Serum Electrolytes PDF
Document Details
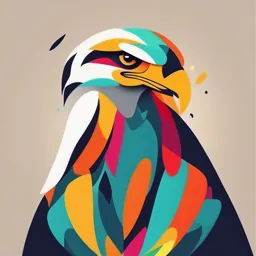
Uploaded by AdoredCharoite
Tags
Related
- Week 2 Learning Objectives PDF
- Interpret Acid Base data 2024-2025 Biochemistry Lab PDF
- Lab. 1 & 2 Serum Electrolytes PDF
- Regulation of Acid to Diuretics COMPILATION PDF
- CHEM-0045 Siemens Dimension EXL and Vista Operating Procedure (Bon Secours Mercy Health) PDF
- Disorders of Sodium and Water Homeostasis PDF
Summary
This document provides an overview of fluid and electrolyte balance in the body, including body fluid compartments, water balance, and osmolality. It also discusses relevant clinical aspects like dehydration and overhydration.
Full Transcript
Fluid and electrolyte balance Body fluid compartments The major body constituent is water. A 70 kg person contains ~42 L (60%) of water in total. 2/3 (28 L) of this is ICF & 1/3 (14 L) is ECF= which is subdivided into plasma (3.5 L) & interstitial fluid (10.5 L). Daily water intake is 1.5-2 L Wome...
Fluid and electrolyte balance Body fluid compartments The major body constituent is water. A 70 kg person contains ~42 L (60%) of water in total. 2/3 (28 L) of this is ICF & 1/3 (14 L) is ECF= which is subdivided into plasma (3.5 L) & interstitial fluid (10.5 L). Daily water intake is 1.5-2 L Women have lower water content than men (more fat) Body fluid compartments • Inlet supply = fluids taken orally or IV • Outlet = the urinary tract. • Insensible loss = surface evaporation. Body fluid compartments Assessment of volume of body fluid compartments is not undertaken by biochemistry laboratory. Patient’s state of hydration, i.e. volume of body fluid compartments, is assessed on clinical grounds. The term ‘dehydration’ → fluid loss has occurred from body compartments. Overhydration → fluid accumulates in body compartments. Clinical features of hydration problems Clinical assessments of skin turgor, eyeball tension & the mucous membranes are not always reliable. Aging affects skin elasticity & the oral mucous membranes may appear dry in patients breathing through their mouths. Body fluid compartments Intracellular fluid loss, e.g., causes cellular dysfunction → evident as lethargy, confusion & coma. Loss of blood, an ECF fluid → circulatory collapse, renal shutdown & shock. Loss of total body water will eventually produce similar effects. However, signs of fluid depletion are not seen at first since the water loss is spread across both ECF & ICF compartments. Water Importance of water in human body: Transport nutrient to the cells Determine cell volume by its transport into & out of cells Remove waste products (urine) Body coolant (sweating) Water [ions] inside the cells & in plasma is maintained by passive diffusion & active transport through ATPase-dependent ion pump Most biologic membranes are permeable to water but not ions & proteins Water & sodium output Kidneys & GI tract Sweat & expired air: ~1L daily Factors that affect the flow of water across the membrane Ions & proteins at one side of the membrane Blood pressure Control of water balance Both water intake & loss are controlled by osmotic gradient across cell membrane in brain hypothalamic osmoreceptor center that control thirst & secretion of antidiuretic hormone (ADH) = AVP (Arginine vasopressin hormone) Thirst is the major defense against hyperosmolality & hypernatremia Control of water balance Antidiuretic hormone Is a polypeptide with t1/2 of 20 min Synthesized by hypothalamus & secreted by posterior pituitary gland 2% increase in osmolality lead to 4 times increase in ADH Low blood pressure & severe hypovolemia stimulate ADH release Stress due to vomiting, nausea & pain may ADH secretion ADH acts by increasing the reabsorption of water in cortical and medullary collecting tubules Control of water balance Control of water balance Hypernatremia rarely occurs in a person with a normal thirst mechanism & access to water, it becomes a concern in: – Infants – Unconscious patients – Anyone who is unable to drink or ask for water. – People older than 60 (osmotic stimulation of thirst progressively ↓) – In the older patient with illness & diminished mental status, dehydration becomes increasingly likely A patient