Biochemistry Illustrated Notebook (2015/2016) PDF
Document Details
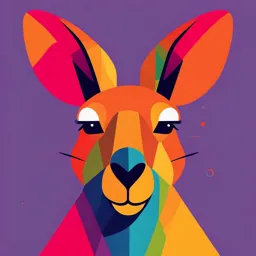
Uploaded by SleekHeliodor
Beni-Suef University
2016
Dr. Reem Samy Hashem
Tags
Summary
This illustrated notebook covers the topic of carbohydrate metabolism in biochemistry. It includes classifications of carbohydrates, their properties, and their roles in various metabolic pathways. The notebook is from Beni-Suef University and was published in 2016.
Full Transcript
Biochemistry Department Faculty of Pharmacy Beni-Suef University Illustrated Notebook of Biochemistry METABOLISM BY Dr. Reem Samy Hashem Assistant Professor of Biochemistry Head Department 2015/2016 ...
Biochemistry Department Faculty of Pharmacy Beni-Suef University Illustrated Notebook of Biochemistry METABOLISM BY Dr. Reem Samy Hashem Assistant Professor of Biochemistry Head Department 2015/2016 Carbohydrate The chemistry of carbohydrates Carbohydrates are the most abundant organic molecules in nature. The empiric formula for simple sugars, or monosaccharides is (CH2O)n, hence the name ―hydrate of carbon.‖ Classification: 1- Monosaccharides are those sugars that cannot be hydrolyzed into simpler carbohydrates. They may be classified as trioses, tetroses, pentoses, hexoses, or heptoses, depending upon the number of carbon atoms, and as aldoses or ketoses, depending upon whether they have an aldehyde or ketone group EX: glucose and fructose. 2. Disaccharides are condensation products of 2 monosaccharide units; examples are maltose and sucrose. 3. Oligosaccharides are condensation products of 3-10 monosaccharides. Most are not digested by human enzymes. 4. Polysaccharides are condensation products of more than 10 monosaccharide units; examples are the starches, dextrins, inulin (a fructose polymer) and cellulose.. Derivatives of the carbohydrates can contain nitrogens, phosphates and sulfur compounds. Carbohydrates also can combine with lipid to form glycolipids or with protein to form glycoproteins. The suffix – ose is used for naming Contain at least 3 carbon atoms Nomenclature The predominant carbohydrates encountered in the body are structurally related to the aldotriose glyceraldehyde and to the ketotriose dihydroxyacetone. All carbohydrates contain at least one asymmetrical (chiral) carbon and are, therefore, optically active. carbohydrates can exist in either of two conformations, as determined by the orientation of the hydroxyl group about the asymmetric carbon farthest from the carbonyl. carbohydrates that are of physiological significance exist in the D-conformation. The mirror-image conformations (L and D forms of a sugar) are called enantiomers. 1 O H O H C C H – C – OH HO – C – H HO – C – H H – C – OH H – C – OH HO – C – H H – C – OH HO – C – H CH2OH CH2OH D-glucose L-glucose Monosaccharides The monosaccharides commonly found in humans are classified according to the number of carbons they contain in their backbone structures. The major monosaccharides contain four to six carbon atoms. Carbohydrate Classifications # Carbons Category Name Relevant examples 3 Triose Glyceraldehyde, Dihydroxyacetone 4 Tetrose Erythrose 5 Pentose Ribose, Ribulose, Xylulose 6 Hexose Glucose, Galactose, Mannose, Fructose 7 Heptose Sedoheptulose 9 Nonose Neuraminic acid, also called sialic acid Cyclization of aldoses and ketoses Intermolecular cyclization of D-Glucose → produces a new chiral center →5 ( not 4 ) asymmetric carbons The 6-membered ring of a monosaccharide →pyranose 5-membered ring →furanose Unlike pyran and furan, the rings of CHets do not contain double bonds 2 Fischer projection of Haworth projection of Chair form α-D-Glucose α-D-Glucose Cyclization of glucose produces a new asymmetric center at C1. The 2 stereoisomers are called anomers, α & β. Haworth projections represent the cyclic sugars as having essentially planar rings, with the OH at the anomeric C1: 1.α (OH below the ring) 2.β (OH above the ring). Conformation of monosaccharides Cyclic sugars→3 dimensional shapes rings furanose →envelope or twist Pyranose → chair or boat The chair conformations minimize steric repulsion 3 Epimers: Isomers differing as a result of variations in configuration of the —OH and —H on one of the asymmetric carbon atoms. Biologically, the most important epimers of glucose are mannose (epimerized at carbon 2) and galactose (epimerized at carbon 4). 4 Derivatives monosacchardies Sugar Phosphates Monosaccharides in metabolic pathways are often converted to phosphate esters. Deoxy Sugars A hydrogen atom replaces one of the hydroxyl groups in the parent monosaccharide. 2-Deoxy- D-ribose is an important building block for DNA Amino Sugars An amino group replaces one of the hydroxyl groups in the parent monosaccharide sometimes the amino group is acetylated. Sugar alcohols The carbonyl oxygen of the parent monosaccharide has been reduced, producing a polyhydroxy alcohol. e.g. Glucose or fructose sorbitol Galactose galactitol Sugar acids Sugar acids are carboxlic acids derived from aldoses oxidation of C-1 → aldonic acid oxidation of highest numbered carbon → alduronic acid. Ascorbic acid is vitamin C 5 6 ascorbic acid Disaccharide and other glycosides The anomeric hydroxyl and a hydroxyl of another sugar or some other compound can join together, splitting out water to form a glycosidic bond: R-OH + HO-R' R-O-R' + H2O Sugars Form Glycosides with Other Compounds & with Each Other If the second group is a hydroxyl O-glycosidic bond e.g. All sugar-sugar glycosidic bond If the second group is an amine N-glycosidic bond Maltose is a disaccharide released during the hydrolysis of the starch maltose is of 2 D-glucose residues joined by an α (1- 4) glycosidic bond , 7 Cellobiose , a product of cellulose breakdown, is the β(1→4)glycosidic bond Lactose, the major CHets in milk is synthesized only in lactating mammary glands Sucrose, common table sugar, has a glycosidic bond linking the anomeric hydroxyls of glucose & fructose. Because the configuration at the anomeric C of glucose is α (O points down from ring), the linkage is α (12). The full name of sucrose is α-D-glucopyranosyl-(12)-β-D-fructopyranose.) 8 Reducing and non-reducing sugars Glucose, maltose, cellobiose, and lactose, are sometimes called reducing sugars. Sucrose is not readily oxidized because both anomeric carbon atoms are fixed in a glycosidic linkage → nonreducing sugars Polysaccharide Homoglycans (homopolysaccharides) containing residues of only one type of monosaccharide. Heteroglycans (heteropolysaccharide) are polymers containing residues of more than one type of monosaccharide Homoglycans Starch and glycogen D-glucose is synthesized in all species excess glucose can be broken down to produce metabolic energy glucose residues are stored as polysaccharides until they are needed for energy production as starch in plants and glycogen in animals , Starch is a mixture of amylose and amylopectin amylose is an unbranched polymer of D-glucose residues It is connected by α – ( 1 to 4 ) glycosidic linkages Amylopectin is a branched version of amylase branches It is attached via α- (1 to 6) glucosidic bonds. Glycogen contains the same types of linkages found in starch Branches are smaller and more frequent in mammals, glycogen can account for up to 10% of the mass of the liver and 2% of the mass of muscle. Cellulose is a structural polysaccharide , major components of the rigid cell walls that surround many plant cells 9 Glycoconjugates consist of polysaccharides linked to proteins or peptides (heteroglycans) : Proteoglycans are complexes of proteins and Polysaccharides called glycosaminoglycans. glycosaminoglycans composed of a repeating disaccharide unit [acidic sugar–amino sugar]n Hyaluronic acid is an EX. of a glycosaminoglycan it is found in the fluid of joints it is also a major component of cartilage. Peptidoglycans are polysaccharides linked to small peptides in the cell walls of many bacteria. Glycorproteins (mucoproteins) are proteins that contain oligosaccharide and sialic acid Ex.: Enzymes , hormones, structural proteins, and transport proteins 10 Structure and distribution of glycosaminoglycans are shown in following figure: 11 INTRODUCTION TO METABOLISM Most pathways can be classified as either catabolic (degradative) or anabolic (synthetic). Catabolic reactions Anabolic pathways break down complex molecules to a few combine small molecules as amino acids simple molecules (CO2, NH3 and H2O) form complex one such as protein Exergonic and Librate (ATP, NADH, Endergonic and require ATP and Pi FADH2) Typically oxidative Mostly reductive require coenzymes such as NAD+ or FAD Coenzymes NADPH Catabolic hormones like Gglucagon, Anabolic hormones like insulin adrenaline Figure 1: Three stages of catabolism 12 REGULATION OF METABOLISM The pathways of metabolism must be coordinated so that the production of energy or the synthesis of end products meets the needs of the cell. Regulatory signals that inform an individual cell of the metabolic state of the body as a whole include hormones, neurotransmitters, and the availability of nutrients. A.Signals from within the cell (intracellular) The rate of a pathway may be influenced by the availability of substrates, product inhibition, or alterations in the levels of allosteric activators or inhibitors. This is important for the moment-to-moment regulation of metabolism. B.Communication between cells (intercellular) For energy metabolism, the most important route of communication is chemical signaling between cells, for example, by blood-borne hormones or by neurotransmitters. C.Second messenger systems Two of the most widely recognized second messenger systems are the calcium/phosphatidylinositol