Fluorescence Spectroscopy PDF
Document Details
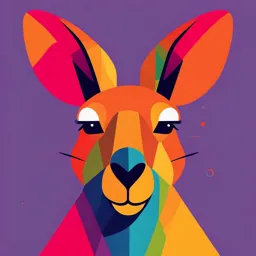
Uploaded by DazzlingFreedom
UP College of Medicine
Tags
Related
- 2022-23 Week 28 BIOL25012 Fluorescence and FRET PDF
- 2022-23 Week 29 BIOL25012 Lasers and Fluorescence - Nottingham Trent University PDF
- Lecture 3 - Tan Bioanalytical Techniques PDF
- Emerging Methods for Structural Analysis of Protein Aggregation PDF 2017
- Fluorescence Spectroscopy - BIOC3570 Notes PDF
- BIOT6002 Lecture 17 Data Handling 5 PDF
Summary
These lecture notes provide an overview of fluorescence spectroscopy in biochemistry. The document explains concepts including luminescence, different types of fluorescence, various phenomena related to the process, and methods such as fluorescence correlation spectroscopy and single molecule detection. Supplementary diagrams and figures enhance understanding.
Full Transcript
Fluorescence Spectroscopy BIOCHEM 125 Lecture Fluorescence • Luminescence – emission of light from any substance • Fluorescence • Phosphorescence Fluorescence Fluorescence Spectral Data • Fluorescence emission spectrum is plotted as fluorescence intensity versus wavelength (𝝺) or wavenumber (c...
Fluorescence Spectroscopy BIOCHEM 125 Lecture Fluorescence • Luminescence – emission of light from any substance • Fluorescence • Phosphorescence Fluorescence Fluorescence Spectral Data • Fluorescence emission spectrum is plotted as fluorescence intensity versus wavelength (𝝺) or wavenumber (cm–1). Fluorescence Spectral Data • Fluorescence emission spectrum is plotted as fluorescence intensity versus wavelength (𝝺) or wavenumber (cm–1). Fluorescence Spectral Data • The Franck-Condon Principle • The electrons undergoing the energetic transition is massless compared to the nuclei and the process takes place fast – the nuclei does not have time to adjust – the adjustment takes place after the transition The Stokes Shift • Fluorescence typically occurs at lower energies or longer wavelengths. This is known as the Stokes shift. The Stokes Shift • Fluorescence typically occurs at lower energies or longer wavelengths. This is known as the Stokes shift. • Internal conversion • Decay to higher vibrational energy levels of S0 • Solvent effects • Excited-state reactions • Complex formation • Energy transfer Kasha’s Rule • The same fluorescence spectrum is generally observed irrespective of the excitation wavelength. Kasha’s Rule • The same fluorescence spectrum is generally observed irrespective of the excitation wavelength. • Fluorophores that exist in two ionization states with two different absorption and emission spectra • Molecules that emit from the S2 level Kasha’s Rule • The same fluorescence spectrum is generally observed irrespective of the excitation wavelength. Symmetry of Absorbance and Emission Spectra • The generally symmetric nature of these spectra is a result of the same transitions being involved in both absorption and emission, and the similar vibrational energy levels of S0 and S1. Quantum Yield and Lifetime • Quantum yield – number of emitted photons relative to the number of absorbed photons • Lifetime determines the time available for the fluorophore to interact with or diffuse in its environment Quantum Yield and Lifetime • Quantum yield – number of emitted photons relative to the number of absorbed photons • Lifetime determines the time available for the fluorophore to interact with or diffuse in its environment 𝞒: emissive rate of the fluorophore knr: rate of non-radiative decay Quantum Yield and Lifetime • Quantum yield – number of emitted photons relative to the number of absorbed photons • Lifetime determines the time available for the fluorophore to interact with or diffuse in its environment 𝞒: emissive rate of the fluorophore knr: rate of non-radiative decay Quantum Yield and Lifetime • Quantum yield – number of emitted photons relative to the number of absorbed photons • Lifetime determines the time available for the fluorophore to interact with or diffuse in its environment 𝞒: emissive rate of the fluorophore knr: rate of non-radiative decay Quantum Yield and Lifetime • Quantum yield – number of emitted photons relative to the number of absorbed photons • Lifetime determines the time