalent bond Work out Lewis structures for In Subtopic 4.2 you met the concept that atoms in covalent bonds have molecules and ions a tendency to have a full valence shell with a total of eight electrons (or Work out the shapes of two in the case of hydrogen). This is known as the octet rule. In most molecules and ions with up to covalent molecules and polyatomic ions (for example, NH4+ or CO32−), four electron domains each atom has an octet in its outer shell. Predict bond angles in molecules It is not, however, always the case that the formation of covalent bonds and ions results in each atom attaining an octet of electrons. In BF3 (Figure 4.20), Predict whether a molecule will boron has only six electrons in its outer shell. This is because a boron be polar or non-polar atom has only three electrons in its outer shell and can therefore share a Describe the structures and maximum of three electrons. Similarly, in BeCl2 the beryllium atom only bonding of giant covalent has a total of 4 electrons in its outer shell. substances Explain the physical properties of giant covalent substances in F F F terms of structure and bonding B F F F F Figure 4.20 Covalent bonding in BF3. FF Figure 4.21 Covalent bonding in SF6. 130 It is also possible for atoms to have more than eight electrons in their outer shell. For instance, in SF6 (Figure 4.21) the sulfur atom has 12 Exam tip electrons in its outer shell, and this is described as sulfur having expanded its Expansion of the octet is very octet. Only elements in period 3 and beyond (periods 4, 5 …) can expand rarely encountered at Standard their octet. Expansion of the octet will be discussed in more detail in the Level. Higher Level section later (see Subtopic 4.6). Coordinate covalent bonds (dative covalent bonds) A coordinate covalent bond is a type of covalent bond in which both electrons come from the same atom. Once a coordinate covalent bond has been formed, it is identical to an ‘ordinary’ covalent bond. For example, NH4+ can be formed when H+ becomes bonded to NH3: NH3 + H+ → NH4+ + H+ does not have any electrons with which to form a covalent bond, but NH3 H H has a lone pair of electrons that can be used to form a covalent bond (Figure H N H+ H N H 4.22). H H A coordinate covalent bond is sometimes shown as an arrow (Figure coordinate coordinate 4.23a). Once it has been formed, a coordinate bond is, however, the same as covalent bond covalent bond any other covalent bond. The ammonium ion can be represented as shown in Figure 4.22 Coordinate covalent bonding Figure 4.23b, in which no distinction is made between the individual bonds. in NH4+. H3O+ is formed when a lone pair of electrons is donated from the O in + H + H2O to the H+: H + H O H+ H O H H N H H N H H H H H coordinate coordinate a b covalent bond covalent bond Figure 4.23 The ammonium ion, a with the coordinate bond shown and b with no NH3 and BF3 can combine to form an adduct (two molecules bonded distinction between the types of bonds. together): H F H F H N B F H N B F H F H F This is an example of a Lewis acid- base reaction (see Subtopic 8.2). coordinate coordinate covalent bond covalent bond In BF3 there are only six electrons in the outer shell of the boron atom – so there is space for the boron to accept a pair of electrons. Carbon monoxide Normally carbon shares four electrons to form four covalent bonds, and oxygen shares two to form two covalent bonds. If a carbon atom combines with an oxygen atom with the formation of two covalent 4 CHEMICAL BONDING AND STRUCTURE 131 bonds, we get the structure shown in Figure 4.24. However, in this C O structure, although the oxygen atom has a full outer shell (octet), the Figure 4.24 The structure of carbon monoxide if two ‘ordinary’ covalent bonds carbon atom has only six electrons in its outer shell. were formed. Both atoms can attain an octet if the oxygen atom donates a pair of electrons to carbon in the formation of a coordinate covalent bond. There C O is now a triple bond between the two atoms, made up of two ‘ordinary’ Figure 4.25 The structure of carbon covalent bonds and one coordinate covalent bond (Figure 4.25). Both monoxide if two ‘ordinary’ covalent bonds and atoms have a lone pair of electrons. one coordinate covalent bond were formed. Other ways of representing the bonding in carbon monoxide