system and the adenylyl cyclase system, D.Adenylyl cyclase This is a membrane-bound enzyme that converts ATP to 3',5'-AMP (cAMP). Different peptide hormones can either stimulate or inhibit the production of cAMP from adenylyl cyclase 1.GTP-dependent regulatory proteins: Opposite figure illustrates the recognition of the signal that trigger cAMP formation These proteins, referred to as G-proteins because they bind guanosine nucleotides (GTP and GDP), form a link in the chain of communication between the receptor and adenylyl cyclase. The inactive form of a G-protein binds to GDP The activated receptor interacts with G-proteins, triggering an exchange of GTP for GDP. The trimeric G-protein then dissociates into an α subunit and a βγ dimer. The GTP-bound form of the α subunit moves from the receptor to adenylyl cyclase which is thereby activated. Rapid hydrolysis of GTP to GDP causes the inactivation of G- protein and in consequence adenylyl cyclase is inactivated (figure 2) Figure 2 cAMP is rapidly converted to 5'-AMP by cAMP phosphodiesterase, 13 The ability of a hormone or neurotransmitter to stimulate or inhibit adenylyl cyclase depends on the type of G-protein that is linked to the receptor. One family of G-proteins, designated Gs, is specific for stimulation of adenylyl cyclase; another family, designated Gi, causes inhibition of the enzyme 2. Protein Kinases: cAMP activates family of enzymes called cAMP-dependent protein kinases, for example, protein kinase A Cyclic AMP activates protein kinase A by binding to its two regulatory subunits, causing the release of active catalytic subunits. activated subunits catalyze the transfer of phosphate from ATP to specific serine or threonine residues of protein substrates. phosphorylated proteins may become activated or inhibited enzymes (fig 3) Not all protein kinases respond to cAMP like protein kinase C 3. Dephosphorylation of proteins: Figure 3 The phosphate groups added to proteins by protein kinases are removed by protein phosphatase This ensures that changes in enzymic activity induced by protein phosphorylation are not permanent. TRANSPORT OF GLUCOSE CELLS Glucose cannot diffuse directly into cells, but enters by one of two transport mechanisms: 1.Facilitated diffusion transport system 2.Na+ -monosaccharide co-transporter system. Facilitated diffusion transport system This system is mediated by a family of at least 14 glucose transporters in cell membranes. They are designated GLUT-1 to GLUT-14 GLUT-1 is abundant in erythrocytes Hepatic GLUT-2 for uptake of glucose, galactose, and fructose from blood Pancreatic GLUT2 molecules mediate an increase in glucose uptake which leads to increased insulin secretion. For this reason, GLUT2 is thought to be a "glucose sensor". GLUT-3 is the primary glucose transporter in neurons. 14 GLUT-4 is abundant in adipose tissue, heart and skeletal muscle. [Note: The number of GLUT-4 transporters active in these tissues is increased by insulin.] GLUT-5 is the only transporter that exclusively transports fructose. It is expressed in intestine, kidney, testes, skeletal muscle, adipose tissue and brain. Na+ -monosaccharide co-transporter system This is an energy-requiring process that transports glucose "against" a concentration gradient Glucose is coupled to the concentration gradient of Na+ which is transported into the cell at the same time. Occurs in the epithelial cells of the intestine, renal tubules Glucose and galactose transported into mucosal cells by sodium-dependent monosaccharide transporter 1 (SGLT-1) GLYCOLYSIS (Embden–Meyerhof pathway) The glycolytic pathway is employed by all tissues for the breakdown of glucose to provide energy (in the form of ATP) and intermediates for other metabolic pathways. Pyruvate is the end product of glycolysis in cells with mitochondria (aerobic glycolysis) because oxygen is required Anaerobic glycolysis is conversion of glucose to lactate to produce ATP in tissues that lack mitochondria (for example, red blood cells) or in cells deprived of sufficient oxygen Most tissues have at least a minimal requirement for glucose. In the brain, the requirement for glucose is substantial, in erythrocytes, it is nearly total It takes place in the cytoplasm 15 16 REACTIONS OF GLYCOLYSIS Reaction #1 α-D-Glucose + ATP → α-D-Glucose-6-Phosphate + ADP It is irreversible reaction ATP energy is used. Phosphorylation of glucose, efficiently trap it inside the cell, since phosphorylated intermediates don‘t readly pass through the cell membrane Four mammalian isozymes of hexokinase are known (Types I–IV), with the Type IV isozyme often referred to as glucokinase. The major differences between hexokinase and glucokinase are sumarized in the following table Hexokinase Glucokinase Tissue distribusion Most tissues Liver and β-cells Km Low (high affinity) High (low affinity) Vmax Low High Inhibition by G-6-P Yes No Substrate specifity On glucose, fructose and On glucose only galactose CHO diet on activity Not affected by fasting or high affected by fasting or CHO diet high CHO diet Insulin or diabetes Not influenced Induced by insulin Why phosphorylated intermediates? phosphorylation keeps the substrate in the cell. Glucose is a neutral molecule and could diffuse across the cell membrane, but phosphorylation confers a negative charge on glucose and the plasma membrane is essentially impermeable to glucose-6-phosphate and so, the phosphorylated intermediates cannot disperse out of the cell. Energy used in the formation of the phosphate ester is partially conserved. High energy phosphate compounds formed in glycolysis (1,3-bisphosphoglycerate and phosphoenolpyruvate) donate phosphoryl groups to ADP to form ATP. Reaction #2: α -D-glucose-6-phosphate D-fructose-6-phosphate It is reversabile reaction Phosphoglucoisomerase catalyzes this isomerization Reaction #3 : D-fructose-6-phosphate + ATP → D-fructose-1,6-bisphosphate It is irreversabile reaction ATP energy is used PFK-1 is considered to be the rate-limiting enzyme Reaction #4: D-fructose-1,6-bisphosphate Dihydroxyacetone phosphate + D- Glyceraldehyde-3- Phosphate It is reversabile reaction Aldolase catalyses the hydrolysis of F1,6BP into two 3-carbon products: dihydroxyacetone phosphate (DHAP) and glyceraldehyde 3-phosphate (G3P). Reaction #5: Dihydroxyacetone phosphate D-Glyceraldehyde-3-Phosphate It is reversabile reaction Triose Phosphate Isomerase catalyses the isomerization Reaction #6: D-Glyceraldehyde-3-Phosphate + NAD+ + Pi 1,3 bisphosphoglycerate + NADH + H+ 17 It is reversabile reaction In this reaction, G3P is phosphorylated and oxidized by glyceraldehyde-3-phosphate dehydrogenase (G-3-P DH) Phosphorylation occurs at the expense of inorganic phosphate (Pi) G-3-P DH can be inhibited by iodoacetate and heavy metals, such as mercury. Reaction #7: 1,3 bisphosphoglycerate + ADP D- 3-phosphoglycerate + ATP It is a reversabile reaction Phosphoglycerate kinase enzyme catalyzes substrate-level phosphorylation of ADP to produce 3- phosphoglycerate (3PG) and the first ATP of glycolysis. Associated with the phosphoglycerate kinase pathway is an important reaction of erythrocytes, the formation of 2,3-bisphosphoglycerate, 2,3BPG (see Figure below) by the enzyme bisphosphoglycerate mutase. 2,3BPG is an important regulator of hemoglobin's affinity for oxygen. Note that 2,3-bisphosphoglycerate phosphatase degrades 2,3BPG to 3-phosphoglycerate, a normal intermediate of glycolysis. The 2,3BPG shunt thus operates with the expenditure of 1 equivalent of ATP per triose passed through the shunt. The process is not reversible under physiological conditions Reaction #8: 3-phosphoglycerate 2-phosphoglycerate It is reversabile reaction Phosphoglycerate Mutase catalyses the isomerization Reaction #9: 2-phosphoglycerate Phosphoenolpyruvate + H2O It is reversabile reaction Enolase catalyses the dehydration reaction 18 Reaction #10: Phosphoenolpyruvate + ADP + H+ → Pyruvate + ATP It is irreversabile reaction Pyruvate Kinase catalyses the substrate-level phosporylation of ADP to produce ATP Energy yield of glycolysis a. Aerobic glycolysis requires two equivalents of ATP to activate the process, with the subsequent production of four equivalents of ATP and two equivalents of NADH. Thus, conversion of one mole of glucose to two moles of pyruvate is accompanied by the net production of two moles each of ATP and NADH. Glucose + 2 ADP + 2 NAD+ + 2 Pi ——> 2 Pyruvate + 2 ATP + 2 NADH + 2H+ + 2H2O The NADH generated during glycolysis is used to fuel mitochondrial ATP synthesis via oxidative phosphorylation, producing either 2 or 3 equivalents of ATP depending upon the type of shuttle Glycerol phosphate shuttle →(2 ATP) Or the malate-aspartate shuttle →(3ATP) 19 The net yield from the oxidation of 1 mole of glucose to 2 moles of pyruvate is, therefore, either 6 or 8 moles of ATP. Complete oxidation of the 2 moles of pyruvate, through the TCA cycle, yields an additional 30 moles of ATP The total yield, therefore being either 36 or 38 moles of ATP from the complete oxidation of 1 mole of glucose to CO2 and H2O. b.Anaerobic Glycolysis Under anaerobic conditions and in erythrocytes under aerobic conditions, pyruvate is converted to lactate by the enzyme lactate dehydrogenase (LDH), The lactate is transported out of the cell into the circulation. The conversion of pyruvate to lactate, under anaerobic conditions, provides the cell with a mechanism for the oxidation of NADH (produced during the G3PDH reaction) to NAD+ which occurs during the LDH catalyzed reaction. This reduction is required since NAD+ is a necessary substrate for G3PDH, without which glycolysis will cease. Normally, during aerobic glycolysis the electrons of cytoplasmic NADH are transferred to mitochondrial carriers of the oxidative phosphorylation pathway generating a continuous pool of cytoplasmic NAD+. Glucose + 2 ADP + 2 Pi 2 Lactate + 2 ATP + 2 H2O 20 Regulation of glycolysis Stimulant Rate limiting steps Inhibitors Glucokinase Phosphofructokinase Insulin Glucagon Pyruvate kinase ATP F-1,6-BP Pyruvate kinase cAMP & acetyl-CoA Well fed state Fasting state ↑Ingestion of glucose ↓Blood glucose ↑Blood glucose ↑Release of glucagon ↑Release of insulin Phosphofructokinase ↑cAMP ↑Protein phosphatase ↑protein kinase activity ↑F-2,6-BP ↓F-2,6-BP AMP & ADP Phosphofructokinase Citrate F-2,6-BP : F-2,6-BP is not a glycolytic intermediates The synthesis of F2,6BP is catalyzed by the bifunctional enzyme phosphofructokinase-2/fructose- 2,6-bisphosphatase (PFK-2/F-2,6-BPase). Dephosphorylation of the enzyme activates PFK-2 and serves to catalyze the synthesis of F-2,6-BP by phosphorylating fructose 6-phosphate. The result is that the activity of PFK-1 is greatly stimulated and the activity of F-1,6-BPase is greatly inhibited. Thus the glycolytic direction is greatly enhanced. phosphorylation of the bifunctional enzyme inactivates PFK-2 and activates F-2,6-BPase, thus leading to decreased formation of F-2,6-BP and inhibition of the glycolytic pathway. 