Summary Lectures MEMS, NEMS & Nanofabrication PDF
Document Details
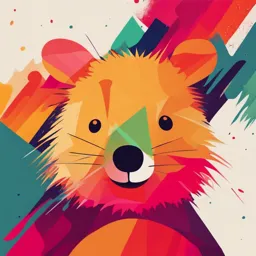
Uploaded by EffectualAmbiguity9570
Gaurav
Tags
Related
- Fabrication Techniques for MEMS-Based Sensors PDF
- Fabrication Techniques for MEMS-Based Sensors: Clinical Perspective PDF
- Fabrication Techniques for MEMS-based Sensors (PDF)
- Silicon Etching - 2024 (PDF)
- MEMS Sensors and Transducers Part 1 PDF
- Microsystems, MEMS Applications, Manufacturing Methods for MEMS PDF
Summary
This document provides a summary of lectures on MEMS, NEMS, and nanofabrication, focusing on thin film deposition techniques. It covers topics including PVD, thermal evaporation, sputtering, and magnetron sputtering. The document also discusses applications of these techniques and methods of fabrication. It also discusses properties of different elements, like silicon.
Full Transcript
Summary Lectures MEMS, NEMS and Nanofabrication Lecture 1: Gaurav_ 1 MEMS (thin film deposition) Approaches - Top down: Start with large material or substrate, where smaller fragments are created, into nano particles. o Imperfection of the surface structure....
Summary Lectures MEMS, NEMS and Nanofabrication Lecture 1: Gaurav_ 1 MEMS (thin film deposition) Approaches - Top down: Start with large material or substrate, where smaller fragments are created, into nano particles. o Imperfection of the surface structure. Lithography can cause crystallographic damage to the patterns. Surface imperfection may lead to change in properties - Bottom up: builds nanostructures atom by atom or molecule by molecule, often through chemical or physical processes o Less defects, more homogeneous chemical composition and better range ordering. PVD: Physical Vapour Deposition (top-down) - Evaporation, sputtering, molecular beam epitaxy Thermal evaporation: the simplest forms of PVD and typically uses a resistive heat source to evaporate a solid material in a vacuum environment to form a thin film. - High deposition rate, low cost, no solvent, suitable for low melting point materials, easy to monitor the deposition rate and thickness film - Not suitable for multicomponent thin film production E-Beam Evaporation: It relies on a high-energy electron beam to heat and vaporize a material in a vacuum chamber Components: Electro gun, beam deflection magnetic lens, Evaporant containing hearth - Energy is supplied on top of evaporant - The e-beam melts relatively small area - This small area is heated above melting point, while the temperature of source material is much lower (cooled) Ion plating: The substrate or growing film experiences a continuous or periodic flux of energetic species that influences the deposition process and resulting film properties. (plasma or vacuum based) Sputtering: Ejection of particles (atoms, ions, clusters) from a surface which is bombarded by energetic ions. - Caused by collision cascades taking place inside the solid, resulting in a flow of target atoms. - Atoms which energies exceeding surface binding energy will then be ejected. o Chemical: Reactions between incoming beam particles and target material o Physical: Energetic particles penetrate a certain distance into the solid. o Plasma: Ionisation of gas can be achieved by electric field or high temperature heating. Sputtering Yield (S): Number sputtered ions per incoming particle The sputtering yield S(E) for an energetic ion having the energy E exceeding 1 KeV can be written as: - S(E) = ¾ π2 * 1/C0U0 * α * Sn(E) o Co: Interaction potential o Uo: Surface potential barrier o α : Efficiency momentum transfer o Sn(E): Elastic stopping power For lower impinging energies, up to about 1 KeV, the sputtering yield is given by: - S(E) = 3/4π2 * α * Tm/Uo o Tm = 4M1M2/(M1 +M2) * E ▪ M1 and M2 are the masses of the incoming ion and the target, respectively Variation of the sputtering yield with the angle of ion incidence θ to the target surface normal: 𝑆(𝐸,ℎ) - 𝑆(𝐸,1) = (𝑐𝑜𝑠ɵ)-f o h: direction cosine, S(E,1): sputtering yield for normal ion incidence, and f a number which lies between 1 and 2. Sputtering - The high-energy inert gas ions are used to deposit the nanomaterials on the surface of the substrate ejected from the target material Advantages: No high temperature, uniform coating on large sample, wide range of targets Disadvantages: Low sputtering rate, heat Magnetic fields are used to increase the number of sputtered material. (Negative potential UT, gives rise to electric field) To initiate magnetron sputtering: 1. Voltage Application: A high DC voltage (1,000–2,000 V) is applied to the target to ignite the discharge, then reduced to 400–800 V for continuous operation. RF or HF potentials can also be used. 2. Gas Introduction: Argon gas is introduced into the vacuum chamber using a gas regulation system. 