are shown in Figure 4.26. C O or C O or C O Figure 4.26 Other ways of showing the Coordinate covalent bonds are important in the formation of bonding in carbon monoxide. transition metal complexes. Lewis (electron dot) structures Lewis structures are diagrams showing all the valence (outer shell) Dots and crosses can be used in electrons in a molecule (or ion). Examples of Lewis structures are shown Lewis structures to indicate where in Figure 4.27. Electrons may be shown individually as dots or crosses, the electrons originally came from. or a line may be used to represent a pair of electrons, as in the Lewis structure of CO shown in Figure 4.27. Rules for working out Lewis structures: O H O C O C O 1 Make sure that the outer atoms have eight electrons in their outer shell H (except, of course, hydrogen which should have two). This is done by Figure 4.27 Lewis structures for H2O, CO2 and CO, showing all outer shell electrons. using single bonds, double bonds, triple bonds and + or − charges. 2 If the central atom is from period 2 it should have no more than eight electrons in its outer shell. It should generally (but not always) have a noble gas electron configuration. 3 If the central atom is from period 3 it may have up to 18 electrons in its outer shell. Note: the outer atom(s) is (are) Let us consider a few examples and go through the steps for drawing usually the more electronegative the Lewis structures. atom (except when hydrogen is also present in the molecule). NF3 The central atom is nitrogen and the three outer atoms are fluorine. A fluorine atom has seven electrons in its outer shell and therefore F only needs to form one single bond to have a full outer shell. Therefore, in order for each F atom to have eight electrons in its outer shell, three single bonds between the nitrogen and the fluorine must be formed. F F N F Figure 4.28 shows the Lewis structure for NF3. Each outer atom has eight electrons in the outer shell and the central atom also has eight Figure 4.28 The Lewis structure for NF3. electrons in its outer shell. All valence electrons are shown. 132 These individual structures differ only in the position of the double bond and are called resonance structures. In CO32−, the carbon– oxygen bonds are equal in length, in O3 the oxygen–oxygen bond lengths are equal and in benzene the carbon–carbon bonds are all identical. This cannot be explained by looking at just one of the structures (in which double bonds would be shorter than single bonds). The actual structure is described as a hybrid of the individual resonance structures. This is shown by the double headed arrow and is considered more fully in the Higher Level section below. Two possible Lewis structures for the same molecule SO2 Two different Lewis structures are possible for SO2 depending on whether the octet on S is expanded or not. Approach 1: Sulfur does not expand its octet a O S O Sulfur has six electrons in its outer shell and can, therefore, form a maximum of two normal covalent bonds. If these are both formed to the b O S O O S O same oxygen atom, we get the structure shown in Figure 4.34a. However, in this structure there is no bond between the sulfur and the right-hand c O S O O S O oxygen atom. The structure can be completed by a coordinate bond between the sulfur and the second oxygen, so that all atoms have eight Figure 4.34 Two possible Lewis structures for SO2. a Sulfur forms two covalent bonds electrons in their outer shell (Figure 4.34b). with one oxygen atom. b In the first Approach 2: approach, the structure is completed with a coordinate bond between sulfur and S expands its octet the second oxygen atom. c In the second Each oxygen atom can achieve an octet by forming a double bond. approach, sulfur expands its octet, forming A sulfur atom has six electrons in its outer shell and when it forms two a double bond with the second oxygen atom. double bonds it will have ten electrons in its outer shell and is said to have expanded its octet (Figure 4.34c). This is possible for elements in period 3 and beyond because they have d orbitals available for bonding – the maximum number of electrons in the third shell (the outer shell in sulfur) is 18. Both approaches to working out Lewis structures are valid, although more detailed considerations which involve working out the formal charge on each atom suggest that the second structure is a better representation of the bonding in the molecule (see the Higher Level section later). The bond lengths in SO2 also suggest the presence of two double bonds in the molecule. Alternative method for working out Lewis structures This approach is useful for working out the Lewis structures of molecules/ions just containing period 2 atoms. 