21 Pasteur Effect If the oxygen concentration grows, pyruvate is converted to acetyl CoA that can be used in the Krebs Cycle, → 38 moles of ATP per mole of glucose. The ATP production increases and the rate of glycolysis slows, because the ATP produced acts as an allosteric inhibitor for PFK-1 Covalent modification of Pyruvate kinase Glucagon & adrenaline stimulation adenyl cyclase PKa ATP cAMP +ve protein kinase phosphatase PKb Some comments on the glycolytic pathway 1- The reaction catalyzed by enolase is inhibited by fluoride, and so when blood samples are taken for measurement of glucose, it is collected in tubes containing fluoride to inhibit glycolysis. 2- Pyruvate kinase deficiency cause hemolytic anemia - Mature erythrocytes contain no mitochondria, totally dependent upon glycolysis for ATP production. - ATP (only from glycolysis) is required for Na+/K+-ATPase-ion transport system which maintains the proper shape of the erythrocyte membrane. 22 Metabolic fates of puruvate within the mitochondria: When transported into the mitochondrion, pyruvate encounters two principal metabolizing enzymes: pyruvate carboxylase (a gluconeogenic enzyme) and pyruvate dehydrogenase (PDH) The fate of pyruvate depends on the cell energy charge. In cells or tissues with a high energy charge pyruvate is directed toward gluconeogenesis; whereas coenzyme A (CoA) is highly acylated, as acetyl-CoA, and allosterically activate pyruvate carboxylase, When the energy charge is low pyruvate is preferentially oxidized to CO2 and H2O in the TCA cycle; whereas CoA is not acylated, pyruvate carboxylase is inactive, and pyruvate is preferentially metabolized via the PDH complex and the enzymes of the TCA cycle. The Pyruvate Dehydrogenase (PDH) Complex The PDH complex is comprised of 3 separate enzymes and 5 different coenzymes: CoA, NAD+, FAD+, lipoic acid and thiamine pyrophosphate (TPP). The pathway of PDH oxidation of pyruvate to acetyl-CoA is diagrammed Regulation of the PDH Complex 23 The Catabolism of Acetyl-CoA: The Citric Acid Cycle: krebs cycle:Tricarboxylic acid cycle ―TCA‖ Biomedical importance The cycle is the major route for the generation of ATP and is located in the matrix of mitochondria adjacent to the enzymes of the respiratory chain and oxidative phosphorylation. The citric acid cycle is amphibolic (catabolic and anabolic) because 1.The citric acid cycle is the final pathway for the oxidation of carbohydrate, lipid, and protein whose common end-metabolite, acetyl-CoA (catabolic role). 2. It is important in the provision of carbon skeletons for gluconeogenesis, fatty acid synthesis, and interconversion of amino acids (anabolic role). A- Role in amino acid synthesis by transamination and deamination e.g. Pyruvate Alanine Oxaloacetate aspartate α-Ketoglutarate glutamate GABA B- Role in Gluconeogenesis All the intermediates of the cycle are potentially glucogenic, since they can give rise to oxaloacetate, and hence net production of glucose (in the liver and kidney) The key enzyme that catalyzes net transfer out of the cycle into gluconeogenesis is phosphoenolpyruvate carboxykinase, which catalyzes the decarboxylation of oxaloacetate to phosphoenolpyruvate. C- Role in fatty acid synthesis e.g. acetyl CoA fatty acid Citrate acetyl CoA fatty acid D- Role in other metabolic reactions e.g. Succinyl CoA Heme Succinyl CoA ketone bodies metabolism (ketolysis) 24 Involvement of the citric acid cycle in transamination and gluconeogenesis. The bold arrows indicate the main pathway of gluconeogenesis Participation of the citric acid cycle in fatty acid synthesis from glucose. The acetyl groups are fed into the citric acid cycle, which enzymatically oxidizes them to CO2. The energy released by oxidation is conserved in the reduced electron carriers NADH and FADH2. Four of the B vitamins are essential in the TCA cycle and therefore in energy-yielding metabolism: (1) Riboflavin (B2), in the form of flavin adenine dinucleotide (FAD), a cofactor in the α- ketoglutarate dehydrogenase (2) Niacin, in the form of nicotinamide adenine dinucleotide (NAD) (3) Thiamin ( B1), as TPP (4) Pantothenic acid, as part of coenzyme A, the cofactor attached to ―active‖ carboxylic acid residues such as acetyl-CoA and succinyl-CoA. 25 Tricarboxylic Acid Cycle (TCA) 26 Citrate Synthase (Condensing enzyme): The first reaction of the cycle is condensation of acetyl-CoA with oxaloacetate (OAA). When the cellular energy charge increases the rate of flux through the TCA cycle will decline leading to a build-up of citrate. Excess citrate is used to transport acetyl-CoA carbons from the mitochondrion to the cytoplasm where they can be used for fatty acid and cholesterol biosynthesis. The highly exergonic nature of the citrate synthase reaction is of central importance in keeping the entire cycle going in the forward direction i.e. irreversible direction Aconitase: Citrate is isomerized to isocitrate by the enzyme aconitase; the reaction occurs in two steps: dehydration to cis-aconitate, some of which remains bound to the enzyme; and rehydration to isocitrate. Aconitase is inhibited by fluoroacetate, a compound that is used as a rat poison. Isocitrate Dehydrogenase: It catalyzes the irreversible oxidative decarboxylation of isocitrate, yielding NADH molecules and the first release of CO2 CO2 is the original C-1 carbon of the OAA used in the citrate synthase reaction. Low-energy signal (ADP and AMP) allosterically activates the enzyme, while high-energy charge ( ATP and NADH) inhibits it α-Ketoglutarate Dehydrogenase (α-KGDH) Complex: This mitochondrial multienzyme complex is very similar to the PDH complex in the intricacy of its protein makeup, cofactors, and its mechanism of action. α-KGDH complex is inhibited by ATP, GTP, NADH, and succinyl CoA, and activated by Ca++. However, it is not regulated by phosphorylation/dephosphorylation reactions. α-ketoglutarate (α-KG) represents a key metabolite linking the entry and exit of carbon atoms from the TCA cycle to pathways involved in amino acid metabolism. Succinyl-CoA, along with glycine, contributes all the carbon and nitrogen atoms required for protoporphyrin heme biosynthesis and for non-hepatic tissue utilization of ketone bodies. Succinyl CoA Synthetase (Succinate Thiokinase ): Tissues in which gluconeogenesis occurs (the liver and kidney) contain two isoenzymes of succinate thiokinase, one specific for GDP and the other for ADP Mitochondrial GTP is used in a trans-phosphorylation reaction to produce ATP and regenerating GDP for the continued operation of succinyl CoA synthetase. GTP + ADP phosphokinase GDP + ATP 27 Succinate Dehydrogenase (SDH): Is bound to the inner surface of the inner mitochondrial membrane. Fumarase (fumarate hydratase): Catalyzes the addition of water across the double bond of fumarate, yielding malate. Fumarate is also produced by the urea, and during catabolism of the amino acids Malate Dehydrogenase (MDH): Although the equilibrium of this reaction strongly favors malate, the net flux is toward the direction of OAA because of 1.The continual removal of OAA (either to form citrate, as a substrate for gluconeogenesis, or to undergo transamination to aspartate) 2.The continual reoxidation of NADH. Regulation of the TCA Cycle Since three reactions of the TCA cycle utilize NAD+ as co-factor it is not difficult to understand why the cellular ratio of NAD+/NADH has a major impact on the flux of carbon through the TCA cycle. Product inhibition also controls the TCA flux, e.g. citrate inhibits CS, and α-KGDH is inhibited by NADH and succinyl-CoA The key enzymes of the TCA cycle (CS, IDH, and α-KGDH) are also regulated allosterically by Ca2+, ATP and ADP. ATP NADH Succinyl CoA Acyl CoA 28 Energy produced by the TCA cycle Energy producing Number of ATP reaction produced 3 NADH 9 FADH2 2 GTP = ATP 1 12 ATP ATP Formation in the Catabolism of Glucose 4 or 6 8 or 10 Net 6 or 8 6 6 6 Citric acid cycle 4 6 Net 30 36 or 38 29 Anaplerotic (filling up) Reactions With depletion of oxaloacetate, it is impossible to continue oxidizing acetyl CoA. To enable the TCA cycle to keep running, cells have to supply enough four-carbon intermediates from degradation of carbohydrate or certain amino acids to compensate for the rate of removal. Pyruvate carboxylase is the main anaplerotic enzyme in the cell. Amino acid degradation forms TCA cycle intermediates 30 Gluconeogenesis Introduction Gluconeogenesis is the biosynthesis of new glucose from non carbohydrate precursors, (i.e. not glucose from glycogen). The production of glucose from other metabolites is necessary for use as a fuel source by the brain, testes, erythrocytes and kidney medulla since glucose is the sole energy source for these organs. The primary carbon skeletons used for gluconeogenesis are derived from pyruvate, lactate, glycerol, and the amino acids alanine and glutamine. The liver is the major site of gluconeogenesis, however, as discussed below, the kidney also has an important part to play in this pathway. Synthesis of glucose from three and four carbon precursors is essentially a reversal of glycolysis. The relevant features of the pathway of gluconeogenesis are diagrammed below: 31 Importance of gluconeogenesis 1- Gluconeogenesis meets the needs of the body for glucose when insufficient carbohydrate is available from the diet or glycogen reserves. 