Silicon Etching - 2024 (PDF)
Document Details
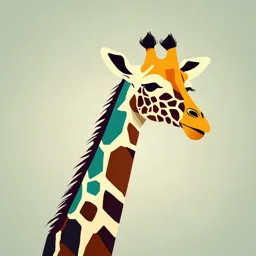
Uploaded by TidyHammeredDulcimer
University of Michigan
Khalil Najafi
Tags
Summary
This document introduces MEMS (Microelectromechanical Systems) and focuses on silicon etching techniques. It reviews different etching methods, etch stops, and wafer bonding, along with common micromachining technologies. The document also explores MEMS-IC integration. It's suitable for readers interested in microfabrication or MEMS design.
Full Transcript
Silicon Etching Khalil Najafi Center...
Silicon Etching Khalil Najafi Center for Wireless Integrated MicroSensors and Systems (WIMS2) University of Michigan 1301 Beal Avenue Ann Arbor, Michigan 48109-2122 Tel: (313) 763-6650, FAX: (313) 763-9324 e-mail: [email protected] oSensin Micr g& ed at Sy gr ste Wi r e l e s s I n t e ms Introduction to MEMS ã Copyright: Khalil Najafi, Do not duplicate without written permission of authors Si Etching, page 1 Outline Review of important technologies used in MEMS: – Silicon etching: isotropic, anisotropic, dry, other techniques – Etch stops (concentration dependent, electrochemical, dielectric) – Wafer bonding (anodic, fusion, eutectic, polymer) Review of most common micromachining technologies: – Silicon bulk micromachining: wet and dry – Surface (sacrificial) micromachining: polysilicon, metals, polymers, etc. – Molding: electroplating, hot embossing, etc. – 3-D machining technologies: micro-milling, EDM, laser machining, etc. MEMS-IC integration techniques and issues oSensin Micr g& ed at Sy gr ste Wi r e l e s s I n t e ms Introduction to MEMS ã Copyright: Khalil Najafi, Do not duplicate without written permission of authors Si Etching, page 2 Silicon Micromachining We have reviewed the basic microfabrication steps used in the manufacture of integrated circuits. These techniques allowed us to deposit, pattern, and modify the properties of a variety of thin films, including insulators, metals (conductors), and semiconductors. In IC fabrication, we are primarily interested in the electrical and electronic properties of these materials. We use these materials to build transistors, capacitors, and resistors, and use them in combination to build complex circuits consisting of millions/billions of these devices. One of the primary features of planar microfabrication technologies is their ability to form millions of electronic devices on a small substrate and then utilize these simple functions to implement a complex task, cost effectively. MEMS utilizes many of these same techniques, and attempts to achieve higher level of overall functional complexity by employing thousands of mechanical structures in a small area, or simply to make one mechanical structure with precision and use it for sensing and actuation. We need to be able to make these physical structures out of the same materials, and more, and using the same basic techniques used in IC fabrication. What materials are used and how do we make physical “microstructures” out of these films to make a variety of MEMS devices? oSensin Micr g& ed at Sy gr ste Wi r e l e s s I n t e ms Introduction to MEMS ã Copyright: Khalil Najafi, Do not duplicate without written permission of authors Si Etching, page 3 Analog Devices MEMS Accelerometers Similar accelerometers from several manufacturers are in your cars, phones, toys, watches, etc. Mechanical components (proof mass) fabricated using surface micromachining oSensin Micr g& ed at Slide courtesy of Dr. Kevin Chau, Formerly with Analog Devices, Inc. Sy gr ste Wi r e l e s s I n t e ms Introduction to MEMS ã Copyright: Khalil Najafi, Do not duplicate without written permission of authors Si Etching, page 4 Omron Blood Pressure Monitoring Cuffs Omron Simplifies Home Blood Pressure Monitoring Fig. 2 ASIC-equipped multi-element MEMS pressure sensor In other words, if the blood vessel can be pressed with appropriate pressing force, the pressing force (i.e., the pressure detected by the pressure sensors) becomes equivalent to the