1 Add up the total number of valence electrons of all the atoms in the molecule/ion. 2 Divide by two to get the total number of valence electron pairs. 3 Each pair of electrons is represented by a line. 134 4 Arrange the lines (electron pairs) so that all the atoms are joined together by at least single bonds and the outer atoms have full outer shells, i.e. are connected to four lines. 5 Rearrange the lines (electron pairs) so that every period 2 atom has four pairs of electrons. The outer atoms already have four pairs, so this should normally involve moving only lone pairs so that they become bonding pairs of electrons. NO3− total no. electrons = 5 + 3 × 6 + 1 = 24e− N 3 × O negative charge 24 There are therefore = 12 pairs of electrons, shown here by 12 lines. 2 Three must be now used to join all the atoms together: O O N O This leaves nine lines (electron pairs) that will be distributed as lone pairs of electrons on the O atoms (as O is the outer atom) to give each an octet. O O N O Now each O is ‘attached to four lines’ (has four pairs of electrons in its outer shell) and all the electrons have been used. The nitrogen, however, has only three pairs of electrons in its outer shell, and one of the lines needs to be moved from being a lone pair on the O to make a double bond between the N and an O. This does not change the number of electrons in the outer shell of the O but will increase the number of electrons in the outer shell of the N to eight. O – O N O O The final Lewis structure for the NO3− ion is shown in Figure 4.35. O N O As with CO32− earlier (with which it is isoelectronic), more than one Figure 4.35 The Lewis structure for NO3−. resonance structure can be drawn with the double bond in different positions. – This is a purely mechanical technique for working out the Lewis O structure and does not really involve any understanding of the bonding in O N O the ion. The Lewis structure showing the electrons as dots and crosses is Figure 4.36 The Lewis structure of NO3− probably clearer (Figure 4.36). with the electrons shown individually. 4 CHEMICAL BONDING AND STRUCTURE 135 NO2− total no. electrons = 5 + 2 × 6 + 1 = 18e− N 2 × O negative charge 18 There are therefore = 9 pairs of electrons 2 Exam tip NO2 has been asked about in examinations. It has an The atoms are joined together using two lines: odd number of electrons and therefore an unpaired O N O electron. The Lewis structure More lines are added to the outer atoms to give each an octet: can be shown with an N=O double bond and an N → O O N O coordinate bond. The unpaired So far, only eight lines (electron pairs) have been used and the last line electron would then be on must be put as a lone pair on the N. It cannot be put anywhere else, as the the N. Os already have four electron pairs each. O N O – O N O N only has three electron pairs and so a lone pair is moved from an O to Figure 4.37 The Lewis structure for NO2−. form a double bond: O N O – O N O This results in the Lewis structure for NO2− shown in Figure 4.37. Figure 4.38 An alternative resonance It is important to note that the Lewis structure could be drawn the structure for NO2−. other way round and that this is entirely equivalent (Figure 4.38). O3 Ozone is isoelectronic with NO2− and has the same Lewis structure: O O O + + NO2+ and CO2 ON O O N O NO2+ and CO2 are isoelectronic and have the same Lewis structures (Figures 4.39 and 4.40). Figure 4.39 The Lewis structures for NO2+. This technique for drawing Lewis structures is useful for molecules/ ions involving just period 2 elements, but it also works for compounds O C O O C O involving period 3 elements (and below) if the idea that the atoms do not expand their octet (approach 1, above) is adopted. Figure 4.40 The Lewis structures for CO2. Nature of science Scientists often make careful observations and look for patterns and trends in data, however, noticing discrepancies in these trends can lead to advances in knowledge. For instance, the observation that compounds containing metallic elements had different properties to those containing just non-metallic elements led to the idea of different types of structure and bonding. 