2- A continual supply of glucose is necessary especially for the brain and erythrocytes. Severe Hypoglycemia causes brain dysfunction, which can lead to coma and death. 3- Glucose is the only fuel that supplies energy to skeletal muscle under anaerobic condition. 4- Glucose is the precursor of milk sugar ―lactose‖ in mammary gland. 5- gluconeogenesis clears lactate produced by muscles and erythrocytes and glycerol produced by adipose tissue. The sequences of gluconeogenesis that do not use enzymes of glycolysis involve the irreversible, regulated steps of glycolysis. These three steps are the conversion of: 1-Pyruvate to Phosphoenolpyruvate (PEP) a- In mitochondria pyruvate converts to oxaloacetate b- In cytosol oxaloacetate converts to PEP 32 Pyruvate carboxylase is allosterically activated by acetyl-CoA during fasting in which OAA is used for the synthesis of glucose by gluconeogenesis in the liver and kidney Oxaloacetate can‘t cross the inner mitochondrial membrane. Thus it transformed into malate that can cross and reoxidized in cytosol to OX.AC (dicarboxylic shuttle) (see the figure) 2-Fructose- 1,6- bisphosphate to Fructose-6-phosphate, Bypass 2 F-1,6-BP F-1,6-Bisphosphatase F-6-P F-2,6-BP (-) H20 Pi 3-Glucose-6-phosphate (G6P) to Glucose (or Glycogen), Bypass 3 G-6P glucose-6-phosphatase Glucose H20 Pi Substrates for Gluconeogenesis Lactate It is formed in RBCs and in skeletal muscle during severe exercise. Lactate is converted back to glucose in liver through gluconeogenesis. The bloodstream carries the glucose back to the muscles for oxidation or storage as glycogen. This process is known as the Cori cycle, or the lactic acid cycle. 33 Pyruvate: Pyruvate, generated in muscle and other peripheral tissues, can be transaminated to alanine which is returned to the liver for gluconeogenesis This pathway is termed the glucose-alanine cycle. Although the majority of amino acids are degraded in the liver some are deaminated in muscle. The glucose-alanine cycle is, therefore, an indirect mechanism for muscle to eliminate nitrogen while replenishing its energy supply. However, the major function of the glucose-alanine cycle is to allow non-hepatic tissues to deliver the amino portion of catabolized amino acids to the liver for excretion as urea. Amino Acids: All 20 of the amino acids, excepting leucine and lysine can be degraded to TCA cycle intermediates. This allows the carbon skeletons of the amino acids to be converted to those in oxaloacetate and subsequently into pyruvate. The pyruvate thus formed can be utilized by the gluconeogenic pathway. When glycogen stores are depleted, in muscle during exertion and liver during fasting, catabolism of muscle proteins to amino acids contributes the major source of carbon for maintenance of blood glucose levels. 34 Glycerol The glycerol backbone of lipids can be used for gluconeogenesis. This requires phosphorylation to glycerol-3-phosphate by glycerol kinase and dehydrogenation to dihydroxyacetone phosphate (DHAP) by glycerol-3-phosphate dehydrogenase Propionate: Oxidation of fatty acids with an odd number of carbon atoms and the oxidation of some amino acids generates as the terminal oxidation product, propionyl-CoA. Propionyl-CoA is converted to the TCA intermediate, succinyl-CoA. 35 Regulation of glycolysis and gluconeogenesis Glycolysis and gluconeogenesis are regulated reciprocally Regulation of the two pathways primarily is brought about by allosteric controls on the enzymes that differ between the two pathways. These enzymes are: Glyolysis Enzyme Gluconeogenesis Enzyme Hexokinase Glucose-6-phosphatase Phosphofructokinase-1 (PFK-1) Fructose 1,6 bisphosphatase Pyruvate kinase 1. Pyruvate Carboxylase 2. Phosphoenolpyruvate carboxykinase (PEPCK) 1.F2,6BP and AMP activate PFK-1 and inhibit F- 1,6-bisphosphatase 2.G6P substrate levels control Hexokinase and Glucose-6-phosphatase 3.Acetyl-CoA inhibits Pyruvate kinase and activates Pyruvate Carboxylase Fructose 2,6 bisphosphate. The major allosteric regulatory factor of the two pathways is Fructose 2,6 bisphosphate. Note that PFK-2 and Fructose 2,6-bisphosphatase are on the same peptide and are affected differently by phosphorylation. Interconversion of PFK-2 and Fructose 2,6-bisphosphatase depends on the level of cAMP (which is stimulated by glucagon and epinephrine and is inhibited by insulin). Increasing cAMP (glucagon/epinephrine) stimulates phosphorylation of PFK-2 and Fructose 2,6-bisphosphatase, favoring the Fructose 2,6-bisphosphatase. Decreasing cAMP (insulin) stimulates dephosphorylation, favoring PFK-2. In fasting state 1.↓Blood glucose → ↑ glucagon → ↑cAMP → ↑ protein kinase activity →↓ F-2,6-BP → ↑F- 1,6-bisphosphatase 2.↑ protein kinase activity →↓Pyruvate kinase →↓conversion PEP to pyruvate→↑conversion of PEP to glucose 3.↑Release of fatty acids from adipose tissue →↑fatty acid oxidation in liver →↑acetyl-CoA in liver→(+) pyruvate carboxylase In fed state ↑Blood glucose → ↑insulin release →↑ Protein phosphatase → ↑ F-2,6-BP → ↓F- 1,6- bisphosphatase 36 Hormonal regulation 1- Insulin inhibits gluconeogensis by decreasing transcription of genes coding for 4 enzymes unique to pathway : Pyruvate carboxylase PEP-carboxykinase Fructose 1,6-bisphosphatase glucose 6-phosphatase 2- Cortisol, epinephrine, glucagon + starvation + diabetes mellitus stimulate gluconeogenesis by increasing transcription of genes of previous enzymes. Glucogenic substrate availability Glucogenic amino acids, markedly increase the rate of gluconeogenesis. Glycerol ( in fasting, diabetes or stress due to increased lipolysis in adipose tissue), favors rate of gluconeogenesis. Lactate ( in blood by muscular exercise and by RBCs), can be converted to glucose in liver (Cori cycle). Consumption of ATP and NADH in gluconeogenesis = 12 ATP and this ENERGY is obtained from β-oxidation of fatty acids 37 Glycogen Metabolism Blood glucose can be obtained from 3 primary sources: 1- diet (is not always a reliable source of blood glucose). 2- gluconeogenesis (slow in responding to a falling blood glucose level). 3- degradation of glycogen ―glycogenolysis‖ (supply of glucose in a rapidly mobilizable form, namely, glycogen). Glycogen is the major storage carbohydrate in animals.It occurs mainly in liver (up to 6%) and muscle, where it rarely exceeds 1%. However, because of its greater mass, muscle contains about three to four times as much glycogen as does liver. The function of muscle glycogen is to serve as a fuel reserve for the synthesis of ATP during muscle contraction. That of liver glycogen is to maintain the blood glucose concentration between meals. After 12–18 hours of fasting, the liver glycogen is almost totally depleted. Glycogen storage diseases are a group of inherited disorders characterized by deficient mobilization of glycogen or deposition of abnormal forms of glycogen, leading to muscular weakness or even death. 38 GLYCOGENIN SYNTHESIS Glycogen is synthesized from molecules of α-D-glucose. The process occurs in the cytosol, and requires energy supplied by ATP (for the phosphorylation of glucose) and uridine triphosphate (UTP). Synthesis of a primer to initiate glycogen synthesis Glycogen synthase cannot initiate chain synthesis using free glucose as an acceptor of a molecule of glucose from UDP-glucose. A fragment of glycogen can serve as a primer in cells whose glycogen stores are not totally depleted. In the absence of a glycogen fragment, a protein, called glycogenin, can serve as an acceptor of glucose residues Transfer of the first few molecules of glucose from UDP-glucose to glycogenin is catalyzed by glycogenin itself. SYNTHESIS OF GLYCOGEN (GLYCOGENESIS) 1. As in glycolysis, glucose is phosphorylated to G-6-P, catalyzed by hexokinase in muscle and glucokinase in liver. 2. G-6-P is isomerized to G-1-P by phosphoglucomutase. 3. G-1-P reacts with UTP to form the active nucleotide uridine diphosphate glucose (UDPGlc)* and pyrophosphate, catalyzed by UDPGlc pyrophosphorylase. 4. Glycogen synthase catalyzes the formation of a glycoside bond between C1 of the activated glucose of UDPGlc and C4 of a terminal glucose residue of glycogen, liberating UDP. 5. When the chain has been lengthened to at least 11 glucose residues, branching enzyme transfers a part of at least 6 glucose residues to a neighboring chain. 6. Branching increases the number of nonreducing ends to which new glucosyl residues can be added and also can be removed. 39 DEGRADATION OF GLYCOGEN (GLYCOGENOLYSIS) 1.Glycogen phosphorylase catalyzes the rate-limiting step in glycogenolysis by promoting the phosphorylytic cleavage by inorganic phosphate of the 1→4 linkages of glycogen to yield G-1P. 2. The terminal glucosyl residues from the outermost chains of the glycogen molecule are removed sequentially until approximately four glucose residues remain on either side of a 1→6 branch 3.Glucan transferase (4:4 transferase) transfers a 3 glucosyl unit from one branch to the other, exposing the 1→6 branch point. 