blood pressure. Therefore, if the pressure sensors are retained with the optimum pressing force, it is possible to measure the blood pressure continuously without interrupting the blood flow and to measure the pressure pulse waveform which is equivalent to the blood pressure waveform measured by inserting a catheter into the blood vessel. at https://www.eetimes.eu/omron-simplifies-home-blood-pressure-monitoring/ ed Micr oSensin g& Sy gr ste Wi r e l e s s I n t e ms Introduction to MEMS ã Copyright: Khalil Najafi, Do not duplicate without written permission of authors Si Etching, page 5 Capacitive Pressure Sensing In Most Commercial Blood Pressure Monitoring Devices A thin, flexible diaphragm (membrane) deflects in response to changing pressure across it. The diaphragm is separated from a fixed electrode by a small air gap. As the diaphragm deflects the capacitance changes, and this change can be measured electronically to provide a measure of changing pressure. Pressure Sensing Membrane: Pressure ~1µm thick, 1mm wide Varying Gap oSensin Micr g& ed at Sy gr ste Wi r e l e s s I n t e ms Introduction to MEMS ã Copyright: Khalil Najafi, Do not duplicate without written permission of authors Si Etching, page 6 MEMS Capacitive Microphones in Your Cell Phones The Knowles MEMS Micrphone oSensin Micr g& ed at Sy gr ste Wi r e l e s s I n t e ms Introduction to MEMS ã Copyright: Khalil Najafi, Do not duplicate without written permission of authors Si Etching, page 7 Micro Structures To build MEMS, we need to make a variety of microstructures of different shapes, sizes, and materials. Structures include beams, membranes (diaphragms), bridges, tubes (pipes), tall pillars, etc. It is also often needed to form parts that have holes and recesses in them or maybe parts that are 3-dimensional or have curved parts. We need to develop techniques that enable us to fabricate these structures in or on a wafer, preferably silicon. Narrow, Tall Structures Suspended Suspended Deep, Narrow Hole Bridge/membrane Thin Cantilever Beam Membrane Shallow, Large (diaphragm) Recess oSensin Micr g& ed at Sy gr ste Wi r e l e s s I n t e ms Introduction to MEMS ã Copyright: Khalil Najafi, Do not duplicate without written permission of authors Si Etching, page 8 Micro Structures One class of structures can be created on top of a silicon wafer by depositing different materials and patterning them to create the desired shapes. For these types of structures, we don’t need to do anything to the underlying wafer since the wafer is acting as a support substrate on which these structures sit. This substrate might be silicon, but it could also be any other substrate/wafer that can be processed. We will come back and review how these kinds of structures can be built later. Narrow, Tall Structures Suspended Cantilever Beam Suspended Bridge/membrane ~ 1-5µm thick, 100µm long ~ 1-5µm thick, 100µm-several millimeters long Support Substrate oSensin Micr g& ed at Sy gr ste Wi r e l e s s I n t e ms Introduction to MEMS ã Copyright: Khalil Najafi, Do not duplicate without written permission of authors Si Etching, page 9 Micro Structures Another class of structures is fabricated from the silicon wafer itself, but selectively removing (i.e., etching) parts of the wafer and leaving behind the rest. Some of these shapes or structures are shown below, such as a shallow recess, a deep hole, a thin diaphragm, or a tall vertical beam. To form any of these features, we have to be able to etch the silicon wafer itself. In other words we need to remove some silicon from the ”bulk” of the silicon wafer. This is called “bulk” silicon etching. Deep, Narrow Hole Thin Tall narrow Membrane Shallow, Large post/beam (diaphragm) Recess Silicon Substrate oSensin Micr g& ed at Sy gr ste Wi r e l e s s I n t e ms Introduction to MEMS ã Copyright: Khalil Najafi, Do not duplicate without written permission of authors Si Etching, page 10 Common MEMS Materials Many materials are used in microfabrication and micromachining. Many of these materials were