3. Plasma Formation: High voltage ionizes the argon gas, creating a plasma with separated ions and electrons. 4. Electron Dynamics: Electrons are influenced by electric (E) and magnetic (B) fields, moving in helical paths due to these forces, which sustain the plasma. Balanced magnetron sputtering: When magnets mounted on the back side of the target are all of equal strength Unbalanced magnetron sputtering: Central magnet is weaker than the outer magnets leading to change in the magnetic field. (enhancing the rate of sputtered ions) Reactive sputtering: Uses reactive gas (oxygen, nitrogen) in addition to the argon Things that influence magnetron sputtering: Temperature, ion bombardment, vacuum and stress. Applications sputtering: Industrial application, optical and photovoltaic coatings and in medical applications. Pulsed Laster Deposition (PLD) - Composed of a vacuum chamber (target and substrate holder) - These components are separated by a predefined gap - Laser is used to ablate the material of the target which creates a plasma that expands towards the substrate: Plasma plume. o Lasers: Excimers, Solid-state lasers, YAG Vacuum chamber: Vacuum is achieved by a series of turbo molecular and rough pumps. (10-6 mbar, 10-9 mbar) - Time that single surface layer of contaminants and impurities can form on the substrate 4 surface: 𝑡 = 𝑛𝑣𝑑2 2.46 * 10−6 - In air the eqn. can be simplified to: 𝑡 = 𝑃 Mean free path: Distance molecule travels before it collides with another molecule. - Environmental pressure influences it Target Holders - Rod-shaped: Adv: Mechanical simplicity, Dis: Poor sintering properties - Disk-Shaped Laser ablation: Uses high intensity laser pulses to fabricate nanocrystals for thin films - Properties can be controlled by laser and liquid medium - Ablation: Process associated with both evaporation and melting Molecular Beam epitaxy (MBE) Epitaxy: Process of growing a crystal of a particular orientation is determined by the underlying crystal. Lecture 2: Gaurav_2 MEMS (Thin film deposition) Pulsed Laster Deposition (PLD) Electrical pumping: Applied electrical field - An electron excited to a higher energy state (E1 to E2) releases energy as photons during relaxation. - Spontaneous emission: A heterogenous process where electrons relax randomly, emitting incoherent photons. - Stimulated emission: A homogenous process where electrons relax together, emitting coherent photons with identical phase, amplitude, and frequency. - Photons undergo amplification through a set of mirrors, thereby directing the photons back and forth within the cavity. Finally, the photons are released from the cavity via a semi-transparent window and focused into an aperture (opening). Key proccesses in PLD: - Laser ablation - Plasma formation and expansion of target material - Subsequent nucleation and growth target material on substrate Laser ablation Two types of interactions: Non-thermal interactions first stage, incident photons provide enough energy to induce band transitions as the target material absorbs light - Electron undergoes intraband transition from the ground state to excited state, and relaxes - Energy of two photons combined to promote the molecule into an excited state: multiphoton excitation 𝐼 - Beer-Lambert Law (penetration depth): 𝐴 = 𝐼0 = ∈𝑐𝑡 o A is the absorbance, I the light intensity, I0 the incident light intensity, e the molar extinction coefficient, c the molar concentration and t the thickness Thermal excitation: rapid heating in a localised area (>1000 Kelvin) - The vibrational relaxation of excited electrons tranfer heat to the system - Surface heat propagates into the bulk of the target, which can cause unintented side effects 1 - ( Thermal diffusion length: 𝐿𝑇 = 2𝐷τ𝐿 ) 2 o D is the thermal diffusivity and τ𝐿 is the laser pulse length. - The material is ejected from the target by the combination of non-thermal (electronic) and thermal ejection mechanism Plasma formation and expansion Mechanisms promoting generation of free electrons, and increase degree of ionisation of the plasma: - Photoionation: Electron ejection from atom following photon absorption - Inverse Bremsstrahlung: Electron gains kinetic energy by collisional energy tranfers with