136 Lewis introduced the idea of atoms sharing electrons and distinguished between compounds with ionic and covalent bonding (although he did not originally call them that). He also considered the unequal sharing of electrons and polar compounds. Linus Pauling built on this work by developing the concept of electronegativity to quantify, to a certain extent, the unequal sharing of electrons. ? Test yourself 4 Work out Lewis structures for the following: 5 Some harder ones – these are likely only to be a H2S d COF2 g FNO j PCl4+ encountered at Higher Level: b PCl3 e HCN h N2H4 k NO+ a XeF4 c BrF5 e N2O g I3− c CCl4 f CS2 i H2O2 l OCN− b PCl6 − d ClF3 f SF4 h N3− Shapes of molecules: valence shell electron pair repulsion theory We can predict the shapes of molecules using the valence shell electron pair repulsion (VSEPR) theory. Pairs of electrons (electron domains) in the valence (outer) shell of an atom repel each other and will therefore take up positions in space to minimise these repulsions – to be as far apart in space as possible. The pairs of electrons may be either non-bonding pairs (lone pairs) or bonding pairs (pairs of electrons involved in covalent bonds). Basic shapes More precisely, it is a question of The shape of a molecule depends on the number of electron pairs in how points can be arranged on the the outer shell of the central atom. There are five basic shapes (for two surface of a sphere to be as far away to six electron pairs), which are derived from the idea of how a number from each other as possible. of things, joined to a central point, can be arranged in space to be as far apart from each other as possible. However, first of all we will just consider molecules with up to four pairs of electrons around the central atom. The A double bond is made up of basic shapes and bond angles are shown in Table 4.6. two pairs of electrons, but these electron pairs are constrained to No. electron Shape Diagram Bond angle Example occupy the same region of space. domains A double bond (or a triple bond) therefore behaves, in terms of 2 linear Y X Y BeCl2(g) 180° repulsion, as if it were just one 3 trigonal planar Y Y BF3 electron pair and so it is better to X 120° talk about the number of electron Y domains – where an electron 4 tetrahedral Y 109.5° CH4 domain is either a lone pair, the electron pair that makes up a single Y X bond or the electrons pairs that Y Y together make up a multiple bond. Table 4.6 Basic molecule shapes and bond angles. 4 CHEMICAL BONDING AND STRUCTURE 137 How to predict the shapes of molecules 1 Draw a Lewis structure for the molecule or ion. 2 Count up the number of electron pairs (bonding pairs and lone pairs) in the outer shell of the central atom. A multiple bond counts as a single electron pair, because the electrons are constrained to occupy the same region of space. This gives the total number of electron domains. a b 3 Look at Table 4.7 to get the basic shape (spatial arrangement of the Figure 4.41 a This symbol indicates a electron domains). bond coming out of a plane. b This symbol 4 A lone pair is just an electron pair in the outer shell of an atom and, indicates a bond going into a plane. as such, it contributes to the overall shape of the molecule but cannot itself be ‘seen’. 5 State the actual shape of the molecule. See Figure 4.41 for how to draw 3D shapes. H CH4 H C H The Lewis structure for CH4 is shown in Figure 4.42. The number of H electron pairs in the outer shell of the central atom (C) is four, i.e. there Figure 4.42 The Lewis structure for CH4. are four electron domains. These four electron domains repel each other and take up positions in space as far away from each other as possible. The H shape that allows four things to be as far away from each other as possible 109.5° is tetrahedral. Therefore, the four electron pairs (electron domains) are H C H arranged tetrahedrally around the C atom. The shape of the methane H molecule is tetrahedral and the H–C–H bond angle is 109.5° (Figure 4.43). Figure 4.43 CH4 is tetrahedral. NH3 The Lewis structure for NH3 is shown