discussed before. The set of materials used in micromachining is increasing and many new materials are being employed for use in MEMS. We cannot discuss them all here. Therefore, we concentrate mostly on those that are commonly used in MEMS. Common materials: – Single-Crystal Silicon SCS (bulk silicon) – Polycrystalline silicon – Silicon dioxide – Silicon Nitride – Metals: Al, Au, Ni, Cu, … – Polymers: photoresist, polyimide, Parylene, plastics, wax, PDMS – Others: glass, ceramics, SiC, polycrystalline diamond,... oSensin Micr g& ed at Sy gr ste Wi r e l e s s I n t e ms Introduction to MEMS ã Copyright: Khalil Najafi, Do not duplicate without written permission of authors Si Etching, page 11 Single-Crystal Silicon Silicon is an excellent MEMS material because of its electrical & mechanical properties. It can be precisely machined (etched) in a variety of wet and dry etchants, has extremely reproducible and stable properties, and is mechanically rugged. Because of its crystalline structure, SCS is brittle and when stressed it will break along a preferred crystallographic plane. Silicon is an elastic (compliant) material, meaning that when stressed it bends, and if stressed too much it will break. It is not ductile, that is it will not plastically deform, and always goes back to original shape when the stress is removed (very much like glass). This is a very important property which makes silicon an excellent material for micromechanical devices. Properties of Si change along the different crystallographic planes. This is especially important for MEMS devices. We will see how the crystalline structure of Si affects these properties and the fabrication techniques. Looking into the (100) direction oSensin Micr (100) Plane g& ed (110) Plane at Sy gr ste Wi r e l e s s I n t e ms Introduction to MEMS ã Copyright: Khalil Najafi, Do not duplicate without written permission of authors Si Etching, page 12 Si Etching (Micromachining) Wet Etching – Isotropic – Anisotropic (orientation-dependent) – Concentration-dependent Heavily-doped etch stops Lightly-doped etch stops – Electrochemical Etch Stop pn junction – Dielectric Etch Stop Dry Etching – Plasma etching at ed Micr oSensin g& – Deep Reactive Ion Etching (DRIE) Sy gr ste Wi r e l e s s I n t e ms Introduction to MEMS ã Copyright: Khalil Najafi, Do not duplicate without written permission of authors Si Etching, page 13 Basic Principles of Chemical Etching Most etching processes involve one or more chemical reactions. The product formed from these chemical reactions must be soluble in the etchant medium, are at least must be carried away from the surface of the device by the etchant medium. One of the various types of reactions that may be involved is oxidation-reduction. This is very common in etching of silicon and we will concentrate mostly on this particular reaction. The oxidation-reduction (or redox) involves converting the material that is being etched to a soluble higher oxidation state, as represented by the following simple formula. M ----> Mn+ + ne- Note that the redox etching can occur either in a completely chemical system by the use of certain chemical oxidizing agents, or in an electrochemical cell. We will use both of these techniques, chemical and electrochemical, etching in micromachining of silicon. oSensin Micr g& ed at Sy gr ste Wi r e l e s s I n t e ms Introduction to MEMS ã Copyright: Khalil Najafi, Do not duplicate without written permission of authors Si Etching, page 14 Chemical Etching of Si in HF Acid One of the common etchants of silicon is hydrofluoric acid (HF), often mixed in with nitric acid (HNO3). The nitric acid is the oxidizing agent, and the HF acid is the reducing agent. So, the etching process will proceed as follows: Si + HNO3 + 6HF ---> H2SiF6 + HNO2 + H2O + H2 (bubbles) The by-products are all water soluble and are carried away by the etchant. The etch rate depends on the relative composition of the etchant. If there is too little nitric acid, then the etch rate is oxidation rate limited