available for the fluorophore to interact with or diffuse in its environment 𝞒: emissive rate of the fluorophore knr: rate of non-radiative decay Quantum Yield and Lifetime • Quantum yield – number of emitted photons relative to the number of absorbed photons • Lifetime determines the time available for the fluorophore to interact with or diffuse in its environment 𝞒: emissive rate of the fluorophore knr: rate of non-radiative decay Quantum Yield and Lifetime • Quantum yield – number of emitted photons relative to the number of absorbed photons • Lifetime determines the time available for the fluorophore to interact with or diffuse in its environment 𝞒: emissive rate of the fluorophore knr: rate of non-radiative decay Quantum Yield and Lifetime • Quantum yield – number of emitted photons relative to the number of absorbed photons • Lifetime determines the time available for the fluorophore to interact with or diffuse in its environment 𝞒: emissive rate of the fluorophore knr: rate of non-radiative decay Fluorescence Quenching • Quenching decreases the intensity of fluorescence • Collisional quenching (dynamic quenching) occurs when the excited-state fluorophore is deactivated upon contact with some other molecule in solution, the quencher. Fluorescence Quenching • Quenching decreases the intensity of fluorescence • Collisional quenching (dynamic quenching) occurs when the excited-state fluorophore is deactivated upon contact with some other molecule in solution, the quencher. Fluorescence Quenching • Quenching decreases the intensity of fluorescence • Collisional quenching (dynamic quenching) occurs when the excited-state fluorophore is deactivated upon contact with some other molecule in solution, the quencher. Stern-Volmer equation K: Stern-Volmer quenching constant kq: bimolecular quenching constant 𝜏0: unquenched lifetime [Q]: quencher concentration Fluorescence Quenching • Quenching decreases the intensity of fluorescence • Collisional quenching (dynamic quenching) occurs when the excited-state fluorophore is deactivated upon contact with some other molecule in solution, the quencher. Common quenchers: • O2 • Halogens Stern-Volmer equation K: Stern-Volmer quenching constant kq: bimolecular quenching constant 𝜏0: unquenched lifetime [Q]: quencher concentration • Amines • Electron-deficient molecules Fluorescence Quenching • Quenching decreases the intensity of fluorescence • Static quenching takes place when fluorophores form nonfluorescent complexes with quenchers. This occurs in the ground state and does not rely on diffusion of molecular collisions. Timescale of Molecular Processes in Solution • Quenching can provide information to understand the role of the excited-state lifetime in allowing fluorescence measurements to detect dynamic processes in solution or in macromolecules. Timescale of Molecular Processes in Solution • Quenching can provide information to understand the role of the excited-state lifetime in allowing fluorescence measurements to detect dynamic processes in solution or in macromolecules. • Franck-Condon principle: absorption occurs so fast that there is not time for molecular motion during the absorption process. Timescale of Molecular Processes in Solution • Quenching can provide information to understand the role of the excited-state lifetime in allowing fluorescence measurements to detect dynamic processes in solution or in macromolecules. • Franck-Condon principle: absorption occurs so fast that there is not time for molecular motion during the absorption process. • In contrast to absorption, emission occurs over a longer period of time. Timescale of Molecular Processes in Solution • Quenching can provide information to understand the role of the excited-state lifetime in allowing fluorescence measurements to detect dynamic processes in solution or in macromolecules. • Franck-Condon principle: absorption occurs so fast that there is not time for molecular motion during the absorption process. • In contrast to absorption, emission occurs over a longer period of time. Timescale of Molecular Processes in Solution Fluorescence Anisotropy • Anisotropy is the property of being directionally dependent, as opposed to isotropy, which means homogeneity in