in Figure 4.44. Bonding pairs of electrons: 3 (in three single bonds) Non-bonding pairs of electrons: 1 (in one lone pair) H Electron domains: 4 H N H Because these four electron domains repel each other and take up Figure 4.44 The Lewis structure for NH3. positions in space to be as far apart as possible, the electron pairs are distributed in a tetrahedral arrangement. The basic shape is tetrahedral – but a lone pair is just a pair of electrons in the outer shell of the central H N atom and, although it repels the other pairs of electrons and influences H H 107.3° the shape, it cannot be ‘seen’. The shape adopted by the atoms is therefore Figure 4.45 The shape of the NH3 molecule trigonal pyramidal (a pyramid with a triangular base) (Figure 4.45). is based on a tetrahedron, but the actual The H–N–H bond angle here is smaller than in a perfect tetrahedron, shape is trigonal pyramidal. because the lone pair repels the bonding pairs of electrons more than they repel each other. This will be considered in more detail later. 138 Here, we talk about it not being possible to pairs of electrons cannot be detected by this technique. ‘see’ a lone pair of electrons, but of course it Do we know or believe the shapes of molecules stated is not possible to see any of these molecules. here? Which ways of knowing do we use to interact There are various techniques for determining the with this microscopic world? In biology, microscopes shapes of molecules experimentally. Probably the are used to ‘view’ things that are too small to be seen most important of these is X-ray crystallography, in with the naked eye. Is there a difference between the which a crystal of the substance is placed in an X-ray use of a microscope to interact with this invisible world diffractometer and, from the position and intensity of and the use of an X-ray diffractometer? What about the diffracted X-ray beams, the shape of the molecules, all use of electron microscopes? the angles and bond lengths can be calculated. The lone CO2 O C O The Lewis structure for CO2 is shown in Figure 4.46. Figure 4.46 The Lewis structure for CO2. Bonding pairs of electrons: 4 (in two double bonds) Non-bonding pairs of electrons: 0 Electron domains: 2 (because a double bond counts as one electron domain – the four electrons that make up the double bond are constrained to occupy the same region of space) O C O Because these two electron domains repel each other and take up positions in space to be as far apart as possible, the electron domains are 180° distributed in a linear arrangement (Figure 4.47), and the shape adopted Figure 4.47 The linear CO2 molecule. by the atoms is linear. SO2 O S O The Lewis structure for SO2 is shown in Figure 4.48. Figure 4.48 The Lewis structure for SO2. Bonding pairs of electrons: 4 (in two double bonds) Non-bonding pairs of electrons: 1 (one lone pair on S) Electron domains: 3 (because a double bond counts as one electron domain) S Because these three electron domains repel each other and take up O O 119° positions in space to be as far apart as possible, the electron domains are a distributed in a trigonal planar (flat triangle) arrangement (Figure 4.49a). The lone pair on sulfur is just a pair of electrons in its outer shell and S cannot be ‘seen’. The shape adopted by the atoms is therefore bent (also O O 119° called ‘angular’, or ‘V-shaped’). b Again the bond angle is slightly less than the ideal angle in a trigonal Figure 4.49 a The bent SO2 molecule planar structure (120°), and this results from the extra repulsion of a lone b alternative model for SO2. pair, so that the bonding pairs are pushed closer together and the bond angle is reduced. If the Lewis structure had been drawn differently (Figure 4.49b), with sulfur not expanding its octet, this would have made no difference to the predicted shape. 