because there is not enough oxidant to oxidize the silicon. If there is too little HF, then the etch rate is reduction limited because there is not enough HF to etch away the oxide. The HF+nitric acid mixture is a very common silicon etchant and is an isotropic etchant of silicon. That is, this etchant etches silicon independent of crystal orientation. oSensin Micr g& ed at Sy gr ste Wi r e l e s s I n t e ms Introduction to MEMS ã Copyright: Khalil Najafi, Do not duplicate without written permission of authors Si Etching, page 15 Isotropic Silicon Etching (ISE) Si can be etched isotropically (equal etch rate in all directions) in a mixture of HF+HNO3, and some acetic (vinegar) acid (this mixture is sometimes referred to as HNA). This etch was developed in the 1960’s as the solid-state circuits industry was working on the development of beam-lead technology for building high-density circuits. The etch removes n- or p-type silicon in all directions at about the same rate. The etch does depend on agitation. The more agitation there is during the etch the larger the undercut under the masked regions. The etch attacks most materials (HF is nasty acid that attacks many materials). For shallow etches, silicon nitride can be used as a mask, for deep etches sometimes it is required to use Cr/Au. HF does not significantly attack silicon directly but the HF+HNO3 mixture is a strong etchant of Si. Mask (usually nitride) Si substrate Si substrate With Agitation With No Agitation M. Baranski et al. J. Electrochem. Soc. at e dM icr oSensin g& 2011;158:D681-D688 Sy gr ste Wi r e l e s s I n t e ms Introduction to MEMS ã Copyright: Khalil Najafi, Do not duplicate without written permission of authors Si Etching, page 16 Cross-Sectional Views As Etch Proceeds Etch rate is not dependent on orientation. Etch proceeds equally in all directions. Si substrate Si substrate Si substrate 1 2 3 Si substrate Si substrate Si substrate 4 5 6 oSensin Micr g& ed at Sy gr ste Wi r e l e s s I n t e ms Introduction to MEMS ã Copyright: Khalil Najafi, Do not duplicate without written permission of authors Si Etching, page 17 Isotropic Si Etching Mask material is nitride or metal Etch is isotropic (i.e., same etch rate) in all directions Cross-sectional view of the at ed Micr oSensin g& etched regions. Sy gr ste Wi r e l e s s I n t e ms Introduction to MEMS ã Copyright: Khalil Najafi, Do not duplicate without written permission of authors Si Etching, page 18 ISE Etchants The overall reaction of HNA with Si is: Si+HNO3+6HF ---> H2SiF6 + HNO2 + H2O + H2 (bubbles) The etch is carried out in a beaker where nitrogen bubbles are introduced to facilitate the transport of etchants and byproducts to and from the wafer. Etchant Temp. Etch Rate Mask Concentration °C µm/min. Material Dependence HF+HNO3+Acetic 25 0.7-3 SiO2, Si3N4 etches n or p >1017 cm-3 (1:3:8) HF+HNO3+Acetic 25 ~4 Si3N4, Cr/Au None (1:2:1) HF+HNO3+Acetic 25 ~7-12 Si3N4, Cr/Au None, etches all (1:8:3.3) Hard to mask concentrations at same rate Isotropic etching has a number of applications: – Removal of damage or polishing Si – Creating structures with round surfaces – Thinning silicon wafers – Delineation of electrical junctions or defects oSensin Micr g& ed at Sy gr ste Wi r e l e s s I n t e ms Introduction to MEMS ã Copyright: Khalil Najafi, Do not duplicate without written permission of authors Si Etching, page 19 Pressure Sensor with Integrated Readout Circuit Fabricate circuits on the front of wafer IC’s Etch using HNA from the back Etch has to be timed, no self stop Hard to control, non-uniform Not very reproducible or uniform Si Isotropically-Etched Diaphragm Top View, light thru diaphragm Top View showing IC oSensin Micr g& ed at Courtesy of Ken Wise, University of Michigan, circa 1970s! Sy gr ste Wi r e l e s s I n t e ms Introduction to MEMS ã Copyright: Khalil Najafi, Do not duplicate without written permission of authors Si Etching, page 20 Si Etching (Micromachining) Wet Etching – Isotropic – Anisotropic (orientation-dependent) – Concentration-dependent