4. Hydrolysis of the 1→6 linkages requires the debranching enzyme. Reciprocal regulation of glycogen phosphorylase and glycogen synthase Glycogen breakdown and synthesis is regulated by hormones. Epinephrine, glucagon, and thyroxin stimulate breakdown. Insulin stimulates synthesis. Glucocorticoid and growth hormone increase liver glycogen due to increased gluconeogenesis Glucagon doesn‘t affect muscle glycogen because there is no receptors glycogen phosphorylase and glycogen synthase control glycogen metabolism in both liver and muscle cells regulation occur by phosphorylation and dephosphorylation catalyzed by protein kinases and protein phosphatases of glycogen phosphorylase and glycogen synthase 40 1- Allosteric regulation of glycogen synthesis and degradation 2- Regulation via covalent modification A- Activation of glycogen degradation by cAMP-directed pathway 41 B. Inhibition of glycogen synthesis by a cAMP-directed pathway 42 Glycogen Storage Diseases ―Glycogen storage disease‖ is a generic term to describe a group of inherited disorders characterized by deposition of an abnormal type or quantity of glycogen in tissues, or failure to mobilize glycogen. 43 PENTOSE PHOSPHATE PATHWAY (PPP) = Hexose monophosphate pathway ―HMP‖ = 6-phosphogluconate pathway The pentose phosphate pathway (PPP) is primarily an anabolic pathway that utilizes the 6 C of glucose to generate 5 C sugars and reducing equivalents. However, this pathway does oxidize glucose and under certain conditions can completely oxidize glucose to CO2 and water. The enzymes of the PPP are cytosolic. PPP doesn‘t generate ATP The sequence of reactions of the pathway may be divided into two phases: an oxidative nonreversible phase and a nonoxidative reversible phase. 44 A.The oxidation reactions: They utilize glucose-6-phosphate (G-6-P) as the substrate, occur at the beginning of the pathway and are the reactions that generate NADPH. The reactions catalyzed by: Glucose-6-phosphate dehydrogenase 6-phosphogluconolactonase 6-phosphogluconate dehydrogenase B.The non-oxidative reactions: They are primarily designed to generate R-5-P. It converts dietary 5 carbon sugars into both 6 (F-6-P) and 3 (glyceraldehyde-3-P) carbon sugars which can then be utilized by the pathways of glycolysis. Transketolase transfer 2 carbon groups from substrates and requires TPP as a co-factor, while Transaldolase transfers 3 carbon groups. The reactions catalyzed by: Isomerase Epimerase Transketolase Transaldolase The primary functions of this pathway are: 1.To generate reducing equivalents, in the form of NADPH, for reductive biosynthesis reactions of fatty acids, cholesterol and steroids within cells 2.To provide the cell with R-5-P for the synthesis of the nucleotides and nucleic acids. N.B. It is not necessary to have a completely functioning PPP for a tissue to synthesize ribose 5- phosphate. Muscle has only low activity of glucose 6-phosphate dehydrogenase and 6-phosphogluconate dehydrogenase, but, like most other tissues, it is capable of synthesizing ribose 5-phosphate by reversal of the nonoxidative phase of the PPP utilizing fructose 6-phosphate. 3. Although not a significant function of the PPP, it can operate to metabolize dietary pentose sugars derived from the digestion of nucleic acids as well as to rearrange the carbon skeletons of dietary carbohydrates into glycolytic/gluconeogenic intermediates. NADPH: Enzymes that function primarily in the reductive direction utilize the NADP+/NADPH cofactor pair as co-factors as opposed to oxidative enzymes that utilize the NAD+/NADH cofactor pair. Cells of the liver, adipose tissue, adrenal cortex, testis and lactating mammary gland have high levels of the PPP enzymes. In fact 30% of the oxidation of glucose in the liver occurs via the PPP. Additionally, erythrocytes utilize the reactions of the PPP to generate large amounts of NADPH used in the reduction of glutathione The conversion of ribonucleotides to deoxyribonucleotides (through the action of ribonucleotide reductase) requires NADPH as the electron source, therefore, any rapidly proliferating cell needs large quantities of NADPH. 45 . Cytochrome P450 monooxygenase system (detoxification of drugs) Synthesis of nitric oxide Erythrocytes and the PPP The PPP supplies the RBC with NADPH to maintain the reduced state of glutathione. The inability to maintain reduced glutathione in RBCs leads to increased accumulation of peroxides, predominantly H2O2, that in turn results in a weakening of the cell wall and concomitant hemolysis. 46 Accumulation of H2O2 also leads to increased rates of oxidation of hemoglobin to methemoglobin that also weakens the cell wall. Glutathione removes peroxides via the action of glutathione peroxidase. Deficiency in the level of activity of G-6-PDH is the basis of favism, primaquine (an anti-malarial drug) sensitivity and some other drug-sensitive hemolytic anemias, anemia and jaundice in the newborn. In addition, G-6-PDH deficiencies are associated with resistance to the malarial parasite, Plasmodium falciparum, among individuals of Mediterranean and African descent. The basis for this resistance is the weakening of the red cell membrane (the erythrocyte is the host cell for the parasite) such that it cannot sustain the parasitic life cycle long enough for productive growth. Precipitating factors in G6PD deficiency 1- Oxidant drugs Antibiotics (e.g. sulfamethoxazole and chloramphenicol), Antimalarials (e.g. primaquine but not quinine), and Antipyretics (e.g. acetanilid but not acetaminophen) 2. Favism (the hemolytic effect of ingesting fava beans) 3. Infection The inflammatory response to infection results in the generation of free radicals in macrophages, which can diffuse into the red blood cells and cause oxidative damage Phagocytosis and the PPP The highest levels of PPP enzymes (in particular G-6-PDH) are found in neutrophils and macrophages. These leukocytes are the phagocytic cells of the immune system and they utilize NADPH to generate superoxide radicals from molecular oxygen in a reaction catalyzed by NADPH oxidase to kill the phagocytized microorganisms. So any defect in enzymes in this process can result in impaired killing of infectious organisms leading to chronic granulomatous disease. Regulation of PPP The regulated step is G-6-PDH which is strongly inhibited by NADPH. The synthesis of G-6-PDH and 6-phosphogluconate DH may also be induced by insulin during conditions associated with the ―fed state‖ when lipogenesis increases 47 METABOLISM OF MAJOR NON-GLUCOSE SUGARS 1.FRUCTOSE METABOLIM Metabolism of fructose. Aldolase A is found in all tissues, whereas aldolase B is the predominant form in liver. (*, not found in liver.) 48 Diets containing large amounts of sucrose (a disaccharide of glucose and fructose) can utilize the fructose as a major source of energy. Muscle which contains only hexokinase can phosphorylate F to F-6-P which is a direct glycolytic intermediate. In the liver which contains mostly glucokinase, which is specific for glucose as its substrate, requires the function of additional enzymes to utilize fructose in glycolysis. Hepatic fructose is phosphorylated on C–1 by fructokinase yielding F-1-P. In liver the form of aldolase that predominates (aldolase B) can utilize both F-1,6-BP and F-1-P as substrates. Clinical Significances of Fructose Metabolism 1.Essential fructosuria: It is a benign metabolic, asymptomatic and harmless disorder due to deficiency of fructokinase Fructose is accumulated in blood and detected in urine 2.Hereditary fructose intolerance (HFI): It is a potentially lethal disorder resulting from a lack of aldolase B which is normally present in the liver, small intestine and kidney cortex. F-1-P accumulates, and ATP and inorganic phosphate levels fall significantly F-1-P inhibits glycogenolysis, by interfering with the phosphorylase reaction The decreased availability of hepatic ATP affects gluconeogenesis (causing hypoglycemia, vomiting, jaundice, hemorrhage, hepatomegaly, renal dysfunction, hyperuricemia, and lacticacidemia.) If fructose (and, therefore, sucrose or sorbitol) is not removed from the diet, liver failure and death can occur. 3.Hereditary fructose-1,6-bisphosphatase deficiency: Results in severely impaired hepatic gluconeogenesis and leads to episodes of hypoglycemia, apnea, hyperventillation, ketosis and lactic acidosis. These symptoms can take on a lethal course in neonates. One consequence of HFI and of hereditary fructose 1,6-bisphosphatase deficiency is fructose- induced hypoglycemia despite the presence of high glycogen reserves, because of fructose 1- phosphate and fructose 1,6-bisphosphate allosterically inhibit liver phosphorylase. Conversion of glucose to fructose via sorbitol The pathway in the seminal vesicles is a major carbohydrate energy source The effect of hyperglycemia on sorbitol metabolism: Both fructose and sorbitol are found in the lens of the eye in increased concentrations The lens, peripheral nerves, and renal glomeruli are not insulin-sensitive. Sorbitol does not diffuse through cell membranes easily and accumulates, causing osmotic damage, resulting in cataract formation, retinopathy, peripheral neuropathy, and nephropathy. 