all directions. It can be defined as a difference in the physical property of a certain material when measured along different axes. Fluorescence Anisotropy • Anisotropy measurements are based on the principle of photoselective excitation of fluorophores by polarized light. Fluorescence Anisotropy • Anisotropy measurements are based on the principle of photoselective excitation of fluorophores by polarized light. Given the sample is excited with vertically polarized light: I∥: fluorescence intensity of the vertically polarized emission I⊥: fluorescence intensity of the horizontally polarized emission Fluorescence Anisotropy • Anisotropy measurements are based on the principle of photoselective excitation of fluorophores by polarized light. Phenomena that decrease measured anisotropy: • Rotational diffusion Fluorescence Anisotropy • Anisotropy measurements are based on the principle of photoselective excitation of fluorophores by polarized light. Phenomena that decrease measured anisotropy: • Rotational diffusion • Transfer of excitation between fluorophores Fluorescence Anisotropy • Anisotropy measurements are based on the principle of photoselective excitation of fluorophores by polarized light. Phenomena that decrease measured anisotropy: • Rotational diffusion • Transfer of excitation between fluorophores Fluorescence Anisotropy • Anisotropy measurements are based on the principle of photoselective excitation of fluorophores by polarized light. Phenomena that decrease measured anisotropy: • Rotational diffusion • Transfer of excitation between fluorophores Resonance Energy Transfer • Another important process that occurs in the excited state is resonance energy transfer (RET). • RET occurs when the emission spectrum of a fluorophore, called the donor, overlaps with the absorption spectrum of another molecule, called the acceptor. Resonance Energy Transfer Resonance Energy Transfer r: distance between the donor (D) and acceptor (A) 𝜏D: lifetime of the donor in the absence of energy transfer Resonance Energy Transfer r: distance between the donor (D) and acceptor (A) 𝜏D: lifetime of the donor in the absence of energy transfer R0: Förster distance r: distance between the donor (D) and acceptor (A) Steady-State and Time-Resolved Fluorescence • Steady-state measurements are more common; performed with constant illumination and observation. Steady-State and Time-Resolved Fluorescence • Steady-state measurements are more common; performed with constant illumination and observation. Steady-State and Time-Resolved Fluorescence • Steady-state measurements are more common; performed with constant illumination and observation. Steady-State and Time-Resolved Fluorescence • Steady-state measurements are more common; performed with constant illumination and observation. • Steady-state: average of the time-resolved phenomena over the intensity decay of the sample Steady-State and Time-Resolved Fluorescence • Steady-state measurements are more common; performed with constant illumination and observation. • Steady-state: average of the time-resolved phenomena over the intensity decay of the sample I0: intensity at t = 0 r0: anisotropy at t = 0 Steady-State and Time-Resolved Fluorescence • Steady-state measurements are more common; performed with constant illumination and observation. • Steady-state: average of the time-resolved phenomena over the intensity decay of the sample I0: intensity at t = 0 r0: anisotropy at t = 0 Steady-State and Time-Resolved Fluorescence • Steady-state measurements are more common; performed with constant illumination and observation. • Steady-state: average of the time-resolved phenomena over the intensity decay of the sample I0: intensity at t = 0 r0: anisotropy at t = 0 Perrin equation Steady-State and Time-Resolved Fluorescence • Steady-state measurements are more common; performed with constant illumination and observation. • Steady-state: average of the time-resolved phenomena over the intensity decay of the sample I0: intensity at t = 0 r0: anisotropy at t = 0 Perrin equation Steady-State and Time-Resolved Fluorescence • Steady-state measurements are more common; performed with constant illumination and observation. • Steady-state: average of the time-resolved