Heavily-doped etch stops Lightly-doped etch stops – Electrochemical Etch Stop pn junction – Dielectric Etch Stop Dry Etching – Plasma etching at ed Micr oSensin g& – Deep Reactive Ion Etching (DRIE) Sy gr ste Wi r e l e s s I n t e ms Introduction to MEMS ã Copyright: Khalil Najafi, Do not duplicate without written permission of authors Si Etching, page 21 Anisotropic Silicon Etching (ASE) A number of etchants etch silicon at different rates along different crystal orientations. These etchants are referred to as orientation-dependent etchants because they etch the different crystallographic planes preferentially. Remember the Miller representation of the three primary planes [(100), (110), and (111)] in silicon. It is believed that ASE occurs because these different planes represent a different density of silicon atoms to the etchant as each plane is exposed. In addition, the atoms at the surface for each plane have different number of dangling bonds (bonds that are not complete). Those planes with more dangling bonds will etch faster because the Si atoms are more weakly bound to the rest of the lattice. Based on this, the etch rates of the three primary planes are as follows: (111) notation to identify a family of equivalent directions (for example ). Use the ( ) notation to identify a specific plane (for example (113)) Use the { } notation to identify a family of equivalent planes (for example {311}). A bar above a index is equivalent to a minus sign. z 1 (Planes) y 1 x 1 [Directions] oSensin Micr g& ed at Sy gr ste Wi r e l e s s I n t e ms Introduction to MEMS ã Copyright: Khalil Najafi, Do not duplicate without written permission of authors Si Etching, page 25 Miller Indices for a Simple Cubic Structure (xyz) values are the inverse of the coordinate of the intercepts of a given plane with the three axes; For example (100) represents the plane that intersects the x axis and runs parallel to the yz plane. [xyz] is a given crystal direction and represents the direction of the vector perpendicular to the plane (100). As we will see later, properties of Si change along these different planes. z z z 1 1 1 (xyz) (100) (110) (111) Plane y Plane y Plane y 1 1 1 x x x 1 1 1 at ed Micr oSensin g& Direction Direction Direction Sy gr ste Wi r e l e s s I n t e ms Introduction to MEMS ã Copyright: Khalil Najafi, Do not duplicate without written permission of authors Si Etching, page 26 Equivalent Planes All 6 Different (100) Planes Six of the 8 Different (111) Planes Four of the 6 Different (110) Planes Micr oSensin g& http://www.doitpoms.ac.uk/tlplib/miller_indices/lattice_examples.php ed at Sy gr ste Wi r e l e s s I n t e ms Introduction to MEMS ã Copyright: Khalil Najafi, Do not duplicate without written permission of authors Si Etching, page 27 All {100} Planes oSensin Micr g& ed at Sy gr ste Wi r e l e s s I n t e ms Introduction to MEMS ã Copyright: Khalil Najafi, Do not duplicate without written permission of authors Si Etching, page 28 All {110} Planes oSensin Micr g& ed at Sy gr ste Wi r e l e s s I n t e ms Introduction to MEMS ã Copyright: Khalil Najafi, Do not duplicate without written permission of authors Si Etching, page 29 All {111} Planes (-1,-1,1) (-1,1,1) (-1,1,-1) (1,-1,-1) (-1,-1,-1) oSensin Micr g& ed at Sy gr ste Wi r e l e s s I n t e ms Introduction to MEMS ã Copyright: Khalil Najafi, Do not duplicate without written permission of authors Si Etching, page 30 Examples of Different Planes Several planes are shown below with their Miller indices. Note that Miller indices are usually expressed using integers. z (122) Plane (210) Plane Obtained from Intercept Obtained from Points (1,1/2,1/2) Intercept points (1/2,1,∞) 1 (110) Plane (100) Plane -1 -1 0 y 1 1 ed Micr oSensin g& x at Sy gr ste Wi r e l e s s I n t e ms Introduction to MEMS ã Copyright: Khalil Najafi, Do not duplicate without written permission of authors Si Etching, page 31 Angles Between (100) and (110)/(111) Planes a a tan (a ) = Þ a = 54.7° a 2 a 2 a Four of the {111} planes make a 