Electrolytes Part 1 PDF
Document Details
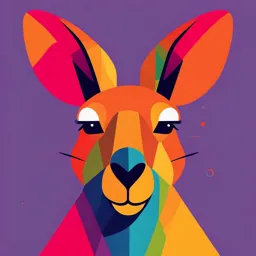
Uploaded by TruthfulMusicalSaw
Conestoga College
null
Tags
Summary
This document covers the basics of electrolytes and water balance, including their roles in maintaining proper body function. It describes the regulation of water balance and electrolytes, types of electrolytes, and their functions. The document also discusses the composition of body fluids, electrolyte distribution, and clinical significance of several electrolytes.
Full Transcript
CHEM2010 Electrolytes - PART 1 CHAPTER 16 - TEXTBOOK (8TH ED.) CHAPTER 11 - TEXTBOOK (9TH ED.) Water Balance, Electrolytes, + Osmolality Water Balance Water intake should equal water output If intake exceeds output → Edema occurs (fluid in the tissues) If outp...
CHEM2010 Electrolytes - PART 1 CHAPTER 16 - TEXTBOOK (8TH ED.) CHAPTER 11 - TEXTBOOK (9TH ED.) Water Balance, Electrolytes, + Osmolality Water Balance Water intake should equal water output If intake exceeds output → Edema occurs (fluid in the tissues) If output exceeds input → Dehydration occurs ex. Seen in severe vomiting or diarrhea Daily Water Balance Kidneys are the ultimate regulators of water in the body and can adjust levels as needed (!) If H2O intake is high - kidneys excrete more H2O → Dilute, pale urine If H2O intake is low - kidneys conserve more H2O → Concentrated, dark yellow urine Kidneys Ultimate regulators Kidneys are flexible and will adjust volume and concentration of urine (!) High H2O intake → Kidneys excrete more H2O → Dilute urine Low H2O intake → Kidneys conserve H2O → Concentrated urine Two important hormones: Antidiuretic Hormone (ADH) [AKA Arginine Vasopressin Hormone (AVP)] Increases water reabsorption Secretion is stimulated by the hypothalamus Aldosterone Increases sodium reabsorption Produced by the adrenal cortex ↑ aldosterone = More Na+ (and Cl-) reabsorbed in exchange for H+ (or K+) into the urine. Water Body Water Compartments The water content of the human body is distributed into 3 main compartments: Intracellular Fluid Inside cells 40-50% of body weight Extracellular Fluid (outside cells) 2 main subdivisions: Intravascular ECF (plasma) 5% of body weight Interstitial Cell Fluid (lymph & tissue) 15% of body weight Transcellular (not significant) Special compartments with special fluids (e.g. CSF, synovial, gastric) Introduction to Electrolytes Electrolytes: Ions capable of carrying an electric charge Two types 1. Anions have negative charge and move toward anode (+). 2. Cations have positive charge and move toward cathode (-). Electrolytes are an essential component in many processes: Volume and osmotic regulation (Na+, Cl-, K+) Myocardial rhythm and contractility (K+, Mg2+, Ca2+) Cofactors in enzyme activation (Mg2+, Ca2+, Zn2+) Blood coagulation (Ca2+, Mg2+) Acid-base balance (HCO3-, K+, Cl-) Neuromuscular excitability (K+, Ca2+, Mg2+) Composition of Body Fluids ECF: Main cation = Na+ Main anion = Cl- ICF: Main cation = K+ Main anion = HPO42- Electrolyte Distribution [Na+] in ECF is 10-15 times that of ICF; (!) Concentration difference is maintained by Na+-K+ ATPase pump. Na+ is the main cation of ECF K+ is the main cation of ICF [K+] is 23 times higher in ICF than in ECF In hemolyzed samples, K+ will be released into the ECF, causing falsely high results. Always check the samples of high K+ results before reporting! Electrolyte Distribution Chief anion of ECF is Cl- Chief anion of ICF is HPO42- Increase in an Anion If you have an increase in one anion, you must also have: A decrease in another anion OR An increase in one or more cations OR Both Why? —> Total Anions = Total Cations Osmolality Osmolality has replaced specific gravity as the test to assess renal concentration. Specific gravity includes number and size of molecules (i.e. weight) Osmolality is a measure of concentration based only on the # of small molecules present in solution (not on their weight or size) ex. 100 mmol Na+ contributes 100 mmol to the osmolality 100 mmol NaCl contributes 200 mmol to the osmolality (2 particles produced upon dissociation) Particles will change the colligative properties of a solution. ex. An increase in particles will decrease the freezing point Changes in Osmolality The hypothalamus will respond to changes in osmolality. As osmolality increases, body fluids become more concentrated. The body controls this increase by stimulating the hypothalamus to: Feel thirsty