Lecture 76: Pharmacology - Metabolic Energetics & Drug Metabolism in Kidneys PDF
Document Details
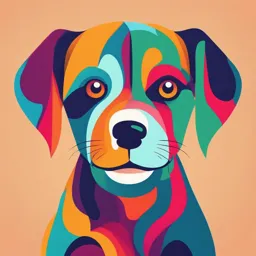
Uploaded by FruitfulIntegral
Wayne State University
Tags
Summary
This document provides an overview of pharmacology and metabolic energetics in the kidneys. It details the role of kidneys in drug metabolism and examines substrate supply in different kidney regions. Key processes such as glomerular filtration, reabsorption, and secretion are highlighted.
Full Transcript
Lecture 76: Pharmacology - Metabolic Energetics & Drug Metabolism in Kidneys Substrate supplies & respiratory rates in different kidney regions: Why kidney energetics are important: kidneys have high O2 consumption (2nd to heart), high blood flow, are particularly susceptible to hypoxic injury, rena...
Lecture 76: Pharmacology - Metabolic Energetics & Drug Metabolism in Kidneys Substrate supplies & respiratory rates in different kidney regions: Why kidney energetics are important: kidneys have high O2 consumption (2nd to heart), high blood flow, are particularly susceptible to hypoxic injury, renal mitochondrial supply lots of ATP Dependence on ATP: renal Na+ pump (“pacemaker” for cellular respiration), tissue-specific synthesis of key proteins (erythropoietin, vitamin D receptor), kidney can be gluconeogenic Role of kidneys in drug metabolism: many drugs (especially ionic ones) undergo renal excretion (impacts efficacy & toxicity), significant drug metabolism activity (especially in proximal tubules) Key processes: glomerular filtration, reabsorption, secretion (all depend on membrane transport) Transporter competition: b/w drugs can lead to drug-drug interactions, b/w drugs & endogenous metabolites can lead to acute kidney injury (AKI) Substrate supply in different kidney regions: PCT doesn’t metabolize glucose, TALH & DCT have high capacity for glucose (& lactate) oxidation, collecting tubules have highest capacity for aerobic glycolysis Drug metabolism & metabolic heterogeneity in different nephron cell types: Gluconeogenesis: enzymes found in PCT (most) & PST, but none in distal nephron segments Na+/K+ ATPase: highest in DCT, TALH, & PCT; lowest in thin limb; intermediate in collecting duct & PST Mitochondrial density: highest in PCT & mTALH (proximal segments = most transport activity) Drug metabolism: mostly in proximal tubules (includes all major enzymes of phase I & phase II metabolism), but selective localization in other nephron segments HETEs & EETs: derived from action of CYPs on arachidonic acid (AA) released by phospholipase A2 (cPLA2) acton on PM lipids 20-HETE: ↑TGF, ↑PKD, ↓IR injury, ↑natriuresis, ↓HTN, ↓glomerular injury, ↑RBF autoregulation EETs: ↑natriuresis, ↓inflammation, ↓HTN, ↓glomerular injury CYPs: important in drug metabolism but also in metabolism of endogenous chemicals Redox balance & renal mitochondrial function: ROS → breaks in mitochondrial DNA (mtDNA) → aberrant mitochondrial proteins → impaired mitochondrial function → further ↑ROS Renal mitochondria: targets for & sources of reactive oxygen species (ROS) Insults: substances (eg. toxic chemicals, reactive metabolites of drugs) that can increase the production of ROS in cytoplasm & mitochondria NADPH oxidase (NOX2 & NOX4): can contribute to the production of ROS Nuclear factor erythroid 2-related factor 2 (NRF2): activated by ROS → binds antioxidant-responsive elements (AREs) in nucleus → activate transcription of genes encoding antioxidant enzymes Mitochondrial superoxide dismutase 2 (SOD2): reduces superoxide anions to H2O2 & H2O Glutathione peroxidase (GPX): oxidizes glutathione (GSH) → results in glutathione disulfide (GSSG) as a byproduct of reducing H2O2 to H2O → mitochondrial GSSG (mGSSG) converted back to GSH by glutathione reductase (GR), requires NADPH Mitochondrial uncoupling protein 2 (UCP2): activity increased in response to ROS breakdown, dissipates proton motive force & decreases ROS production Mitochondrial biogenesis as a pharmacological target: Mitochondrial homeostasis: mitochondria adapt to different metabolic conditions by regulation of mechanistic/mammalian target of rapamycin (mTOR) & AMP-activated protein kinase (AMPK) pathways Fission: the splitting of mitochondria in 2; isolates damaged mitochondria from the mitochondrial network, allowing damaged mitochondria to be targeted for mitophagy Fusion: the combining of 2 mitochondria; leads to the elongation of mitochondrial under physiological