4 CHEMICAL BONDING AND STRUCTURE 139 Lone pairs and bond angles The order of repulsion strength for pairs of electrons is: smaller distance H N larger H lone pair–lone pair > lone pair–bonding pair > bonding pair–bonding pair H distance Figure 4.50 Lone pairs lie closer to the central nucleus than bonding pairs. This is because lone pairs are held closer (Figure 4.50) to the central nucleus than are bonding pairs (lone pairs are in the outer shell of the central atom, whereas a bonding pair can be imagined as being, on Exam tip average, halfway between the bonded atoms). The lone pairs are thus A general rule of thumb, if you closer to the bonding pairs of electrons than the bonding pairs are to each are asked to predict a bond other and repel them more strongly. This means that the repulsion due to angle in a particular molecule, lone pairs causes other bond angles to become smaller. just take two or three degrees Consider CH4, NH3 and H2O, each of which has four electron pairs off the basic angle (the bond in the outer shell of the central atom (Table 4.7).The basic shape is the angle in the basic shape) for arrangement of the electron pairs in the outer shell of the central atom. each lone pair present on the The more lone pairs present, the smaller the H–X–H bond angle. This central atom. For example, is due to greater repulsion from lone pairs than from bonding pairs of a bent molecule based on a electrons. Two lone pairs cause greater repulsion than one, so the bond trigonal planar structure (one angle gets smaller as the number of lone pairs increases. lone pair on the central atom) could have a bond angle of Molecule Lewis Bonding Lone Basic Actual shape H–X–H bond 120 − 3 = 117°. There is no structure pairs pairs shape angle scientific basis for doing this, but it is useful for answering H examination questions. CH4 H C H 4 0 tetrahedral 109.5° H tetrahedral H Note: both SO2 and H2O have bent trigonal NH3 H N 3 1 pyramidal 107.3° structures, but the SO2 structure is H based on trigonal planar whereas the H2O structure is based on H2O O H 2 2 bent 104.5° tetrahedral. This means that the H bond angle is larger in SO2. Table 4.7 Structural characteristics of CH4, NH3 and H2O. + Predicting the shapes of ions H H N H The approach to predicting the shapes of ions is exactly the same as for neutral molecules. H Figure 4.51 The Lewis structure for NH4+. NH4+ The Lewis structure for NH4+ is shown in Figure 4.51. + Bonding pairs of electrons: 4 H Non-bonding pairs of electrons: 0 109.5° N Electron domains: 4 H H H Because these four electron domains repel each other and take up positions in space to be as far apart as possible (Figure 4.52), the electron Figure 4.52 Tetrahedral NH4+. pairs are distributed in a tetrahedral arrangement. 140 H3O+ + The Lewis structure for H3O+ is shown in Figure 4.53. H O H Bonding pairs of electrons: 3 H Non-bonding pairs of electrons: 1 Figure 4.53 The Lewis structure for H3O+. Electron domains: 4 + H O Because these four electron domains repel each other and take up H positions in space to be as far apart as possible, the electron pairs are H distributed in a tetrahedral arrangement. One of the electron pairs is Figure 4.54 Trigonal pyramidal H3O+. a lone pair, so the actual shape of the ion is trigonal pyramidal (Figure 4.54). This structure is based on tetrahedral (bond angle 109.5°) with one No definitive value for the bond lone pair, so a bond angle of about 107° for the H–O–H bond could be angle in H3O+ exists.Values have predicted (the molecule is isoelectronic with ammonia). been measured, but they depend NO2− on the actual compound i.e. the The Lewis structure for NO2− is shown in Figure 4.55. negative ion present. Bonding pairs of electrons: 3 (one single bond and one double bond) Non-bonding pairs of electrons: 1 – O N O Electron domains: 3 (because a double bond counts as one electron domain) Figure 4.55 The Lewis structure for NO2−. Because these three electron domains repel each other and take up – positions in space to be as far apart as possible, the electrons pairs are N distributed in a trigonal planar arrangement. One of the electron pairs O O is a lone pair, so the actual shape of the ion is bent (Figure 4.56). This Figure 4.56 NO2− is bent. structure is based on trigonal planar (bond angle 120°) with one lone pair, so a bond angle of about 117° could be predicted. No definitive value for the bond angle in NO2− exists.Values have Molecules with more than one central atom been measured, but they depend The approach to predicting the shapes of molecules with more than one on the actual compound. central atom is the same as for other molecules, except that each ‘central atom’ must be considered separately. N2H4 H H The Lewis structure for N2H4 is shown in Figure 4.57. In this molecule, N N the two