Secrete Antidiuretic Horomone (ADH) In response to the thirst sensation: More fluid is consumed The H2O content of the ECF increases Elevated solute levels (Na+) are diluted Osmolality of plasma decreases Reference Ranges for Osmolality Serum 275 - 295 mOsm/kg Urine (24hr) 300 - 900 mOsm/kg Urine (random) 50 - 1200 mOsm/kg Osmolal Gap 5 - 10 mOsm/kg Critical serum values 325 mOsm/kg Changes in Osmolality Na+ (and its associated anions) account for ~90% of osmotic activity in plasma. The concentration of Na+ in plasma is affected by: The regulation of osmolality The regulation of blood volume Osmoreceptors in the hypothalamus respond to small changes in osmolality. An increase in osmolality causes an increase in ADH concentration. A decrease in osmolality shuts off ADH production. ADH increases H2O reabsorption in the kidneys Water Excess Renal water excretion is important in controlling water excess Excess intake of H2O (polydipsia) will lower plasma osmolality. AVP and thirst will be suppressed H2O will not be reabsorbed Large volume of dilute urine excreted (10 - 20 L) Hypo-osmolality and hypo-natremia usually only occur if there is an impairment with the renal excretion of water. Water Deficit Thirst is important in preventing water deficit. Deficit of H2O will increase plasma osmolality. AVP and thirst will be activated AVP will cause H2O to be reabsorbed Thirst is the major defense against hyperosmolality and hypernatremia Hyper-osmolality and hyper-natremia is a concern for: Infants Unconscious patients Older patients Those with diminished mental status For these patients, dehydration is a concern. Diabetes Insipidus No ADH production or no ability to respond to circulating ADH Excessive thirst Increased urine output (up to 10 L/day) Water intake = water output Plasma osmolality remains normal Regulation of Blood Volume Response to decreased blood volume or blood pressure (hypovolemia): Renin is secreted near renal glomeruli Renin converts Angiotensinogen → Angiotensin I Angiotensin-Converting Enzyme (ACE) converts Angiotensin I into Angiotensin II Angiotensin II causes vasoconstriction Blood pressure is increased Aldosterone is excreted which increases retention of Na+ and the H2O that accompanies it. Electrolytes + Ion-Selective Electrodes Ion-Selective Electrode Potentiometry - Key Words Activity - directly related to the concentration of an ion Oxidation - loss of electrons at the anode Reduction - gain of electrons at the cathode Anode - positive electrode; where oxidation takes place Cathode - negative electrode; where reduction takes place Electrochemical cell - combination of 2 half cells (oxidation and reduction), connected by a salt bridge Electrolyte Functions Affect almost all metabolic processes Maintain osmotic pressure and water distribution (Na+) Maintain pH (HCO3-/H+) Regulate the function of heart and other muscles (K+, Ca++) Involved in oxidation-reduction reactions (H+) Act as cofactors for enzymes One of the most important lab analyses Usually includes sodium, chloride, potassium and bicarbonate As well as the anion gap ISE Potentiometry The first ion-selective electrode (ISE) instrument was developed in the 1930’s - Our friend, the pH meter :) Potentiometry - Measures voltage (mV) generated by an electrochemical cell at zero current Electrochemical cell - 2 half cells or electrodes connected by a salt bridge Indicator (ISE) Electrode - detects the ion of interest Reference Electrode - provides constant potential Note: ISE’s used in instruments have all the components (indicator electrode, reference electrode and salt bridge) in one unit. Indicator (ISE) Electrode Measuring electrode constructed to detect a specific ion of interest Generates electrical potential when placed in a solution containing the ion to be measured. The potential generated is proportional to the concentration of the ion (activity) Reference Electrode Surrounded by a solution of constant composition At constant pH; generates constant potential; compensates for changes in temp., electrical noise, age of electrodes, etc. Common reference electrodes: Silver/ silver chloride (Ag/AgCl) Calomel (mercury/ mercury chloride) Hg/HgCl Difference between potential from the reference electrode and the indicator (ISE) electrode = principle of potentiometry i.e. The potential generated by the indicator electrode is compared to the constant potential of the reference electrode and the potential