with diabetes insipidus (no ADH) may excrete 10 L of urine day, but as water intake matches output, plasma Na remains normal. Osmolality Physical property based on [solutes] (mmol)/kg of solvent (w/w). This affect different properties of solution as: Freezing point depression Vapor pressure decrease The osmolality of the ICF is normally the same as the ECF. Increase in osmolality will induce secretion of ADH while decrease in osmolality will lead to turning off ADH secretion Significance of osmolality Because it is the parameter by which the hypothalamus responds It is affected by Na concentration as it represents 90% of osmotic activity in plasma Na concentration is affected by blood volume Determination of Osmolality Osmolality may be measured in serum or urine. Plasma use is not recommended because osmotically active substances may be introduced into the specimen from the anticoagulant. Samples must be free of particulate matter to obtain accurate results. Turbid serum and urine samples should be centrifuged before analysis Osmolality This simple formula only holds if the serum concentration of urea and glucose are within the reference intervals. Osmolal gap is the difference between the measured osmolality and the calculated osmolality Osmolal gap indirectly indicates the presence of osmotically active substances other than sodium, urea, or glucose, such as ethanol, methanol, ethylene glycol, lactate, or β- hydroxybutyrate. Determination of Osmolality Osmometers are standardized by NaCl solution, then the freezing point of the sample is measured and this is compared to the calculated value as double of serum sodium or according to the following 2 formulas: Importance of electrolytes Ions are capable of carrying an electric charge (cations or anions) The dietary requirements for electrolytes vary some need to be consumed only in small amounts. Others, e.g. Ca, K, & Phosphorus, are excreted continuously and must be ingested regularly to prevent deficiency Sodium (Na+) is the principal extracellular cation, & potassium (K+), the principal intracellular cation. Inside cells the main anions are protein & phosphate, whereas in the ECF chloride (Cl−) and bicarbonate (HCO3−) predominate. Normal ranges Importance of electrolytes They are involved in many processes: Volume & osmotic pressure (Na, K, Cl) Myocardial rhythm & contractility (K, Mg, Ca) Cofactors in enzyme activation (Mg, Ca, Zn) Regulation of ATPase ion pump (Mg) Acid-base balance (HCO3, K,Cl) Blood coagulation (Ca, Mg) Neuromuscular excitability (K, Ca, Mg) Production & use of ATP from glucose (Mg, PO4) Electrolytes, Sodium (Na) Total body Na of a 70 kg man is 3700 mmol → ~75% is exchangeable. 1/4 body Na is non-exchangeable = incorporated into tissues e.g. bone & has a slow turnover rate. Most of the exchangeable Na is in ECF. Na daily intake is ~60-150 mmol Na balance is regulated by blood flow & aldosterone (hormone secreted by adrenal cortex) Electrolytes, Sodium (Na) Na is the most abundant cation in the ECF (90% of all extracellular cations) & largely determines the osmolality of the plasma. To prevent equilibrium from occurring, active transport systems, such as ATPase-dependent ion pumps (moves 3 Na out of cell for each 2 K moving into the cell) are present in all cells The normal range of Sodium (133–146 mmol/L) Regulation of sodium Plasma Na conc. depends on: the intake & excretion of water & renal regulation of Na. Most Na excretion is via kidneys. Some Na is lost in sweat (~5 mmol/day) & in feces (~5 mmol/day). In disease, GIT is often the major route of Na loss. Regulation of sodium Three processes are of primary importance: (1) intake of water in response to thirst, as stimulated or suppressed by plasma osmolality (2) excretion of water, largely affected by ADH release in response to changes in either blood volume or osmolality (3) blood volume status, which affects Na excretion through aldosterone, angiotensin II, & ANP (atrial natriuretic peptide). Regulation of sodium Kidneys have the ability to conserve or excrete large amounts of Na, depending on Na content of ECF & blood volume. Normally, 60-75% of filtered Na is reabsorbed in proximal tubule Some Na is also reabsorbed in the loop & distal tubule (controlled by aldosterone) & exchanged for K in the connecting segment & cortical collecting tubule. Regulation of sodium Urinary sodium output is regulated by two hormones: 1- Aldosterone . 