other particles Plasma formation and early stage expansion: Electrons and light elements lead the plasma plume expansion. Electrostatic gradient forms an electrostatic double layer. Electrons split into two types: energetic electrons (front of the plume) and cold electrons (back). The electric field gradient accelerates the ions inside the double layer Film nucleation and growth Surface diffusion and nucleation - When plasma species reach the substrate, they can physisorb and chemisorb, with the adsorption mode depending on surface coverage: low coverage leads to tracer-like diffusion, while high coverage favors Coulomb interactions and chemical diffusion. - On uniform surfaces, atoms diffuse intrinsically, while defects cause mass transport diffusion; the adatoms move via hopping, tunneling, atomic exchange, or vacancy diffusion Coalescence (samensmelting) - Driving force of these mechanisms is the reduction of Gibbs free energy (shring surface area) - Frank: Interactions between atoms and surface is stronger than between atoms - Stranski: Films starts growing in layer by layer, because of external forces the growth is disturbed - Volmer: High surface tension resulting from high atom-atom interactions compared to atom-surface causes the film to grown in island like formations Molecular beam epitaxy (MBE) (Picture see lecture 1) - Analysis chamber: analytical techniques fitted in - Preparation chamber: In-situ processes: etching, metallisations, insulator depositions. Growth chamber: controlled environment for the growth or deposition of materials - Cells to produce molecular beams - Shutters to switch molecular beams on/off - Heatable substrate holder - Loadlock system allows for wafers into growth chamber - Cryopanels to remove contaminats (CO, CO2, H20) and thermal insulation Sources of molecular beam Thermal-evaporation cells (TEC) - Crucibles (smeltkroezen) are heated by TA ribbons - Thermal isolation by radian shields made of TA foils. Valved cracker cells (VCC) - TEC hard to use with large capacity, so VCC used - VCC can generate molecular beams with fast time reponse - As cells have cracking stages, evaporated As tetrameters are converted into dimers - Temperature cracking stage determines amount as4 and as2 - Dimers are more advantageous favourable surface kinetics Physical chemistry of solid surfaces: Increase in the ratio of surface atoms to interior atoms in nanostructures and nanomaterials are responsible for great changes in the physical and chemical properties. - Surface area increases while total volume remains constant Surface energy: The extra energy possessed by the surface atoms - Surface Atoms: Fewer neighbors (dangling bonds) create inward forces, shortening bond distances and increasing surface energy. - Small Particles: Reduced lattice constants due to significant bond shortening at the surface. 3 ways of Surface Energy Reduction: Surface relaxation: Inward shifts of surface atoms, more prominent in liquids. Surface restructuring: Formation of new strained bonds. Surface adsorption: Chemical or physical adsorption of species via bonding or weak forces. Composition segregation: Surface enrichment of impurities through diffusion. - Combining Nanostructures: Merging small structures into larger ones to reduce surface area, requiring sufficient activation energy. Includes sintering (structures merge) and Ostwald ripening (larger structures grow by consuming smaller ones). - Agglomeration: Clustering of individual nanostructures without changing their individual form. Chemical Vapour Deposition (CVD): process in which a carrier gas or diffusion transports a precursor to a reaction zone where the precursor either react with gases or decompose while it is in the gas phase (homo), or it can adsorb on the surface and react with gases or decompose (hetero). (semiconductor, dielectric, conducting or magnetic materials), under high temperature - Chemisorption: Chemical binding of a molecule to a surface by electron transfer - Physisorption: Physical binding of molecule to surface by electrostatic attraction or Van der Waals forces - Planar technology: Process allows the fabrication of flat and smooth surfaces - Precursor: Compound that can be vaporized and transported to the substrate - Substrate: Material surface upon which a thin film is deposited PVD CVD High quality and thin films Can result in contamination, complex chemical reaction Low deposition rate High deposition rate Expensive Cheap CVD system - Carrier gas and gas manifold