Fluorescence Spectroscopy - BIOC3570 Notes PDF
Document Details
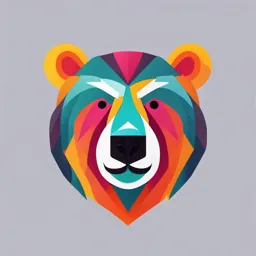
Uploaded by YouthfulGothicArt
Tags
Related
- Lecture 3 - Tan Bioanalytical Techniques PDF
- Molecular Analytics Premaster PDF - Introduction to UV-vis & Fluorescence
- Molecular Analytics Premaster L1 Introduction PDF
- Physical Chemistry II CHEM 3340 Molecular Spectroscopy: Electronic Transitions PDF
- Chapter 15 Molecular Luminescence Spectroscopy PDF
- Fluorescence Spectroscopy PDF
Summary
These are lecture notes on fluorescence spectroscopy, covering the essence of various spectroscopic concepts, including fluorescence, phosphorescence, chemiluminescence, and bioluminescence. It also describes fluorescence properties like the process of fluorescence, the importance of fluorescent molecules, types of fluorophores, quantification of fluorescence and examples of fluorescence in applications. Information in the text is presented using diagrams and other visualizations to help explain the concept.
Full Transcript
Fluorescence spectroscopy The essence of “-escences” Absorbance: Photon in ® excited state energy is dissipated as translational/rotational energy (heat) Fluorescence: Photon in ® excited state energy emitted as a photon (within a few nanoseconds) Phosphorescence: Photon in ® exc...
Fluorescence spectroscopy The essence of “-escences” Absorbance: Photon in ® excited state energy is dissipated as translational/rotational energy (heat) Fluorescence: Photon in ® excited state energy emitted as a photon (within a few nanoseconds) Phosphorescence: Photon in ® excited state energy emitted as a photon (within a seconds to minutes) Chemiluminescence: Chemical reaction ® excited state energy emitted as a photon (e.g. luminol + H2O2) Bioluminescence: chemiluminescence, but produced by a living organism 493-507 Fluorescence Kimber 2020 In fluorescence, we are measuring the intensity of light that certain molecules – termed fluorophores - emit This light is at a longer wavelength than the light absorbed, and is emitted in all directions All fluorophores absorb light, but only some light absorbing molecules emit significant fluorescence Fluorescence Spectroscopy: The fluorescence process Absorbing a photon results in an excited electronic state Intermolecular collisions (in solution) cause rapid (picosecond) relaxation to the lowest vibration rotation state of S Fluorescence (photon emission) occurs from the ground vibrational state of S to any vibrational state of G. The emission spectrum is shifted to longer l than the excitation spectrum. This is known as Stokes' shift. Kimber 2020 479-481 Fluorescent molecules are typically rigid Kimber 2020 Molecules with considerable internal flexibility can disperse energy by transferring it into rotational modes This tends to result in the highest energy rotational modes of the ground electronic state overlap with the lowest excited electronic state The molecule can therefore reach the ground state without emitting a UV/visible photon Rigid, generally polycyclic aromatics have few rotational state Relaxation requires emitting a high energy photon – or to fluoresce Absorption vs emission spectra Anthracene absorption The different excited states spectrum are split by the same relaxation modes This means that the fluorescent spectrum tends to show a similar set of emission peaks to the absorption spectrum spectrum, only mirrored Examples of fluorophores used in biochemistry Fig. 13.18 Ethidium Fluorescein ANS (8-anilinospthalene-1-sulfonic acid) Acridine orange Fluorescence intensity is a function of both excitation and emission wavelengths 3D plot of fluorescence spectrum; peak at λexc = 405 nm and λem = 517 nm Elsinghorst et al., Org. Biomol. Chem. 7: 3940-3946, 2009. Fluorescence spectrometer sample cell incident transmitted emitted excitation lamp monochromator emission monochromator Fluorescence is measured as photons emitted against a “black” background (no fluorescence = no light). detector This is fundamentally much more sensitive than measuring light differences (as in absorbance). 