49 The effect of hyperglycemia on sorbitol metabolism Because insulin is not required for the entry of glucose into the cells of lens, retina, nerves, liver, kidney, placenta, RBCs, ovaries and seminal vesicles, large amounts of glucose may enter these cells during times of hyperglycemia (e.g. diabetes mellitus). Elevated intracellular glucose concentrations and an adequate supply of NADPH cause aldose reductase to produce a significant increase in the amount of sorbitol, which cannot pass efficiently through cell membranes and, therefore, remains trapped inside the cell. Sorbitol dehydrogenase is low or absent in retina, lens, kidney, and nerve cells. As a result, sorbitol accumulates in these cells, causing strong osmotic effects and, therefore, cell swelling as a result of water retention. Some of the pathologic alterations associated with diabetes can be attributed, in part, to this phenomenon, including cataract formation, peripheral neuropathy, and microvascular problems leading to nephropathy and retinopathy. 50 II.GALACTOSE METABOLIM Pathway of conversion of (A) galactose to glucose in the liver and (B) glucose to lactose in the lactating mammary gland. Clinical Significances of Galactose Metabolism: The major dietary source of galactose is lactose (galactosyl β-1,4-glucose) obtained from milk and milk products. Like fructose, the entry of galactose into cells is not insulin-dependent. Galactose is required in the body not only in the formation of lactose but also as a constituent of glycolipids (cerebrosides), proteoglycans, and glycoproteins. The enzymes for galactose conversion to glucose 1-P are present in many tissues, including the adult erythrocyte, fibroblasts, and fetal tissues. The liver has a high activity of these enzymes, and can convert dietary galactose to blood glucose and glycogen. galactosemia results from loss of galactose-1-phosphate uridyl transferase and galactokinase. Vomiting and diarrhea occur following ingestion of milk, hence individuals are termed lactose intolerant. Cause hypergalactosemia, metabolic acidosis, and urinary galactitol excretion. 51 Unless controlled by exclusion of galactose from the diet, these galactosemias can go on to produce blindness and fatal liver damage. Conversion of circulating galactose to galacitol, by an NADPH-dependent galactose reductase that is present in neural tissue and in the lens of the eye. galacitol in the lens causes osmotic swelling, with the resultant formation of cataracts and other symptoms. The principal treatment of these disorders is to eliminate lactose from the diet. Lactose (Milk sugar) synthesis Lactose is a disaccharide composed of galactose and glucose. Lactose is synthesized only in the mammary gland of the adult during lactation. Lactose synthase present in lactating mammary gland catalyzes the transfer of galactose from UDP-galactose to glucose. Lactose synthase is composed of two protein subunits a galactosyltransferase and α-lactalbumin, which is a modifier protein synthesized after parturition (childbirth) in response to the hormone prolactin. However, galactose is not required in the diet for lactose synthesis because galactose can be synthesized from glucose by the reversible action of epimerase. 52 Disorders of galactose metabolism A- Classical galactosemia deficiency of galactose 1-phosphate uridylyltransferase results in the accumulation of galactose 1-phosphate in tissues and the appearance of galactose in the blood and urine, Causes galactosemia and galactosuria, vomiting, diarrhea, and jaundice. Accumulation of galactose 1-phosphate and galactitol in nerve, lens, liver, and kidney tissue causes liver damage, severe mental retardation, and cataracts. Prenatal diagnosis is possible by chorionic villus sampling. Newborn screening is available. Therapy: Rapid diagnosis and removal of galactose (and therefore lactose) from the diet. B- Nonclassical galactosemia Deficiency of galactokinase results in the accumulation of galactose Causes elevation of galactose in blood (galactosemia) and urine (galactosuria). Causes galactitol accumulation if galactose is present in the diet. Elevated galactitol can cause cataracts. Treatment is dietary restriction. Metabolism of galactose 53 GLUCURONATE METABOLISM (Uronic acid pathway) L-xylulose reductase L-gulonolactone oxidase Uronic acid pathway. (Asterisk indicates the fate of carbon 1 of glucose) 54 The uronic acid pathway is an alternative pathway for the oxidation of glucose that does not provide a means of producing ATP The uronic acid pathway catalyzes the conversion of glucose to glucuronic acid, ascorbic acid (except in human and other species for which ascorbate is a vitamin), and pentoses. UDP-glucuronate is used in the synthesis of glycosaminoglycan and proteoglycans as well as forming complexes with bilirubin, steroids and certain drugs. It takes place in the cytoplasm of liver Clinical Significance of Glucuronate In the liver, bilirubin is solubilized by conjugation to glucuronate. The soluble conjugated bilirubin diglucuronide is then secreted into the bile. An inability to conjugate bilirubin, for instance in hepatic disease or when the level of bilirubin production exceeds the capacity of the liver, is a contributory cause of jaundice. The conjugation of glucuronate to certain non-polar drugs is important for their solubilization in the liver. Glucuronate-conjugated drugs are more easily cleared from the blood by the kidneys for excretion in the urine. The glucuronate-drug conjugation system can, lead to drug resistance; chronic exposure to certain drugs, such as barbiturates, leads to an increase in the synthesis of the UDP- glucuronyltransferases and results in a higher rate of drug clearance leading to a reduction in the effective dose of glucuronate-cleared drugs. Glucuronate is reduced to L-gulonate, the direct precursor of ascorbate in those animals capable of synthesizing this vitamin, in an NADPH-dependent reaction. In humans and other primates, as well as guinea pigs, bats, and some birds and fishes, ascorbic acid cannot be synthesized because of the absence of L-gulonolactone oxidase. 55 Metabolic Effects of Insulin, Glucagon and Epinephrine Action of Insulin Insulin promotes 1. Uptake of fuel substrates into some cells; 2. Storage of fuels (lipids and glycogen); and 3. Biosynthesis of macromolecules (nucleic acids and protein). Because insulin promotes biosynthesis, insulin can be considered a growth hormone. Specific effects of insulin include the following: 1. Increased uptake of glucose in muscle and adipose tissue; 2. Activation of glycolysis in liver; 3. Increased synthesis of fatty acids and triacylglycerols in liver and adipose tissue; 4. Inhibition of gluconeogenesis in liver; 5. Increased glycogen synthesis in liver and muscle; 6. Increased uptake of amino acids into muscle with consequent activation of muscle protein synthesis; and 7. Inhibition of protein degradation. Insulin stimulates glucose uptake into muscle and adipose cells, at least partly by translocating the glucose transporter (a membrane protein that carries out facilitated diffusion of glucose) from the cytosol (where it resides in the absence of insulin) to the cell surface, in response to insulin. Action of Glucagon Glucagon is a 3.5-kilodalton polypeptide hormone synthesized by the α cells of the islets of Langerhans in the pancreas. These endocrine cells sense the blood glucose concentration and release glucagon in response to low levels. In the liver, glucagon increases cAMP levels, to promote glycogenolysis and inhibit glycogen synthesis. In addition, cAMP inhibits glycolysis and activates gluconeogenesis by activating the hydrolysis of fructose-2,6-bisphosphate, In the liver, inhibition of pyruvate kinase (PK) causes phosphoenolpyruvate (PEP) to accumulate. The level of pyruvate decreases, both because its synthesis from PEP is blocked and because it continues to be converted to PEP, via the pyruvate carboxylase and phosphoenolpyruvate carboxykinase reactions. Accumulation of PEP promotes gluconeogenesis, while the inhibition of pyruvate kinase diminishes the glycolytic flux rate. Glucagon also raises cAMP levels in adipose tissue. There the chief effect of cAMP is to promote triacylglycerol mobilization via phosphorylation of hormone-sensitive lipase, yielding glycerol and fatty acids. 56 Action of Epinephrine In muscle, epinephrine increases cAMP, with concomitant activation of glycogenolysis and inhibition of glycogen synthesis. Triacylglycerol breakdown in adipose tissue is also stimulated by epinephrine, providing fuel for the muscle tissue. In consequence, glucose uptake into muscle is diminished, contributing to an increase in blood glucose levels. Epinephrine also inhibits insulin secretion and stimulates glucagon secretion. These effects tend to increase glucose production and release by the liver. The net result is to increase blood glucose levels. 57 Maintaining Blood Glucose Levels Blood glucose increases shortly after a carbohydrate-containing meal, which stimulates the secretion of insulin and suppresses the secretion of glucagon, in order to remove glucose from the blood. These effects promote the uptake of glucose into the liver, stimulate glycogen synthesis, and suppress glycogen breakdown. There, increased levels of glycolytic intermediates and fatty acids stimulate triacylglycerol synthesis. Finally, increased glucose uptake into muscle increases levels of substrates for glycogen synthesis in that tissue as well. Several hours later, when dietary glucose is not available, the above events are reversed in order to elevate blood glucose levels. Insulin secretion slows, and glucagon secretion increases. This promotes glycogen breakdown in liver via the cAMP-dependent cascade mechanisms that activate glycogen phosphorylase and inactivate glycogen synthase. Triacylglycerol breakdown in adipocytes is activated as well, via the action of hormone-sensitive lipase, generating fatty acids for use as fuel by liver and muscle. At the same time, the decrease in insulin levels reduces glucose use by muscle, liver, and adipose tissue. Consequently, nearly all the glucose produced in the liver is exported to the blood and is available for use by the brain. 