system, controlled flow of gases - Gas delivery system (vaporizer), provides precursos in geaseous state - Gas distribution into the reaction chamber control gas ratio - Energy sources provides energy for deposition reaction - Exhaust gas cleaning system that destroys volatiles Precursor and vaporizer Requirements: Volatile, nontoxic, easy to handle, chemically and thermally stable, cost-effective and clean decomposition Volatility: Enables the precursor to vaporize and transport to the substrate in a gas flow. Stability in Gas Phase: Prevents premature decomposition before reaching the substrate. Controlled Decomposition: At the heated substrate, the precursor decomposes or reacts to form the thin film. Organic Ligands: Must produce volatile, thermally stable byproducts to avoid contaminating the thin film. Reactor is designed to control film thickness, crystal structure, surface morphology, film composition. CVD Variants: Horizontal & Vertical CVD: o Horizontal: Common configuration; substrates mounted at different angles. o Vertical: Equipped with a showerhead mixer for uniformity and growth rate. Low-pressure & Atmospheric-pressure CVD: o Low-pressure: Uses a vacuum pump for gas flow. o Atmospheric-pressure: No pump required; slower gas flow. Hot-wall & Cold-wall CVD: o Hot-wall: Entire chamber uniformly heated. o Cold-wall: Heats only the substrate area for rapid heating/cooling. Plasma, Photo-assisted & Laser-assisted CVD: o Plasma-enhanced: Uses ionized gas (plasma) to reduce reaction temperature. o Photo/Laser-assisted: High-intensity light or laser promotes deposition. Metal–organic CVD (MOCVD): o Uses volatile metal-organic precursors to form thin films. o Common for synthesizing III–V compound semiconductors (e.g., optoelectronics). Hot Filament/Wire CVD: o Uses resistively heated filaments to decompose precursors for deposition (tungsten, tantalum, molybdenum) o Deposits inorganic films (e.g., amorphous silicon, silicon nitride). Initiated CVD: o Variation of hot filament CVD for polymer thin films. o Uses initiators and monomers as vapor-phase reactants for chain-growth polymerization. o Enables lower filament temperatures to preserve organic functional groups. Oxidative CVD: o Uses oxidants and monomer vapors that spontaneously react on the substrate. o Produces conducting and semiconducting polymer films via step-growth polymerization. Materials Fabricated by CVD - Group III/V, IV, IIB/VI semiconductor material Lecture 3: Crystal structures and fundamentals Silicon strenghts - Good mechanical material - Thermal conductor - Transparant in infrared - Semiconductor - Optically smooth and flat - Known inside out Types of silicon materials Crystalline (single crystal) Polycrystalline Amorphous (non-crystalline) All atoms arranged in pattern. Regions of single crystalline Random arrangement of Quartz (SiO2) silicon seperated by grain atoms. The periodicity of atoms can be boundaries with irregular Glass described by a network of bonds points in space: Lattice Crystalline Solid: Is the solid form a substance in which the atoms or molecules are arranged in a definite, repeating pattern in three dimensions. - Single crystals, ideally have a high degree of order, or regular geometric periodicity, throughout the entire volume of the material. (diffraction pattern) Polycrystalline solids: Agregate of many small single crystals. High degree of order over many atomic or molecular dimensions. - These ordered regions, cary in size and orientation with respect to another: Grains o Seperated by grain boundaries - Atomic order can vary form one domain to the next. o Grain are usually 100 nm – 10 microns in diameter..1 Phosphorous (P) Wafer flats – Purpose and function - Orientation for automatic equipment - Indicate type and orientation of crystal Primary Flat: The flat of longest length located in the circumference of the wafer. The primary flat has a specified crystal orientation relative to the wafer surface; major flat. Secondary Flat: Indicates the crystal orientation and doping of the wafer. The location of this flat varies. Demands Silicon Wafers - Each wafer must be fabricated with a specific orientation, resistivity and dopant type. - Defects in the wafer must be below tolerance - Impurity concentration must be below permissible level (99.99999% pure, so 7 nines) - Thickness must be within tolerance (±25μm or less) - (1,0,0) or desired wafer orientation parallel to the surface within 2 degrees. - Dopants and resistivity within tolerance (