481 - 482 Light source: Xenon arc lamp Extreme intensity gives increased sensitivity of detection, and is desirable for fluorescence measurements Xenon arc lamps give intense continuous light output 250-700 nm These have a fused quartz envelope (UV transparent), tungsten electrodes and are filled with Xenon (noble gas) at high pressure (10 atmospheres) A specially designed housing protects the user from explosion danger and intense brightness An operating xenon arc lamp should never be observed directly Fluorescence spectroscopy: quantitation Intensity of fluorescence depends on lamp intensity There is no direct way to compare output to input (as in absorbance/transmission) Fluorescence is measured in arbitrary (instrument-specific) units. “Arbitrary units” or “a.u.” (e.g., text, Fig. 13.17) (do not confuse with AU = absorbance units!) (some papers prefer “relative fluorescent units” or RFU) To quantify concentrations, prepare and measure a standard curve on the same instrument, under identical conditions. 486 - 487 Quantum yield and quenching Quantum yield (Q) is the number of fluorescence photons emitted per absorption event. i.e. Q = F / (I0 - I) Q £ 1 (at most one photon emitted per photon absorbed) biphenyl Q ~ 0.2 fluorene Q ~ 1 Molecular environment affects Q, as interactions with solvent quench (reduce) fluorescence Q can therefore serve as a probe of the local environment of the fluorescent species. Fluorescence spectroscopy: quantitation Q = F / (I0 - I) (the definition of Q) I = 10"#$% (Beer-Lambert law, since A = log [I0/I]) I! F = Q (I0 - I010-εcl) = Q I0 (1 - e-2.3εcl) For small values of x, 1 – ex is approximately x So F ≃ 2.3 Q I0 ε c l At low concentrations, fluorescence intensity is proportional to: 1) incident light intensity and path length and 2) the concentration, quantum yield, and molar extinction coefficient of the fluorophore. Practice question Which of the following does not affect the intensity of observed fluorescence? A. The wavelength of the incident light B. The molar extinction coefficient of the fluorophore C. The intensity of the incident light D. The concentration of the fluorophore E. Actually, these all affect fluorescent intensity Practice question - solution Which of the following does not affect the intensity of observed fluorescence? A. The wavelength of the incident light B. The molar extinction coefficient of the fluorophore C. The intensity of the incident light D. The concentration of the fluorophore E. Actually, these all affect fluorescent intensity Applications of fluorescence spectroscopy in biochemical analysis 482 - 489 enzyme assays: peptidase/protease degradation assays detection of biomolecules by binding of extrinsic fluorophores - covalently, e.g. to protein cysteine residues; DNA primers; “dye-deoxy” terminators for DNA sequencing -non-covalently, e.g. bound to enzyme active sites detection of intrinsic fluorescence of biomolecules (e.g. Trp) Applications of fluorescence spectroscopy in biochemical analysis Peptidase assays using 7-amino-4-methylcoumarin (AMC) ClpP PKM-AMC PKM + AMC o SaClpP Active o o o o o Higher fluorescence o intensity because of o higher quantum yield o o o o Dead ClpP2 o o o o o o o o o o o o o ClpP1 Time (min) Sigma 17 Vahidi, S., Ripstein, Z. A., Juravsky, J. B., Rennella, E., Goldberg, A. L., Mittermaier, A. K., Rubinstein, J. L., & Kay, L. E. (2020). PNAS, 117(11), 5895–5906 Applications of fluorescence spectroscopy in biochemical analysis Characterizing rapid mixing devices 18 Vahidi, S., Stocks, B. B., Liaghati-Mobarhan, Y., & Konermann, L. (2013). Analytical Chemistry, 85, 8618–8625. Intrinsic fluorescence of polypeptides emission max ~350 nm intensity (arb. units) Tryptophan: fluorescence spectrum l (nm) http://www.mdtmag.com/article/2015/11/uvc-leds-redefining-point-care-diagnostics Tryptophan is the only amino acid that has significant fluorescence Trp is commonly used as a “reporter” of protein conformation Tryptophan emission spectrum is sensitive to its environment 1 = native 2 = denatured exc. = 290 nm. Bougie and Bisaillon, J. Biol. Chem. 278: 52471-52478, 2003. Fluorescence emission spectrum of NS5B protein Emission max. (339 nm) is blue-shifted relative to that of free Trp (350 nm) This shift is due to shielding of the Trp residues from the aqueous phase Denaturation (8 M urea) results in a red shift of λmax toward 350 nm and a decrease in total fluorescence Trp fluorescence can therefore be used to monitor protein unfolding Protein denaturation can be monitored via the Trp emission peak LDH thermal denaturation profiles were monitored via trp fluorescence (exc. = 295 nm, em.