2- Atrial natriuretic peptide. Aldosterone It is released from the adrenal cortex in response to activation of the renin–angiotensin system, stimulates Na reabsorption in the distal parts of the distal convoluted tubules & collecting ducts and is the major factor controlling renal Na excretion. Renin secretion is stimulated primarily by a decrease in renal perfusion secondary to a decrease in arterial blood volume. Atrial natriuretic peptide (ANP) Is 28 a.a. peptide secreted by the cardiac atria in response to atrial stretch following a rise in atrial pressure (e.g. due to ECF volume expansion). ANP acts both directly by inhibiting distal tubular Na reabsorption & through decreasing renin (& hence aldosterone) secretion It also antagonizes the pressor effects of norepinephrine (noradrenaline) & angiotensin II (and thus tends to increase GFR) & has a systemic vasodilatory effect. It provides ‘fine tuning’ of Na homoeostasis but is more important in pathological states than physiologically. Sodium and ECF volume The volume of the ECF is directly dependent on the total body Na content as water intake and loss are regulated to maintain a constant ECF osmolality, and hence [Na] In health, the response to an increase in ECF volume (e.g. as a result of infusing isotonic saline) is a decrease in secretion of aldosterone (with no change in that of vasopressin), leading to natriuresis. In Na depletion, an increase in aldosterone secretion leads to Na retention: free water retention is only stimulated in severe sodium depletion. In general, the control mechanisms for ECF volume respond less rapidly than the control mechanisms for ECF osmolality. Hyponatraemia Hyponatraemia is defined as a serum Na concentration below the reference interval of 133–146 mmol/L. Levels below 130 mmol/L are clinically significant. Serum concentration of Na is simply a ratio, of Na (millimoles) to water (litres), and hyponatraemia can arise either because of loss of Na ions (water is lost with it) or retention of water (dilutes Na). Causes of hyponatraemia: Water imbalance Increased water retention (decreased water excretion = water retention) HM Renal failure or Nephrotic syndrome Hepatic cirrhosis Congestive heart failure syndrome of inappropriate antidiuresis (SIAD) Most hyponatraemic patients due to water retention have SIAD. Causes of hyponatraemia: Water imbalance SIAD is encountered in conditions e.g. infection, malignancy, chest disease, & trauma (e.g. surgery); also is drug-induced. SIAD results from inappropriate secretion of AVP, which is associated with pulmonary disease, malignancies, CNS disorders, infections. Excess water intake (polydipsia, increased thirst) if water intake was chronic. In a normal individual, excess intake will not affect Na levels. Causes of hyponatraemia: Sodium loss Pathological Na loss, either from GIT or urine. GI losses include vomiting and diarrhea Urinary loss may result from: mineralocorticoid deficiency (especially aldosterone) or from drugs that antagonize aldosterone, e.g. spironolactone. Hypoadrenalism Potassium difficiency (exchange in kidney) Diuretic use (thiazide) Ketonuria (sodium lost with ketones) Salt losing nephropathy (renal tubular disorders) Severe burns Pseudohyponatraemia Hyponatraemia sometimes reported in patients with severe hyperproteinaemia or hyperlipidaemia. Na & other electrolytes are distributed in water fraction only, & these patients have a normal Na conc. in their plasma water. methods used in analytical instruments measure Na conc. in total plasma volume (no account of a water fraction)→ artificially low Na. Normal serum osmolality with severe hyponatraemia = pseudohyponatraemia. Assessed by calculating osmolal gap Classification of hyponatraemia by osmolality With Low Osmolality • Increased sodium loss • Increased water retention With Normal Osmolality (Increased nonsodium cations) • Lithium excess • Increased -γ-globulins-cationic (myeloma) • Severe hyperkalemia • Severe hypermagnesemia • Severe hypercalcemia • Pseudohyponatremia • Hyperlipidemia • Hyperproteinemia • Pseudohyperkalemia as a result of in vitro hemolysis With High Osmolality • Hyperglycemia • Mannitol infusion Symptoms of hyponatraemia They reflect neurological dysfunction resulting from cerebral overhydration induced by hypo-osmolality. Include nausea, malaise, headache, lethargy & a reduced level of consciousness. Seizures, coma & focal neurological signs are not seen until Na conc. is 115 mmol/L. Symptoms of hyponatremia Symptoms depend on the serum level. Between 125 and 130 mmol/L: symptoms are gastrointestinal below 125 mmol/L: More severe neuropsychiatric symptoms are seen including nausea and vomiting, muscular weakness, headache, lethargy, and ataxia. More severe symptoms also include seizures, coma, and respiratory depression. Treatment of hyponataremia Hypovolaemic patients are depleted & should be given Na. Na Normovolaemic patients are likely to be retaining water & should be fluid restricted. Oedematous patients have an excess of both total body sodium & water → given a diuretic to induce natriuresis, & be fluid restricted. More aggressive treatment (usually requiring hypertonic saline) may be indicated if symptoms attributable to hyponatraemia are present, or Na conc. is 110 mmol/L. Hypernatraemia It is in serum Na conc. above reference interval of 133–146 mmol/L It develops either because of water loss or sodium gain. Causes of hypernatraemia 1) Excess Water Loss Diabetes insipidus Prolonged diarrhea Severe burns 2) Decreased Water Intake Older persons Mental impairment Renal tubular disorder Profuse sweating Poorly controlled DM Infants 3) Increased Na Intake or Retention (salt poisoning) HyperaIdosteronism Na bicarbonate excess Dialysis fluid excess Clinical features of hypernatraemia mild hypernatraemia (<150 mmol/L) more severe hypernatraemia (150-170 mmol/L) obvious dehydration → ECF volume ↓ (loss of both water & Na) pure water loss is likely if the clinical signs of dehydration are mild in relation to the severity of the hypernatraemia. This is because pure water loss is distributed evenly throughout all body compartments (ECF and ICF). gross hypernatraemia (>180 mmol/L) salt poisoning if there is little or no clinical evidence of dehydration; the amount of water that would need to be lost to elevate the sodium to this degree should be clinically obvious. Salt gain may present with clinical evidence of overload, such as raised jugular venous pressure or pulmonary oedema. Symptoms of hypernatraemia Involve the central nervous system (CNS) as a result of the hyperosmolar state which include: • Altered mental status • Irritability • Seizures • Fever, nausea or vomiting • Lethargy • Restlessness. • Muscle twitching, hyperreflexes • Difficult respiration, & ed thirst Serum sodium of more than 160 mmol/L is associated with a mortality rate of 60-75% Hypernatraemia related to urine osmolality Urine Osmolality <300 mOsm/kg Diabetes insipidus (impaired secretion of ADH or kidneys cannot respond to ADH) Urine Osmolality 300-700 mOsm/kg Partial defect in ADH release or response to ADH Osmotic diuresis Urine Osmolality >700 mOsm/kg Loss of thirst Insensible loss of water (breathing, skin) GI loss of hypotonic fluid Excess intake of sodium Treatment of hypernatraemia If it is due to pure water loss → given water orally, or IV as 5% dextrose. Dehydration (indicate loss of Na) → Na should be administered. Salt poisoning & Na overload → diuretics & natriuresis replaced with water. Caution with the use of IV dextrose in salt-poisoned patients; they are volume-expanded already & susceptible to pulmonary oedema. Appropriate management of fluid administration is critical. It must be corrected gradually to avoid cerebral edema & death. the maximal rate should be 0.5 mmol/L per hour. Chronic hypernatremia in an alert patient is indicative of hypothalamic disease Determination of sodium Sodium can be measured in serum, plasma, and urine. When plasma is used, lithium heparin, ammonium heparin, and lithium oxalate are suitable anticoagulants. Hemolysis does not cause a significant change in serum or plasma values as a result of decreased levels of