phenomena over the intensity decay of the sample I0: intensity at t = 0 r0: anisotropy at t = 0 Perrin equation Time-Resolved Fluorescence – Value? Time-Resolved Fluorescence – Value? • Molecular information is lost in the time averaging process performed Time-Resolved Fluorescence – Value? • Molecular information is lost in the time averaging process performed Time-Resolved Fluorescence – Value? • Molecular information is lost in the time averaging process performed Time-Resolved Fluorescence – Value? • Intensity decays reveal how acceptors are distributed in space around the donors • They reveal whether quenching is due to diffusion or to complex formation Biochemical Fluorophores • Intrinsic fluorophores and Extrinsic fluorophores Biochemical Fluorophores • Intrinsic fluorophores and Extrinsic fluorophores • Intrinsic fluorophores – those that occur naturally Biochemical Fluorophores • Intrinsic fluorophores and Extrinsic fluorophores • Intrinsic fluorophores – those that occur naturally • Extrinsic fluorophores – those added to a sample that does not display the desired spectral properties Biochemical Fluorophores • Intrinsic fluorescence of proteins Biochemical Fluorophores • Extrinsic fluorescence for membranes Biochemical Fluorophores • Extrinsic fluorescence for DNA Biochemical Fluorophores • Other examples Biochemical Fluorophores • a Biochemical Fluorophores • a Biochemical Fluorophores • Displays high intensity • Stable during continuous illumination • Does not substantially perturb the biomolecule or process being studied Fluorescent Indicators • Fluorophores whose spectral properties are sensitive to a substance of interest Molecular Information from Fluorescence • EMISSION SPECTRA AND THE STOKES SHIFT Molecular Information from Fluorescence • EMISSION SPECTRA AND THE STOKES SHIFT Molecular Information from Fluorescence • EMISSION SPECTRA AND THE STOKES SHIFT Molecular Information from Fluorescence • EMISSION SPECTRA AND THE STOKES SHIFT Molecular Information from Fluorescence • QUENCHING OF FLUORESCENCE Molecular Information from Fluorescence • QUENCHING OF FLUORESCENCE Molecular Information from Fluorescence • QUENCHING OF FLUORESCENCE Molecular Information from Fluorescence • FLUORESCENCE POLARIZATION OR ANISOTROPY Molecular Information from Fluorescence • FLUORESCENCE POLARIZATION OR ANISOTROPY Molecular Information from Fluorescence • FLUORESCENCE POLARIZATION OR ANISOTROPY • Larger molecules rotate more slowly; smaller molecules rotate faster Molecular Information from Fluorescence • FLUORESCENCE POLARIZATION OR ANISOTROPY Molecular Information from Fluorescence • FLUORESCENCE POLARIZATION OR ANISOTROPY Molecular Information from Fluorescence • FLUORESCENCE POLARIZATION OR ANISOTROPY Molecular Information from Fluorescence • RESONANCE ENERGY TRANSFER (RET) Molecular Information from Fluorescence • RESONANCE ENERGY TRANSFER (RET) Molecular Information from Fluorescence • RESONANCE ENERGY TRANSFER (RET) Basic Biochemical Phenomena • Emission spectra of proteins Basic Biochemical Phenomena • Association reactions and anisotropy Basic Biochemical Phenomena • RET and DNA hybridization Basic Biochemical Phenomena • RET and gene expression New Fluorescence Technologies Multiphoton Excitation Multiphoton Excitation Multiphoton Excitation • a Fluorescence Correlation Spectroscopy • FCS based on temporal fluctuations occurring in a small observed volume Fluorescence Correlation Spectroscopy • FCS based on temporal fluctuations occurring in a small observed volume Fluorescence Correlation Spectroscopy • FCS based on temporal fluctuations occurring in a small observed volume Fluorescence Correlation Spectroscopy • FCS based on temporal fluctuations occurring in a small observed volume Fluorescence Correlation Spectroscopy • FCS based on temporal fluctuations occurring in a small observed volume Single Molecule Detection • SMD gives very high obtainable sensitivity Single Molecule Detection • SMD gives very high obtainable sensitivity Single Molecule Detection • SMD gives very high obtainable sensitivity Single Molecule Detection • SMD gives very high obtainable sensitivity Single Molecule Detection • SMD gives very high obtainable sensitivity Fluorescence Spectroscopy