N atoms are ‘central atoms’ and each one must be considered H H separately (Table 4.8). Figure 4.57 The Lewis structure for N2H4. Left-hand nitrogen Right-hand nitrogen bonding pairs of electrons: 3 bonding pairs of electrons: 3 non-bonding pairs of electrons: 1 non-bonding pairs of electrons: 1 electron domains: 4 electron domains: 4 Table 4.8 Counting electron domains around the nitrogen atoms in N2H4. N H H N It can be seen (Figure 4.58) that the arrangement of electron pairs H around each nitrogen is tetrahedral and, with one lone pair on each H nitrogen, the shape about each N atom is trigonal pyramidal. Figure 4.58 The structure of N2H4. 4 CHEMICAL BONDING AND STRUCTURE 141 C2H2 The Lewis structure for C2H2 is shown in Figure 4.59. In ethyne, each C H C C H is considered separately, but each one is identical. For each C: Figure 4.59 The Lewis structure for C2H2. Bonding pairs of electrons: 4 (one single bond and one triple bond) Non-bonding pairs of electrons: 0 H C C H Electron domains: 2 180° 180° The triple bond counts as one electron domain, so, with two electron domains around each C, the shape is linear (Figure 4.60) about each C Figure 4.60 Linear C2H2. and therefore linear overall. ‘Basic shape’ is the arrangement VSEPR summary of the electron pairs around the The shapes taken up by molecules depend on the number of pairs of central atom. electrons in the outer shell of the central atom. To take account of molecules containing multiple bonds, this is often expressed in terms of electron domains, where one multiple bond counts as one electron domain. Extension Pairs of electrons in the outer shell of an atom repel each other. The VSEPR gives us no information about pairs of electrons may be either bonding pairs or lone pairs. The pairs of how the two NH2 groups in hydrazine electrons take up positions in space to minimise repulsions in a molecule, are twisted relative to each other, and i.e. to be as far apart from each other as possible. The basic shapes adopted in the gas phase hydrazine adopts are shown in Table 4.9. the conformation with the two NH2 Lone pairs influence the shapes of molecules but cannot actually be groups staggered relative to each other. ‘seen’. The shapes of some specific molecules are also shown in Table 4.9. H Lone pairs repel bonding pairs of electrons more than the bonding pairs N repel each other. Therefore the presence of lone pairs results in a closing H H up of bond angles. H view of a hydrazine molecule looking down the N—N bond Total electron Bonding Lone Basic shape Actual shape Example domains pairs pairs 2 2 0 linear linear CO2, NO2+, HCN, BeCl2 3 3 0 trigonal planar trigonal planar BF3, SO3, NO3−, CO32− 3 2 1 trigonal planar bent, V-shaped, angular SO2, O3, NO2− 4 4 0 tetrahedral tetrahedral CCl4, XeO4, NH4+, BCl4−, SO42−, PO43− 4 3 1 tetrahedral trigonal pyramidal NH3, PCl3, XeO3, H3O+, ClO3−, SOCl2 4 2 2 tetrahedral bent, V-shaped, angular H2O, SCl2, ClF2+, I3+ Table 4.9 The basic and actual shapes of some specific molecules – see Subtopic 4.6 for information about atoms with more than four electron domains. 142 Polar molecules The electronegativity difference between two atoms covalently bonded dipole moment positive negative together results in the electrons lying more towards one atom than the other. We call such a bond polar. However, whether an overall molecule is δ– polar also depends on the shape of the molecule. δ+ δ– δ+ δ– δ+ δ+ O δ+ H Cl H N H H H For a molecule to be polar it must have a positive end to the molecule and a negative end. For instance HCl, NH3 and H2O are all polar (Figure H δ+ 4.61). These molecules all have an overall dipole moment, and the arrow Figure 4.61 These molecules are all polar – one end of the molecule is slightly positive indicates the direction of the moment. compared with the other end. The arrow indicates the dipole moment direction. Although individual bonds may be polar, a molecule may be non- polar overall if, because of the symmetry of the molecule, the dipole moments of the individual bonds cancel out. CO2 is a non-polar molecule (Figure 4.62). Each C=O bond is polar, δ– δ+ δ– δ– δ– O C O F δ+ F because oxygen is more electronegative than carbon, but overall the B dipoles cancel so that there is no overall dipole moment and the molecule dipoles cancel is non-polar.