58 Role of the Kidney in Blood Glucose Control Although the liver is the major site of glucose homeostasis, the kidney plays a vital role in the overall process of regulating the level of blood glucose. The kidney carries out gluconeogenesis primarily using the carbon skeleton of glutamine and while so doing allows for the elimination of waste nitrogen and maintaining plasma pH balance. Kidney excrete glucose via glomerular filtration as well as to reabsorb the filtered glucose in the proximal convoluted tubules. The kidneys will filter around 180gm of glucose per day. Of this amount less than 1% is excreted in the urine due to efficient reabsorption. This reabsorption process is critical for maintaining blood glucose homeostasis and for retaining important calories for energy production. Transport of glucose from the tubule into the tubular epithelial cells is carried out by specialized transport proteins termed sodium-glucose co-transporters (SGLTs). There are two SGLTs in the kidney involved in glucose reabsorption, SGLT1 and SGLT2 SGLT2 is responsible for approximately 90% of the glucose reabsorption activity of the kidney. SGLT2 has become the target for therapeutic intervention of the hyperglycemia associated with type 2 diabetes. By specifically inhibiting SGLT2 there will be increased glucose excretion in the urine and thus a lowering of plasma glucose levels Diagrammatic representation of the re-uptake of glucose in the S1 segment of the proximal tubule of the kidney by the Na+-glucose co-transporter SGLT2. Following re-uptake the glucose is transported back into the blood via the action of GLUT2 transporters. The Na+ that is reabsorbed with the glucose is transported into the blood via a (Na+-K+)-ATPase. 59 60 LIPID METABOLISM 1 Biological molecules that are insoluble in aqueous solutions and soluble in organic solvents are classified as lipids. The lipids of physiological importance for humans have four major functions: 1. They serve as structural components of biological membranes. 2. They provide energy reserves, predominantly in the form of triacylglycerols. 3. Both lipids and lipid derivatives serve as vitamins and hormones. 4. Lipophilic bile acids aid in lipid solubilization. Lipids vs. carbohydrates for energy storage: LIPIDS CARBOHYDRATES -CH2- -CHOH- more reduced C, more potential energy per more oxidized C to start with, so less potential C energy available per C slower mobilization in metabolism, but more more rapid mobilization in metabolism even energy available though there's less energy there lower weight per C atom -- esp. useful for more weight per C atom -- but OK for immobile MOBILE organisms (animals) organisms (plants) Classification. There are many different methods of classifying lipids. It can be classified biologically as shown in the following scheme 61 Also, some commonly used classifications of lipids and the general biologic functions of each class are listed in the following table (table 1) Table 1: Classifications and Functions of Lipids Lipid Primary Functions Fatty acids Energy sources, biosynthetic precursors Glycerides' Storage, transport, metabolic intermediates phosphoglycerides Membrane components Ketone bodies Energy sources Sphingolipids Membrane components Ecosanoids Modulators of physiologic activity Cholesterol Membrane component Steriod hormones Modulators of physiologic activity Fatty Acids Composed of a carboxylic acid ―head group‖ and a long hydrocarbon―tail‖ tail generally contains an even number of carbon atoms Hydrocarbon tail can be saturated or unsaturated unsaturated hydrocarbon tails contain one or more double bonds Nomenclature. The most commonly used system for designating the position of double bonds in an unsaturated fatty acid is the delta (Δ) numbering System. The terminal carboxyl carbon is designated carbon 1, and the double bond is given the number of the carbon atom on the carboxyl side of the double bond. For example, palmitoleic acid has 16 carbons and has a double between -: carbons 9 and 10. It is designated as 16:1 :Δ9. The methyl carbon at the other end of fatty acid is known as omega (ω) carbon. The systematic name gives the number of carbons, with the suffix -anoic appended. Structure. The general formula of saturated fatty acids is CH3—(CH2)n—COOH where n specifies the number of methylene groups between the methyl and carboxyl carbons. Table: 2 Common carbon Melting Point Systematic Name Name skeleton (°C) Lauric Dodecanoic 12:0 43.5 Myristic Tetradecanoic 14:0 54.4 Palmitic Hexadecanoic 16:0 62.5 Stearic Octadecanoic 18:0 69.6 Pamitoleic 16:1(Δ9) Hexadecenoic 16:1(Δ9) 1.0 Oleic c/s-Δ9-Octadecenoic 18:1(Δ9) 13.5 Linoleic All Cis-Δ9,Δ12 -Octadecadienoic 18:2(Δ9,12) -11.0 Linolenic All Cis-Δ9,Δ12, Δ15 -Octadecatrienoic 18:3(Δ9,12,15) -11.2 Arachidonic All Cis-Δ5,Δ8, Δ11, Δ14-Eicosatetraenoic 20:4(Δ5,8,11,14) -49.5 62 Source 1.Nonessential fatty acids. All nonessential fatty acids can be synthesized from product of glucose oxidation.They are nonessential in the sense that they don‘t have to be obligatorily included in the diet. 2.Essential fatty acids. Fatty acids of the linoleic (18:2:Δ9, 12) and linolenic (18:3 : Δ9, 12, 15 ) families must be obtained from the diet. There are no human enzyme systems That can introduce a double bond beyond the ninth carbon atom (9-10 position) of a fatty acid chain, and all double bonds that are introduced are separated by three-carbon intervals. This rule, combined with the fact that fatty acid elongation only occurs by two-carbon additions, makes it impossible to synthesize de novo certain polyunsaturated fatty acids. C. Physical properties 1.Fatty acids are detergent-like due to their amphipathic nature; that is, they have nonpolar (CH3) and polar (-COOH) ends and, in biphasic systems, they orient with the polar end associated with water and the nonpolar end associated with the hydrophobic phase. 2.The melting point of fatty acids is related to chain length and degree of unsaturation. longer the chain length, the higher the melting point, and the greater the number of double bonds the lower the melting point (see Table 2). Nutrition and Fatty Acids essential fatty acids: linoleic and α-linolenic fatty acids; must get these from plants ―Good fats‖: high in polyunsaturated Fats. Typical foods include vegetable oils, like olive, canola, sunflower, etc. ―Bad fats‖: high in saturated fats. Classic offenders stearic (beef); palm & coconut oils (found in candy) ―Really bad fats‖: trans fatty acids, result from partial hydrogenation of vegetable oils. Margarine has trans fatty acids. difficult to metabolism; lead to increased cholesterol levels in the blood Lipid peroxidation Peroxidation (auto-oxidation) of lipids is responsible for deterioration of foods and also damage of tissues which causes cancer, inflammatory disease, atherosclerosis, etc. The reaction is initiated by an existing free radical (X.), by light or by metal The deterioration effects cause by free radicals (ROO , RO , OH ) produced during peroxide formation from fatty acids (unsaturated) Anti-oxidants: BHA (butylated hydroxyanisole) and BHT (butylated hydroxytoluene) are antioxidants used as food derivates Vit E, C, Beta carotene, glutathione Catalase, superoxid dismutase, lipooxygenase Rancidity Polyunsaturated fats spoil more easily than saturated fats. Rancidity: Flavor and odor of fat is affected, due to the oxidation of double bonds. 63 To protect polyunsaturated fats from rancidity: a)Refrigeration b)Hydrogenation Triglycerides (triacylglycerols) Structure. Triglycerides are triesters of glycerol and three fatty acids. Function. Fatty acids are converted to triglycerides for transport between tissues and for storage of metabolic fuel. a.The main stores of metabolic fuel in humans are the fat deposits in fat cells (adipocytes). A very large proportion of ingested fats are stored as triglycerides in the fat droplets of the adipocytes, where they serve long-term needs for metabolic fuel. b.Triglycerides have several advantages over other forms of metabolic fuel. 1.Triglycerides are light (less dense than water). They provide a concentrated form of fuel because their complete combustion to CO2 and water releases 9 kcal/g as opposed to 4kcal/g for carbohydrate. 