= 347 nm). (LDH has 6 Trp) longjaw mudsucker Fluorescence intensity was monitored at 347 nm as the cuvette temperature was stepped from 20-85 °C. G. mirabilis LDH M G. seta LDH F Melting temperature is measured where the fluorescence intensity reaches half maximum Fields et al., Temperature adaptation in Gillichthys (Teleost: Gobiidae) A4-lactate dehydrogenases, J. Exp. Biol. 205: 1293-1303, 2002. Trp fluorescence can also be used to monitor structural changes redox sensitive oxidizing conditions disulfide bond reducing conditions Trp13 Pena et al, PNAS 2009 This carbonic anhydrase from cyanobacteria requires a disulfide bond to form to be active It is inactive when first synthesized in the cytosol(reducing), and only switches on once it reaches an oxidizing environment Trp13 becomes locally unfolded when reduced, and is therefore quenched Trp fluorescence allows local structural changes to be monitored CD monitors protein structural transitions 23 Vahidi, S., Stocks, B. B., Liaghati-Mobarhan, Y., & Konermann, L. (2012). Analytical Chemistry, 84, 9124–9130. With Trp in the binding site, intrinsic fluorescence can report on ligand binding Trp181 human galectin-3 (pdb 4jck) Scientific Reports volume 9, Article number: 11851 (2019) Surface exposed Trp residues tend to be quenched by the solvent If Trp is exposed in a binding site, ligand binding can be observed as increasing Trp fluorescence as the ligand protects the Trp Here, the binding affinity of lactose to Galectin-3 is determined by observing increase in fluorescence at 346 nm Labelling proteins with extrinsic fluorophores Maleimide groups will react note: maleimide will react with efficiently and irreversibly with any –SH group, not just Cys thiols to form new covalent bonds fluorophore Maleimide can be used to selectively cysteines on proteins Cys-S Cys-SH + The cysteine needs to be reduced, and surfaced exposed to react maleimide Suitable cysteines may be introduced at specific positions by site directed mutagenesis for labelling Alternatively, lysine can be modified by using isothiocyanate-linked fluorophores e.g. fluorescein- lysozyme covalently 5-maleimide labelled at Lys1 by Note: other types of labels can also isothiocyanate- be attached; e.g. isotope mass labels fluoroescein for mass spectrometry Forster resonance energy transfer (FRET) Kimber 2020 FRET can occur when one fluorophore (the donor) has an emission spectrum that overlaps another molecule’s absorption spectrum (acceptor) Energy is transferred directly from the donor to the acceptor without emitting a photon (this is the “resonance”) The effect is monitored as a reduction in donor fluorescent intensity This process – Forster resonant energy transfer (FRET) – can only occur over short distances (< 10 nm) and falls of rapidly with distance (~r6) FRET reports on the closeness of donor and acceptor FRET can be used to: monitor binding of chromophores/fluorophores monitor protein complex formation to measure distances on a molecular scale to measure motions on a molecular scale Trp is a suitable donor for many common acceptors FRET can also be used to monitor protein interactions within a living cell (using GFP labelled protein) FRET example Jo et. Al. J Enzyme Inhib Med Chem. 2020. 35(1):145-151 The authors used FRET to monitor the binding of flavonoid inhibitors to SARS-CoV 3CL protease Intrinsic Trp fluorescence was excited at 290 nm, and fluorescence was monitored at 340 nm The reduction in Trp fluorescence as a function of inhibitor concentration yielded binding constants for all 3 inhibitors Fluorescent microscopy – fluorescent dyes A number of molecules have been discovered that specifically bind specific cellular macromolecules These include synthetic organic molecules with intrinsic fluorescence, or repurposed natural products with added labels These stains can be used to label a specific molecule in the cell In addition, antibodies can be modified with fluorophores, and used to label almost any protein in cells However, this requires that the cells be permeabilized by making holes in membranes (e.g. with detergents), so cells are dead Fluorophores that become fluorescent upon binding specific metal ions (e.g. Ca2+) are also available Stain e.g. #1: DAPI DAPI Absorbance max: 358 nm DAPI binds specifically in the minor Emission max: 461 nm groove of dsDNA DAPI was originally developed as a (failed) trypanosomiasis drug It was noted to bind dsDNA with high affinity Once bound to DNA it fluoresces blue (water quenches free DAPI fluorescence) It is hydrophobic enough to get into cells without permeabilizing DAPI added to cells will stain all DNA (generally the nuclei) blue Stain e.g. #2: Phalloidin actin