90° angle with the (110) planes, and four make the following angle b: b a 2 tan (b ) = 2 Þ b = 35.26° a oSensin Micr g& ed at Sy gr ste Wi r e l e s s I n t e ms Introduction to MEMS ã Copyright: Khalil Najafi, Do not duplicate without written permission of authors Si Etching, page 32 Angles Between (110) and (100) Planes a This is of course straightforward. Some of these planes make a 90° angle, and others make a 45° angle. No calculation is necessary. oSensin Micr g& ed at Sy gr ste Wi r e l e s s I n t e ms Introduction to MEMS ã Copyright: Khalil Najafi, Do not duplicate without written permission of authors Si Etching, page 33 Si Wafer Marking and Designation Standard designations have been developed by creating “flats” on Si wafers to represent their doping type (n- or p-), and wafer orientation. There are two types of flats: major flat (typically at the bottom of the wafer, and the minor flat, which may be present on the side of the wafer, as illustrated below. oSensin Micr g& ed at Sy gr ste Wi r e l e s s I n t e ms Introduction to MEMS ã Copyright: Khalil Najafi, Do not duplicate without written permission of authors Si Etching, page 34 Si Wafer Marking and Designation oSensin Micr g& ed at Sy gr ste Wi r e l e s s I n t e ms Introduction to MEMS ã Copyright: Khalil Najafi, Do not duplicate without written permission of authors Si Etching, page 35 Si Wafer Relation between wafer flat and different crystal planes for (100) Si wafer. The wafer flat aligns with one of the directions, in this example the flat is shown; it is perpendicular to the (110) plane as shown. flat is ^ to (110) plane at ed Micr oSensin g& From Fundamentals of Microfabrication by M. Madou ) Sy gr ste Wi r e l e s s I n t e ms Introduction to MEMS ã Copyright: Khalil Najafi, Do not duplicate without written permission of authors Si Etching, page 36 Example of (100) Si Wafer with a Direction Relation between wafer flat and (100) Si wafer surface: (110) Plane (110) Plane Direction (100) Si Wafer (100) Plane tion rec ] Di [110 Direction oSensin Micr g& ed at Sy gr ste Wi r e l e s s I n t e ms Introduction to MEMS ã Copyright: Khalil Najafi, Do not duplicate without written permission of authors Si Etching, page 37 Example of {110} and {111} Planes Direction Relation between some {110} and {111} planes (110) Plane (100) Si Wafer (100) Plane tion rec ] Di [110 (111) Plane oSensin Micr g& ed at Sy gr ste Wi r e l e s s I n t e ms Introduction to MEMS ã Copyright: Khalil Najafi, Do not duplicate without written permission of authors Si Etching, page 38 Si Wafer Primary Crystallographic Planes Relation between wafer flat and different crystal planes for a (100) Si wafer: {100} Plane {110} Plane > 10 {100} 10 (100) 1020 cm-3 1.4 400 Etches oxide Si3N4 (Water, Isopropyl Alcohol) not very fast Ethylene Diamine B>5x1019 cm-3 1.3 40 Saturates Si3N4, SiO2 Pyrocatechol (EDP), 115°C w/ silicates Au Tetramethyl Ammonium B>2x1020 cm-3 1 12-50 IC compatible Si3N4 Hydroxide (TMAH), 90°C easy to use SiO2 N2H4 (water), isopropyl B>1.5x1020 cm-3 3.0 10 Toxic Si3N4 alcohol, 115°C Explosive SiO2 (Hydrazine) metal films oSensin Micr g& ed at Sy gr ste Wi r e l e s s I n t e ms Introduction to MEMS ã Copyright: Khalil Najafi, Do not duplicate without written permission of authors Si Etching, page 53 Etch Rates of Si Along Different Directions (In EDP) After Seidel, 1990 oSensin Micr g& ed at Sy gr ste Wi r e l e s s I n t e ms Introduction to MEMS ã Copyright: Khalil Najafi, Do not duplicate without written permission of authors Si Etching, page 54 Etch Rates of Si Along Different Directions (In KOH) oSensin Micr g& ed at Sy gr ste Wi r e l e s s I n t e ms Introduction to MEMS ã Copyright: Khalil Najafi, Do not duplicate without written permission of authors Si Etching, page 55 ASE Etching Using A Mask, (100) Wafer thickness 54.7° Bottom of etch pit If etch stops early, the wafer will Etch reaches bottom of wafer at ed Micr oSensin g& have tapered sidewalls. when the mask opening is wide Sy gr ste Wi r e l e s s I n t e ms