intracellular sodium. But with marked hemolysis, levels may be decreased (dilutional effect). Whole blood samples may be used with some analyzers. The specimen of choice in urine Na analyses is a 24-hour collection. Sweat is also suitable for analysis. Potassium K disorders are important because of the role K plays in determining the resting membrane potential of cells. Changes in plasma K mean that ‘excitable’ cells (nerve & muscle), may respond differently to stimuli. In heart → can be fatal e.g. arrhythmias. Many cellular functions require low ECF conc. of K (only 2% of total K in plasma) → plasma K conc. is a poor indicator of the total body content. Serum potassium concentration is normally kept within a tight range (3.5– 5.3 mmol/L). Factors affecting plasma K conc. The intracellular [K+] is large & provides a reservoir for the extracellular compartment → changes in water balance have little direct effect on the plasma [K+], unlike plasma [Na+]. The normal K intake is ~60–100 mmol/day. K enters & leaves the extracellular compartment by three main routes: – intestine (absorbed in small intestine) – kidneys (filtered by glomeruli & almost completely reabsorbed in proximal tubules). K is secreted in distal tubules & collecting ducts in exchange for Na+; H+ compete with K+. – membranes of all other cells. factors that determine potassium excretion: • glomerular filtration rate and the plasma potassium concentration. • An additional factor often implicated in hyperkalaemia and hypokalaemia is redistribution of potassium. • Nearly all of the total body potassium (98%) is inside cells. If, for example, there is significant tissue damage, the contents of cells, including potassium, leak out into the extracellular compartment, causing potentially dangerous increases in serum potassium Relationship b/n H& K ions Extracellular [H+] affects entry of K into all cells. Changes in relative proportions of K+ & H+ in distal renal tubular cells affect the urinary loss of K. There is a reciprocal relationship between K+ & H+: In acidosis, increased loss of K from cells into the ECF coupled with reduced urinary secretion of K causes hyperkalaemia. In alkalosis, net increased uptake of K into cells & increased urinary K loss cause hypokalaemia. Hyperkalaemia Hyperkalaemia is the commonest & most serious electrolyte emergency encountered in clinical practice. Hyperkalemia causes muscle weakness that may be preceded by paresthesia. However, the first manifestation may be cardiac arrest. If severe (>7.0 mmol/L) → immediately life-threatening → cardiac arrest. ECG changes in hyperkalaemia may mimic other conditions e.g. myocardial infarction → important to check serum [K] in patients after cardiac arrest. Causes of hyperkalaemia Hyperkalaemia can be categorized as due to increased intake, redistribution or decreased excretion. Increased intake: K intake may result in dangerous hyperkalaemia, particularly with impaired renal function. e.g. many oral drugs are administered as K salts. blood products may give rise to hyperkalaemia (stored RBCs release K down its concentration gradient). Decreased excretion: Renal failure ( GFR). Hypoaldosteronism( adrenal insufficiency or drugs). Redistribution out of cells: K release from damaged cells. – in rhabdomyolysis (skeletal muscle is broken down), extensive trauma, or tumor lysis syndrome. Metabolic acidosis. – There is a reciprocal relationship between K & H ions. Insulin deficiency. – Insulin stimulates cellular uptake of K→ used in ttt of severe hyperkalaemia Hyperkalaemic periodic paralysis. – A rare familial disorder with autosomal dominant inheritance → recurrent attacks of muscle weakness or paralysis, precipitated by rest after exercise. Pseudohyperkalaemia. Pseudohyperkalaemia It is in measured [K] resulting from K movement out of cells during or after the drawing of the blood specimen. It should be suspected when there is no apparent cause for hyperkalemia, & no ECG changes reflecting altered cardiac muscle contractility Occur if there has been a delay in separating plasma from cells, esp. if blood sample has been refrigerated, when activity of Na/K-ATPase pump is slowed. The commonest cause is haemolysis → due to