filament chemically linked fluorophore of choice (any colour) death cap mushroom phalloidin phalloidin (pdb 6T1Y) Phalloidin is a cyclic peptide naturally made by highly toxic death cap mushrooms It binds tightly to actin filaments (but not depolymerized actin) This prevents actin de-polymerization, which will kill the organism Synthetic analogs readily enter cells, and can be bought pre-labelled with a wide variety of fluorophores Phalloidin stains the actin filaments of the cell; colour depends on linked fluorophore Stains allow facile identification of key cellular structures in fluorescence microscopy Rat glioma cells stained with rhodamine phalloidin (red) for actin and DAPI (blue) for nucleus. Imaged with a confocal fluorescence microscope. http://spine.rutgers.edu/if/ Fluorescent microscopy with fluorescent proteins: Green fluorescent protein (GFP) Pacific jellyfish, Aequoria victoria GFP localization Pacific jellyfish, like many related organisms, are bioluminescent Bluish luminescent light is produced by a molecule known as aequorin This light is emitted via a coupled molecule known as green fluorescent protein This allows the jellyfish to glow green, despite being only capable of making blue light. Green fluorescent protein (GFP) MW: 26.9 kDa extinction peak: 395 nm emission peak : 509 nm Quantum yield: 0.8. purified recombinant GFP GFP is a small protein that is naturally fluorescent GFP has a blue excitation peak, and green emission with excellent quantum yield. It can readily be expressed recombinantly in most organisms top view 11 stranded b-barrel 12th strand inserted in the middle of the barrel three residues from the middle The structure of GFP (pdb 1EMA) strand react to form the fluorophore GFP fluorophore OH cyclization OH dehydration OH NH Ser65 NH NH H NH HOCH2 HOCH2 N N Tyr66 HOCH2 O HO N HN O N O O CH2 CH2 CH2 Gly67 O OH oxidation NH NH N N HOCH2 HOCH2 N N OH O CH2 CH2 Cody et al., Biochemistry 32: 1212-1218, 1993; Barondeau et al., JACS 128: 3166-3168, 2006. Engineered fluorescent protein variants allow labelling across the spectrum Tsien 2008, Nobel address mCherry variants Amino acid substitutions in or near (mushroom coral; 2000) the fluorophore shift the spectrum GFP labelling proteins gfp multi-cloning study protein linker gfp site A common microscopy strategy is to build a construct where the protein of interest is fused to a desired GFP colour variant Expression within a cell will reveal allow real time visualization of the protein’s location GFP labelling does not disrupt cells, so GFP-fusion proteins can be used to follow the behaviours within living cells Since you can do this for almost any protein, this is a key enabling technology for modern cell biology Practice question A major advantage of using GFP (or another fluorescent protein) fusion instead of fluorescently labelled antibodies to visualize proteins in cells is: A. More colours are available B. The fluorescent signal is brighter C. The fluorescence will then show the location of the protein within the cell D. Cells can be imaged alive E. No recombinant work is required Practice question - answer A major advantage of using GFP (or another fluorescent protein) fusion instead of fluorescently labelled antibodies to visualize proteins in cells is: A. More colours are available B. The fluorescent signal is brighter C. The fluorescence will then show the location of the protein within the cell D. Cells can be imaged alive E. No recombinant work is required Recall cells need to be permeabilized to get antibodies into the cell; this kills them Fluorescence spectroscopy: Summary Principle: Molecules absorb photons, exciting electrons in molecular orbitals; excited states decay by radiative processes, emitting photons of lower energy. Applications: Quantitative analysis of molecules with useful fluorophores. These are typically structurally rigid aromatic molecules. Rare in nature, but many synthetic fluorescent molecules are available. Strengths: High sensitivity, because photons are detected relative to a dark background. Can be used in high-throughput mode (microplate readers). Direct measurement of analyte concentration, if a standard is available. Few interferences, since few biomolecules are fluorescent. Weaknesses: Applicable only to molecules with good fluorophores. Sensitive to environmental quenching – although this can be turned to an advantage, using fluorescence as a structural probe (e.g., protein denaturation).