difference is measured. Most electrodes have both half cells in one unit Nernst Equation The relationship between the potential generated at the electrode to the concentration of an ion in solution. E = measured potential Eo = standard electrode potential (constant) R = molar gas constant T = absolute temperature n = ionic valence F = Faraday’s constant a = activity (concentration) of the ion Reduces to: E ~ log a i.e. Measuring potential (mV) ~ activity (concentration) of the ion Notes Regarding Ion-Selective Electrodes ISEs are selective but not specific (aka. Other interfering ions are also measured to a slight degree) Each ion has a selectivity factor to determine the amount of interference it will cause Electrodes are designed to have the lowest possible selectivity factors for interfering ions. Classes of Electrodes Solid material (eg. Glass) Specific types of glass used (eg. H+, Na+) Can be modified using a gas-permeable membrane (eg. CO2) Liquid (Ion-exchange) Binds with ion being measured (eg. K⁺) Solid state Insoluble inorganic salts in a membrane; potential due to the ion exchange process. (eg. Cl⁻) Enzyme Enzyme bound to surface; reacts with a non-ion to produce ionic species which can then be measured (urea/urease = NH₄+) Sodium (Na+) Sodium (Na+) Major cation of ECF (90%) Essential in maintaining water distribution and osmotic pressure Largely determines osmolality of plasma. Maintained by dietary intake of 130-260 mmol NaCl: Body uses 1-2 mmol/day, the rest is excreted Na+ and K+ move between the ICF and ECF by the Na+/K+-ATPase ion pump. 3 Na+ ions move out of the cell in exchange for 2 K+ ions as ATP is converted to ADP. Water follows Na+ out of the cell which prevents osmotic rupture of the cell. Regulation of Sodium (Na+) Regulated by three processes: 1. Intake of H2O in response to thirst Stimulated/suppressed by plasma osmolality 2. Excretion of H2O In response to Anti-Diuretic Hormone (ADH) release due to changes in blood volume or osmolality 3. Excretion of Na+ In response to blood volume status Involves aldosterone, angiotensin II, and atrial natriuretic peptide (ANP) Specimen Testing - Sodium Serum (Gold SST or red-top tube) Heparinized Plasma (Lithium heparin, ammonium heparin, lithium oxalate) Urine (Random or 24 hour collection) Not significantly affected by hemolysis Refrigerate or freeze for delayed analysis Methods of Analysis - Sodium Chemical methods - outdated Flame emission spectrophotometry Atomic absorption spectrophotometry (AAS) Ion-selective electrodes (ISEs) Most common method in clinical labs Sodium Electrode A glass ion-exchange membrane is used for Na+ measurement. Na⁺ interacts with the tip (measuring electrode) producing a potential that is dependant on the activity/concentration of Na+. The reference electrode maintains a constant potential. The difference in potential between the reference and measuring electrodes = the activity of Na⁺. Measurement Modes for ISEs There are two types of ISE Measurement: Direct Measurement Undiluted sample interacts with the ISE membranes Elevated lipids/proteins do not interfere More accurate Indirect Measurement A diluted sample is used for measurement. Results are the same as the direct method EXCEPT when samples have increased lipids or proteins. Electrolyte exclusion effect Excess lipids or proteins displace plasma water Less aqueous phase (containing the electrolytes) gets added to the diluent This causes pseudohyponatremia – falsely decreased Na+ concentration Na+ is found in the highest concentration in serum/plasma and therefore is the most affected. Sodium Electrode - Sources of Error Protein build up on electrode membranes through continuous use. Causes poor selectivity and poor reducibility of results Solution: Routine maintenance to remove protein buildup :) Sodium - Clinical Significance Hyponatremia - A Na+ level 160 mmol/L are critical Causes of Hypernatremia Increased Intake or Retention Excess H2O Loss Decreased H2O Intake Hyperaldosteronism Diabetes insipidus Older persons or infants ↑ aldosterone; ↑ Na+ reabsorption Loss of water through urine Excess ingestion of salt Profuse Sweating Patients with mental impairment Administration of hypertonic Fever, burns, diarrhea, exposure solutions to heat Sodium bicarbonate Dialysis solutions Sodium Reference Ranges Critical Results: < 120 or >160 mmol/L Potassium (K+) Potassium (K+) Major cation of the intracellular fluid (ICF). Average