mechanical trauma during venopuncture → release of K from RBCs Small amount of K is released from WBCs & platelets during normal clotting In patients with grossly elevated white cell or platelet counts due to haematological malignancies→ amount of K released is much greater Also, blood tubes contaminated with K-EDTA (ethylenediamine tetra-acetic acid): blood samples for K assay should usually be collected in lithium heparin tubes. Causes of hyperkalaemia Decreased excretion: In practice, all patients with hyperkalaemia will have a reduced GFR. Renal failure. – Kidneys not be able to excrete a K load → hyperkalaemia. Hypoaldosteronism. – Aldosterone stimulates Na reabsorption at expense of K & H. Deficiency results in loss of Na, causing ↓ in GFR → retention of K & H ions. – hyperkalaemia due to hypoaldosteronism is seen with the use of ACE inhibitors & angiotensin receptor blockers (ARBs) – spironolactone & K-sparing diuretics antagonize aldosterone effect Symptoms of hyperkalaemia Muscle weakness at [K] of 8 mmol/l Tingling, numbness and mental confusion Cardiac arrhythmia & cardiac arrest at conc of 6-7 mmol/l, which alter ECG Fatal cardiac arrest at conc > 10 mmol/l Treatment of hyperkalemia Treatment should be started if K>6-6.5 or if ECG changes occur An infusion of Ca gluconate may be given to reduce threshold potential of myocardial cells The commonest form of treatment for acute hyperkalemia is infusion of bicarbonate, insulin & glucose to move K ions into cells. K can be removed by loop diuretics in good renal function Na polystyrene sulphonate enema which binds K secreted in colon Dialysis is frequently necessary to treat severe hyperkalemia. Hypokalemia It is a plasma [K] below the lower limit of the reference range Hypokalemia can occur with GI or urinary loss of K or with increased cellular uptake of potassium. Hypokalemia, Common causes Common causes include: vomiting & diarrhoea; & diuretics. Increased urinary K excretion in the face of potassium depletion suggests urinary loss rather than redistribution or gut loss. Equally, low or undetectable urinary K in this context indicates opposite. Where the cause is not immediately obvious, urine potassium measurement may help to guide investigations. Hypokalemia causes: Reduced intake: This is a rare cause of hypokalaemia. Renal retention of potassium in response to reduced intake ensures that hypokalaemia occurs only when intake is severely restricted. Since potassium is present in meat, fruit and some vegetables, marked potassium restriction is difficult to maintain. Hypokalaemia should be a consideration when severely hypocaloric diets are prescribed to bring about rapid weight loss. Redistribution into cells: 1. Metabolic alkalosis. 2. Treatment with insulin. 3. Refeeding [‘refeeding syndrome’ was first described in prisoners of war, anorexia nervosa, cancer, alcoholism and postoperative patients]. 4. β-Agonism [salbutamol (used to treat asthma) or dobutamine (heart failure)]. 5. Treatment of anaemia [megaloblastic anaemia]. 6. Hypokalaemic periodic paralysis. Increased losses: Gastrointestinal The common causes (diarrhoea and vomiting) are obvious. In cholera (associated with massive fluid loss from the gut), daily potassium losses may exceed 100 mmol, compared with ~5 mmol normally. Less frequently, chronic laxative abuse may be responsible Urinary Diuretics [Both loop diuretics and thiazide diuretics]. Mineralocorticoid excess. Hypomagnesaemia. Tubulopathies [The most common causes are chemotherapeutic agents, especially platinum containing drugs]. Symptoms of hypokalemia Mild hypokalemia (3-3.4 mmol/L) is asymptomatic Muscle weakness and paralysis that interfere with breathing Dangerous for patients with cardiovascular disease as it may cause arrhythmia leading to sudden death in some patients Treatment K salts are unpleasant to take orally & are usually given prophylactically in an enteric coating. Severe K depletion often has to be treated by intravenous potassium Intravenous potassium should not be given faster than 20 mmol/hour except in extreme cases and under ECG monitoring. Mild chronic hypokalemia can be treated by diet rich K (dried fruits, nuts, banana and orange juice