Introduction to MEMS ã Copyright: Khalil Najafi, Do not duplicate without written permission of authors Si Etching, page 56 ASE Etched Silicon (111) Plane (100) surface Etched Surface Transmitted light Top view of a piezo-resistive pressure Scanning Electron Micrograph (SEM) sensor. Diaphragm formed using ASE. of an etched pit, showing the (111) and Note that the etched surface is not (100) planes. perfectly polished. oSensin Micr g& ed at Photos courtesy of Prof. Ken Wise, University of Michigan Sy gr ste Wi r e l e s s I n t e ms Introduction to MEMS ã Copyright: Khalil Najafi, Do not duplicate without written permission of authors Si Etching, page 57 Si Wafer Etched in EDP and Stopped on Diaphragm Back View Bulk > 10 > 10 10 fe 10 i wa < 1 Silicon }S 00 {1 Mask layer A > > 10 10 2x1020 cm-3 1 12-50 IC compatible Si3N4 Hydroxide (TMAH), 90°C easy to use SiO2 oSensin Micr g& ed at Sy gr ste Wi r e l e s s I n t e ms Introduction to MEMS ã Copyright: Khalil Najafi, Do not duplicate without written permission of authors Si Etching, page 67 Concentration Dependence Etch Rate of Si in KOH & EDP Heavily Boron Doped (p++) p/p++ Selectivity as High as 0.1-0.001 EDP More Selective Than KOH Excellent Etch Stop at ed Micr oSensin g& After H. Seidel Sy gr ste Wi r e l e s s I n t e ms Introduction to MEMS ã Copyright: Khalil Najafi, Do not duplicate without written permission of authors Si Etching, page 68 Forming Highly Boron-Doped Regions In Silicon High-concentration boron-doped layers can be formed in silicon using several techniques: High-temperature diffusion using solid/gaseous sources High-temperature diffusion using spin-on glass (SOG) sources Epitaxial growth Low-temperature ion implantation & annealing Most common techniques involves the use of solid boron oxide or boron nitride sources. Typical pre-deposition is performed at 1100-1175°C for times between 1-15 hours. This process is performed in nitrogen with a 2-5% of oxygen flow. This will prevent reaction of boron with silicon and the creation of pits on the surface; Concentrations of 2x1020cm-3 at depths of 10-15 µm can be achieved. This high concentration is sufficient to achieve an excellent etch stop; Spin-on sources are also used, and provide A less costly alternative to solid sources. They, however, are not easily amenable to the formation of deep high-doped regions; Epitaxial growth of high-doped regions on silicon is also very powerful and can be used to form layers several microns in thickness. Often these layers are counter-doped with some germanium to reduce the high intrinsic stress in the resulting highly-doped material; Ion implantation is very attractive because of its low temperature nature, but can only be used to form shallow regions; oSensin Micr g& ed at Sy gr ste Wi r e l e s s I n t e ms Introduction to MEMS ã Copyright: Khalil Najafi, Do not duplicate without written permission of authors Si Etching, page 69 Diffused Boron Concentration Profile In Silicon Profile and diffusion depth are very reproducible Concentration profile non-uniform through layer thickness This causes the internal stress to be non-uniform through thickness This non-uniform internal stress can be somewhat annealed before release Non-uniformity causes problems in cantilever beams, not in doubly-supported structures Depth p++ Lightly-Doped Si at ed Micr oSensin g& (µm) Sy gr ste Wi r e l e s s I n t e ms Introduction to MEMS ã Copyright: Khalil Najafi, Do not duplicate without written permission of authors Si Etching, page 70 Application of Boron Etch-Stop to Fabrication of Silicon Microprobes Si doped to a depth of 15µm Rest of probes fabricated on top of the doped regions The entire wafer is then dissolved away in EDP Only the p++ and other protected areas remain 1) Grow and Pattern Thermal Oxide, Deep Boron Diffuse to Form Substrate OUTPUT LEADS 2) Deposit Bottom Dielectric Films, Deposit and Pattern Interconnect Deposit Top Dielectric Films 3) Pattern and Etch Top Dielectric Films, to Define Recording Sites, Deposit and Liftoff