daily dietary requirements: 50-150 mmol/day. Small amount used, most excreted by kidneys (not stored well) Potassium concentration regulates neuromuscular excitability, heart contraction Too low or too high of a concentration can lead to cardiac arrest (!) Regulation of Potassium (K+) Nearly all K+ is reabsorbed in the proximal tubules of the kidney Additional K+ is secreted into urine in exchange for Na+ Any excess K+ consumed in the diet is excreted in the urine Can accumulate to toxic levels in renal failure Factors that influence distribution of K+ K+ loss due to Na+,K+ ATPase pump inhibition Hypoxia, hypomagnesemia, digoxin overdose Insulin promotes acute entry of K+ into skeletal muscle and liver by ↑ Na+,K+ ATPase activity. Catecholamines like epinephrine (β-stimulator) promote entry of K + into cells. Propanolol (β-blocker) impairs entry of K+ into cells. Factors that influence distribution of K+ Exercise K+ released from muscle cells during exercise Fist pumping during venipuncture can cause falsely high K+ levels Cellular Breakdown Damage to RBCs releases K+ into the ECF Specimen Collection - Potassium Serum (Gold SST or red-top tube) Plasma (Heparin preferred) Don’t use EDTA (as K2EDTA contains potassium!) Will get an erroneously high potassium result Urine (random or 24 hour collection) Minimize hemolysis (!) Separate plasma / serum from cells ASAP or may give a false (spurious) ↑ K+ result as RBC breakdown occurs and/or intracellular-extracellular fluid exchange occurs with the RBCs Methods of Measurement Flame Photometry Older method Ion-Selective Electrodes (ISEs) Most routinely used Potassium Electrode Ion-exchange electrode Solid plastic membrane containing valinomycin An antibiotic that will selectively bind with K+. KCl is the inner electrolyte solution. Can be direct or indirect mode The difference between the two methods is only slight compared to Na+ Potassium - Clinical Significance Remember: It is important to maintain a normal serum K+ concentration. A ↓’d or ↑’d concentration will affect muscle irritability = cardiac arrest Potassium Reference Ranges Critical Results: < 2.0 or >6.0 mmol/L Remember: Critical K+ levels can cause cardiac arrhythmias and cardiac arrest (!) Chloride (Cl-) Chloride The major anion in ECF (extracellular fluid) Major contributor to osmolality (with Na+) Involved in maintaining proper hydration, blood volume and electric neutrality Regulation of Chloride Cl- usually shifts due to changes in Na+ or HCO3- Cl- in the diet: Absorbed by intestinal tract Then filtered by glomerulus Then passively reabsorbed (with Na+) by proximal tubules Excess Cl- is excreted in urine and sweat. Excessive sweating stimulates aldosterone to act on the sweat glands to conserve Na+ and Cl-. How Chloride Maintains Electroneutrality 1. Na+ is reabsorbed along with Cl- in proximal tubules (kidneys) Na+ reabsorption is limited by amount of Cl- available 2. Chloride Shift **See next slide** CO2 from cellular metabolism in tissues moves into the plasma and RBCs In RBCs, CO2 form carbonic acid which splits into H+ and HCO3- Deoxyhemoglobin (HHb) buffers H+ HCO3- diffuses into plasma Cl- diffuses into RBC and electric balance is maintained Chloride Shift (p.280) (AKA Hamburger Phenomenon) Tissue Cell Plasma RBC CO2 HCO3- CO2 Metabolism Cl- Note: In the lungs, the reverse will occur. HCO3- will enter the cell, Cl- will leave the cell and CO2 will be expelled Chloride Specimen Collection Serum/plasma (separate from cells) Best anticoagulant – lithium heparin Urine (24hr is best) CSF (↓Cl in bacterial meningitis) Sweat (Screening test for Cystic Fibrosis) > 60 mmol/L is positive for Cystic Fibrosis Chloride Methods of Analysis Amperometric-coulometric titration Mercurimetric titration Colorimetry Ion-selective electrodes (ISEs) Chloride Methods of Analysis Amperometric-coulometric titration Silver ions are generated coulometrically from a silver electrode and combine with chloride ions in the patient sample. Ag+ + Cl- → AgCl When all of the Cl- in the sample has bound to Ag+, the free Ag+ indicates the endpoint. The coulometric generator and timer shut off and the amount of time elapsed is used to calculate the Cl- in the sample. An example of this is the Cotlove Chloridometer. Chloride Methods of Analysis Ion-selective electrodes (ISEs) Most common method Uses an ion-exchange membrane to selectively bind Cl- via a redox reaction Used in clinical chemistry