conditions, helping to maintain oxidative phosphorylation (Oxphos) Mitophagy: a process by which mitochondria are degraded Mitochondrial biogenesis: activation of peroxisome proliferator-activated receptor γ-coactivator 1⍺ (PGC-1⍺) → translocation to nucleus initiates translation of various genes, including that for mitochondrial transcription factor A (TFAM) → protein products for Oxphos, TCA cycle, & mitochondrial biogenesis Activation of GPCRs (eg. β2-adrenergic receptors, β2AR & 5-hydroxytryptamine receptor 1F, 5-HT1F) → dissociation of G protein subunits → activation of PKA & endothelial nitric oxide synthase (eNOS) → eNOS stimulates soluble guanylate cyclase (sGC) to form cGMP (can be converted to GMP by 3’,5’-cyclic phosphodiesterase, PDE5)→ cGMP activates PGC-1⍺ Chemicals: can activate nuclear receptors to induce mitochondrial biogenesis; eg. peroxisome- proliferator-activated receptors (PPARs) & estrogen-related receptors (ERRs) Other transcription factors: can directly act on PGC-1⍺ to induce mitochondrial biogenesis; eg. nuclear respiratory factor 1 (NRF1) & NRF2 Other stimuli: can activate eNOS → ↑cGMP → activates PGC-1⍺; eg. caloric restriction mTOR: serine-threonine kinase complexes, inhibited by hypoxia & AMPK mTORC1: acts as a nutrient sensor (activated by growth factors, nutrients, & oxidative stress) regulating cell growth & proliferation (↑protein, lipid, & nucleotide synthesis & mitochondrial biogenesis) & inhibiting autophagy by stimulating anabolic processes via activation of PGC-1⍺ mTORC2: believed to regulate K+ & Na+ levels in the kidneys AMPK: acts as a nutrient sensor in the kidneys & stimulates catabolic (degradative) processes via phosphorylation of several proteins (leads to production of antioxidant enzymes, induction of mitochondrial biogenesis via phosphorylation of PGC-1⍺, and ↑glycolysis, FA oxidation, & glucose transport); activated by high AMP:ATP & low [O2], also acts by inhibiting mTORC1 Sirtuins: a family of 7 NAD+-dependent deacetylases (stimulated by ↑NAD+:NADH) that regulate histone proteins at specific lysine residues, promoting post-translational modification that results in chromatin silencing & transcriptional repression SIRT1: acts as a positive transcriptional regulator of PGC-1⍺ (promotes mitochondrial biogenesis & Oxphos) through promoter deacetylation; activated by resveratrol (a stilbenoid found in red wine); inhibits TNF⍺-dependent transactivation of NF-kB, limiting the expression of proinflammatory genes; responds to DNA damage/oxidative stress by deacetylation of p53 & FoxO transcription factors → ↓cell apoptosis & senescence; regulates expression of hypoxia-inducible factor-2⍺ (responsible for induction of erythropoietin by renal cells); represses genes regulated by PPAR-γ after food withdrawal SIRT3: major regulator of the organelle acetylome; binds ETC complexes to boost ATP; major regulator of mitochondrial antioxidant pathways by activation of SOD2, isocitrate DH 2 & deacetylation of acetyl-CoA synthase 2, & glutamate DH (fuels urea cycle); also promotes β-oxidation (by driving long-chain acyl CoA DH activity) & ketone body generation (by promoting deacetylation of 3-hydroxy-3-methylglutaryl CoA synthase 2) SIRT4: predominantly an ADP-ribosylase that has opposite effects of SIRT 3 (inactivates enzymes of urea cycle & β-oxidation) but induces lipogenesis through malonyl CoA decarboxylase deacetylation; governs cellular response to DNA damage via glutamine metabolism inhibition (potential role as tumor suppressor) SIRT5: mitochondrial deacetylase that regulates the urea cycle through direct activation of carbamoyl phosphate synthetase 1; also exhibits demalonylase & desuccinylase activities (controls ketogenesis); induces energetic flux via glycolysis Aging & kidney function: Nephron loss: kidney loses nephrons & mass → remaining nephrons undergo hypertrophic adaptation Cellular senescence: permanent cell cycle arrest after cumulative damage (telomere shortening, DNA damage, ROS) derived through the p53 or p16INK4a pathway Cellular hypertrophy: associated w/ interstitial fibrosis, tubular atrophy, glomerulosclerosis (GS), & microvascular rarefaction; podocyte hypertrophy is believed to drive GS Immunological & inflammatory responses: senescence-associated secretory phenotype (SASP) associate w/ inflammatory factors which act in a paracrine fashion, recruiting immune cells which destroy the microenvironment & altering the function of neighboring cells