Site Material 4) Etch Field Dielectric Films Outside of dM i c roS ens i ng Probe Areas, Etch and Free Probes in e & at Silicon (EDP) Etchant Ken Wise & Khalil Najafi, University of Michigan Sy gr ste Wi r e l e s s I n t e ms Introduction to MEMS ã Copyright: Khalil Najafi, Do not duplicate without written permission of authors Si Etching, page 71 Examples Of Micromachined Silicon Probes Sharp Probe Tip Recording/Stimulating Sites 2mm oSensin Micr g& ed at Prof. Khalil Najafi, University of Michigan Sy gr ste Wi r e l e s s I n t e ms Introduction to MEMS ã Copyright: Khalil Najafi, Do not duplicate without written permission of authors Si Etching, page 72 Boron Etch-Stop Used to Fabricate an IR Detector Boron-Doped 15µm-Thick p++ Silicon Rim Region Gold-Polysilicon Thermocouples Dielectric Diaphragm High Responsivity, Detectivity Volume Production Detectors Designed To Customer Specs 3.5mm oSensin Micr g& ed at Prof. Khalil Najafi, University of Michigan Sy gr ste Wi r e l e s s I n t e ms Introduction to MEMS ã Copyright: Khalil Najafi, Do not duplicate without written permission of authors Si Etching, page 73 Hemispherical Shells Fabricated Using ISE Fabricate hemispherical shells Use boron doping and p++ etch stop (described later) Release shells in concentration-dependent etchant Si substrate Etch Si using HNA and a nitride mask Boron Doped Boron dope shells to desired depth Released Shells Released Si Shells Release shells in EDP/KOH ~2µm in thickness oSensin Photos Courtesy of Prof. Ken Wise Micr g& ed at University of Michigan Sy gr ste Wi r e l e s s I n t e ms Introduction to MEMS ã Copyright: Khalil Najafi, Do not duplicate without written permission of authors Si Etching, page 74 Lightly-Doped Si As An Etch Stop It is also possible to achieve a doped etch-stop on the lightly-doped side of a pn junction. The HF+Nitric+Acetic (1:3:8) etch, etches highly-doped regions doped to a concentration > ~1017cm-3 much faster than the undoped regions. This etch is not used very often because it is difficult to mask against the HNA etch, and also because it is not easy to form a lightly-doped region in an already highly-doped region (note that we cannot diffuse into a highly-doped region and create a region with a lower concentration.) The 1:3:8 HNA etch is sometimes used to remove layers of highly-doped Si and stop on lightly-doped silicon. Heavily-doped Buried layer N+ doped Lightly-doped N+ region etched away Etch heavily-doped In 1:3:8 HNA oSensin Micr Etch lightly-doped Si in EDP/KOH g& ed at Sy gr ste Wi r e l e s s I n t e ms Introduction to MEMS ã Copyright: Khalil Najafi, Do not duplicate without written permission of authors Si Etching, page 75 Dielectric Etch Stop Silicon-On-Insulator (SOI) wafers are becoming and abundant because of their use in IC manufacturing. The Si on the insulator (which is usually a silicon dioxide) can be either single-crystal, or polycrystalline The silicon oxide acts as an excellent etch stop because it does not etch appreciably in etchant like KOH or EDP. The thickness of the silicon on top of the insulator can be controlled very accurately with better than 5% uniformity. SOI wafers are typically much more expensive than standard Si wafers. Polycrystalline Si Single-Crystal Si (SOI) at ed Micr oSensin g& Dielectric Dielectric Sy gr ste Wi r e l e s s I n t e ms Introduction to MEMS ã Copyright: Khalil Najafi, Do not duplicate without written permission of authors Si Etching, page 76 Si Etching (Micromachining) Wet Etching – Isotropic – Anisotropic (orientation-dependent) – Concentration-dependent Heavily-doped etch stops Lightly-doped etch stops – Electrochemical Etch Stop pn junction – Dielectric Etch Stop Dry Etching – Plasma etching at ed Micr oSensin g& – Deep Reactive Ion Etching (DRIE) Sy gr ste Wi r e l e s s I n t e ms Introduction to MEMS ã Copyright: Khalil Najafi, Do not duplicate without written permission of authors Si Etching, page 77 Plasma Etching of Si Standard plasma etching can be used to etch Si. Of course this etch is