analyzers Chloride - Clinical Significance Cl- passively follows Na+. Therefore, Cl- disorders often have the same causes as Na+ disorders, with a few exceptions. Causes of Hypochloremia Causes of Hyperchloremia Increased Cl- Loss Increased Intake Prolonged vomiting Diabetic ketoacidosis - ↑ organic acid ions/ ↓ Cl- to keep neutrality Aldosterone deficiency - ↓ aldosterone, ↓ Na+, ↓ Cl- Pyelonephritis (loss of salts) High Serum HCO - Excess Loss of HCO3- 3 Compensated respiratory acidosis Renal tubular acidosis (RTA) Metabolic alkalosis Metabolic acidosis Through GI tract Chloride Reference Ranges Bicarbonate (HCO3 ) - Bicarbonate (HCO3-) or Total CO2 (TCO2) Bicarbonate is 2nd most abundant anion in ECF Total CO2 is comprised of: Dissolved CO2 Carbonic acid (H2CO3) Bicarbonate Bicarbonate accounts for 90% of total CO2. Therefore, total CO2 is indicative of HCO3- level (!) Bicarbonate (HCO3-) or Total CO2 (TCO2) HCO3- is a major component of buffer systems in blood. NaHCO3-/H2CO3 (sodium bicarbonate/carbonic acid) Participates in “Chloride Shift” in the tissues and lungs This maintains electroneutrality (pluses = minuses on either side of the cell membrane) If HCO3- → into cell And vice versa Then out ← Cl - Regulation of Bicarbonate (HCO3-) 85% of HCO3- is reabsorbed by proximal tubules in kidneys; 15% by distal tubules. Tubules are only slightly permeable to bicarb, so usually reabsorbed as CO2. HCO3- combines with H+ to form H2CO3 H2CO3 dissociates into H2O and CO2 CO2 diffuses back into ECF Excess HCO3- flows into the urine Regulation of Bicarbonate (HCO3-) In alkalosis: Kidneys increase excretion of HCO3- (basic / alkaline) in urine to correct pH In acidosis: HCO3- (basic / alkaline) is reabsorbed by the proximal and distal tubules to correct pH. Bicarbonate Specimen Collection Serum and lithium heparin plasma (arterial and whole blood measurements were discussed last chapter) Analyze ASAP (!) Keep anaerobic Don’t decap specimen Exposure to air causes a loss of CO2 = ↓TCO2 Can ↓ by 6 mmol/L per hour TCO2 Methods of Analysis ISE Method (pCO2) Combination pH electrode Silicon rubber membrane which is permeable to CO2 gas pH sensitive glass electrode detects a change in pH (↓ pH is measured) OR Gas-sensing electrode PCO2 (Bicarbonate) pH Glass Electrode ↓ in pH measured i.e. H+ ions that are produced Sample → The sample is acidified first to convert all froms of CO2 to CO2 gas Gas-Sensing CO2 Electrode The membrane is in contact with a solution that is permeable only to CO2. The change in pH of the HCO3- is detected by a pH electrode. TCO2 Methods of Analysis TCO2 /HCO3- - Clinical Significance Remember: HCO3- is basic Causes of Decreased HCO3- Causes of Increased HCO3- Metabolic acidosis Metabolic Alkalosis - Severe vomiting Renal Tubular Failure with Hyperchloremia - Excessive intake of alkali Compensated Respiratory Alkalosis Compensated Respiratory Acidosis Severe Diarrhea CO2 Reference Ranges Plasma / serum TCO2 (venous) 23 - 29 mmol/L Plasma / serum HCO3- 20 - 35 mmol/L Anion Gap ** Calculation ** Anion Gap A number that represents the concentration of unmeasured anions (proteins, phosphates, sulfates and organic acids) Not a real “gap” in anions and cations; Not an individual test Created by concentration differences between commonly measured cations (Na+ + K+) and anions (Cl- + HCO3-) Useful for: Indicating an increase in one or more unmeasured anions in serum (Metabolic Disorders) Quality control for analyzer used to measure electrolytes Anion Gap Calculation Calculation: measured cations – measured anions (Na+) - (Cl- + HCO3-) OR (Na+ + K+ ) - (Cl- + HCO3-) Anion Gap (AGap) should be positive 7 - 16 mmol/L or 10 - 20 mmol/L if K+ included Abnormal Anion Gap Low (** Rare) Multiple myeloma Instrument error High → “MUD PILES” Methanol Uremia Diabetic ketoacidosis Paraldehyde Iron, inhalants, ibuprofen Lactic acidosis Ethylene glycol, ethanol ketoacidosis Salicylates, starvation ketoacidosis Anion Gap: Demonstration of anion gap from concentrations of anions and cations in normal state and in lactic acidosis ↑ Lactate Lactic acidosis due to anaerobic metabolism in hypoxic tissue, eg. AMI ↓ HCO3- (Figure 16.9) Summary of Serum/Plasma Reference Ranges Normal Range Critical Values Na+ 135 - 145 mmol/L < 120 or > 160 mmol/L K+ 3.5 - 5.1 mmol/L < 2.0 or > 6.0 mmol/L Cl- 95 - 110 mmol/L N/A TCO2 23 - 29 mmol/L < 10 or > 40 mmol/L HCO3- 20 - 35 mmol/L < 10 or > 40 mmol/L BREAK TIME