2.Because they are water insoluble, triglycerides present no osmotic problems to the cell even when stored in large amounts. c.Lipolysis. The appearance of fatty acids in the blood during fasting is due to the mobilization of fat stores by the process of lipolysis. Lipolysis involves the hydrolysis of triglycerides to free glycerol and free fatty acids (also known as nonesterified fatty acids (NEFFA)). Free FAs are found in trace quantities in cells. FAs are either: I.part of a lipid molecule II.complexed to a carrier protein (e.g. albumin on blood) Waxes - Esters of long chain fatty acids (C14-36) with long chain (C16-30) alcohols - High melting points (60-100C) - Energy storage Phospholipids are the major lipid constituents of cellular membranes and occur in high concentrations in the lipids of blood plasma, egg yolk, and in the seeds legumes. They comprise about 40% of the lipids in the erythrocyte membrane and over 95 % of the lipids in the inner mitochondrial membrane. About 20% of the lipids of the inner mitochondrial membrane are cardiolipin, a phosphoglyceride. They contain phosphorous and have a backbone of glycerol (e.g., phosphoglycerides) or sphingonise (e.g., sphingomyelin). L-Glycerol-3-phosphate, the backbone of phospholipids 64 Classification of phosphoglycerides. Phosphatidylcholine (lecithin) High amount of circulation PL (69%) A PL that is used in plasma membrane The most common PL in the membrane PLC is a store of Choline in the body Dipalmitoyl Lecithin is a surfactant Functions: Choline is required for the proper metabolism of fats; Facilitates the movement of fats in and out of cells. In liver, it exports the fat from the hepatocytes (Choline deficiency=Fat accumulation, fatty liver, cirrhosis) 65 Like Vitamin B12, 5-adenosylmethionine, and Folic Acid, choline acts in the human body as a methyl donor. Choline is needed for cell membrane integrity because of the critical role it plays in the manufacture of primary components of cell membranes, such as phosphatidylcholine and sphingomyelin. Choline is essential in the synthesis of acetylcholine. Choline supplementation increases the accumulation of acetylcholine which plays a crucial role in many brain processes, including memory. Phosphatidylcholine increases the solubility of cholesterol and thereby decreases cholesterol‗s ability to induce atherosclerosis. Phosphatidyl Ethanolamine (Cephalin) 5% of circulation PL Isolated from Brain Phosphatidylethanolamine is the second most abundant phospholipid in animal and plant lipids and is the main lipid component of microbial membranes. It can amount to 20% of liver phospholipids and as much as 45% of those of brain Functions: secretion of the nascent very-low-density lipoproteins from liver membrane fusion and fission Integration of plasma membrane Cardiolipin (bisphosphatidyl glycerol) Phosphatidyl Glycerol + Phosphatidic Acid Inner mitochondrial membrane, where it constitutes about 20% of the total lipid composition. Is seen in mitochondria Functions Regulates aggregate structures Helps to build quaternary structure Triggers apoptosis Serves as proton trap for oxidative phosphorylation Cholesterol translocation from outer to the inner membrane of mitochondrial Import protein into mitochondrial Ether Lipids (Plasmalogen [phosphatidal]& Platelete activating factor [PAF]) Similar to Lecithin, except that instead of ester link, they have ether link Plasmalogen has unsaturated Acyl group and PAF has saturated 66 Plasmalogen (Phosphatidal) The heart of vertebrates is rich of ether lipids The importance is unknown, it seems that these ether link protected phospholipids from phospholipase activity PAF Released from Basophiles and stimulated platelet to secrete Serotonin Phospholipases PLA1: phospholipase A1 PLA2: phospholipase A2 PLC: phospholipase C IP3 :inositol-1,4,5-trisphosphate DAG: diacylglycerol PLD: phospholipase D PLA2 produce 1. Lysolecithin (emulicification of triglycerides at high concentration 2. Arachidonic acid (the precursor of eicosanoids (Prostaglandines, Leukoterines, Tromboxane, Prostacycline)) The Poisonous Snakes contain phospholipase A2 that breakdown Lecithin and produces Lysolecithin Lysolecithin acts a detergent and dissolves the membrane of red blood cells. PLC: produces the second messengers (DAG & IP3) DAG: Activation of protein kinase C and phosphorylation of target proteins IP3 Release of Ca from endotelium And regulating activation of proteins Sphingolipids are present in nearly all human tissues. The greatest concentration is found in CNS, particularly in white matter. Sphingosine is 18C amino alcohol that forms the backbone of sphingolipids (rather than glycerol); Sphingosines are important components of biological membranes; Ceramide = sphingosine + fatty acid (via an amide linkage); Sphingomyelins = ceramide + phospholipids (via 1-hydroxyl group) Glycosphingolipids = ceramide + β-linked sugar at the 1-hydroxyl moiety 67 A. Sphingomyelin Is the major phospholipid component of membrances in neural tissues. They are the only sphingolipid that contain phosphate and have no sugar moiety. B.Glycosphingolipids. At least four classes of glycosphingolipids have been distinguished: cerebrosides, sulfatides, globosides, and gangliosides. 1. Cerebrosides are ceramide monohexosides, Ceramide + Glucose= Glucocerebroside (found in RBC, WBC, Membrane) Ceramide + Galactose= Galactocerebroside (in Brain, particularly the myelin sheath.) 2. Sulfatides. β-Sulfogalactocerebroside, a major brain sulfolipid, accounts for about 15% of the lipids in white matter. 3. Globosides are ceramide oligosaccharides; they contain two or more sugar molecules, most often galactose, glucose, or N-acetylgalactosamine, attached to ceramide. Globosides are found in serum, the spleen, the liver, and erythrocytes. 68 4.Gangliosides are Ceramide + 3 (or more) sugars including one sialic acid. They are found in high concentration in ganglion cells of the CNS and in lower concentration in the membranes of most cells. They have limited abundance; key tissue specific signaling molecule. They determine blood type: O, A and B antigens that give rise to blood types are gangliosides. The polar ―head groups‖ of these gangliosides are different Immune system of a person early in development "learns" to recognize oligosaccharides on their own glycoproteins and glycolipids as "self", and doesn't make antibodies to those. Antigenic determinants (epitopes) that are LACKING on person's own glycoproteins and glycolipids are treated as "foreign", and if immune system encounters those it makes antibodies against the "non-self" antigens. Thus a blood transfusion containing "non-self" antigens causes an immune response, rejection of the "foreign" blood. Look at the structures of the oligosaccharide blood group antigens to the left -- Why would individuals who are type AB (have both type A and type B oligosaccharides themselves) be called "universal acceptors", able to accept blood transfusions of ANY blood type? Why would type O individuals be called "universal donors", able to donate blood to people of any blood type (A, B, AB, or O)? 69 Eicosanoids Eicosanoids are oxygenated derivates of polyunsaturated 20-carbon fatty acids Most cells, except red blood cells, produce eicosanoids, Have profound physiological effects at extremely low concentrations. They have specific effects on target cells close to their site of formation. They are rapidly degraded, so they are not transported to distal sites within the body. Physiological functions of eicosanoids Eicosanoid Functions prostaglandins inflammation, fever production, prevent platelet aggregation and induce labor thromboxanes produced by platelets to promote their aggregation (blood clotting) leukotrienes allergic reactions NSAIDs (Non steroid anti-inflammatory drugs) Acetylsalicylic acid and related NSAIDs selectively inhibit the cyclooxygenase activity of prostaglandin synthase and consequently the synthesis of most eicosanoids. Aspirin acetylates a serine hydroxyl group near the active site, preventing arachidonate binding. The inhibition by aspirin is irreversible 70 Most other NSAIDs, such as indomethacin and ibuprofen, inhibit cyclooxygenases by competing with arachidonate. Side effects of NSAIDs+ Impairing hemostasis because of inhibiting synthesis of thromboxanes by thrombocytes Increasing HCl secretion and inhibiting the formation of protective mucus. Long-term NSAID use damages the gastric mucosa. Selective COX inhibitors Celecoxib Rofecoxib Because COX-2 is usually specific to inflamed tissue, there is much less gastric irritation associated with COX-2 inhibitors, with a decreased risk of peptic ulceration. A. Effects of Prostaglandins 1.Stimulate or inhibit smooth-muscle contraction 2. Inhibit HCl secretion in the stomach, particularly PGE1 3. Promote mucus secretion, which protects the gastric mucosa against the acid. 4. In the immune system, prostaglandins attract leukocytes to the site of infection 5. PGF2a – causes constriction of the uterus 6. PGE2 is applied locally to help induce labor at term 7.Inhibit Platelet aggregation 8.Sodium and water retention by kidney tubules B. Effects of Thromboxanes (TX) 1.Thromboxane A2 (TXA2) is produced by platelets; it causes contraction of arteries and triggers platelet aggregation. 2.These effects are exactly the opposite of those caused by prostacyclin (PCI2), which is produced by the endothelial cells of the vascular system. 3.TXA3 and PGI2 are antagonistic and have a balanced working relationship. 4.Diet rich in Fish oil containing w-3 fatty acids produce PGI3 & TXA3. Whereas, (PGI3 > PGI2 as antiaggregator) & (TXA3 < TXA3 as platelet aggregator) D. Effects of Leukotrienes 1.Leukotrienes are involved in chemotaxis, inflammation, and allergic reactions. 2.Leukotriene D4 (LTD4) has been identified as the slow-reacting substance anaphylaxis (SRS-A), which causes smooth muscle contraction more potent than histamine in constricting the pulmonary airways. SRS-A increases fluid leakage from small blood vessels and constricts coronary arteries 3.Leukotriene B4 (LTB4) attracts neutrophils and eosinophils, which are found in numbers at sites of inflammation. Steroids Are lipids that contain four fused carbon rings that form the steroid nuclei cyclopentanoperhydrophenanthrene. Cholesterol is the most common steroid in animals and precursor for all other steroids in animals; Steroid hormones serve many functions in animals - including salt balance, metabolic function and sexual function; 71 Steroid hormones carry messages between tissues Derivates of sterols Sex hormones and hormones from adrenal cortex Play important roles in gene expression Prednisolone and prednisone are steroid drug with potent antiinflammatory activities. 72 DIGESTION, ABSORPTION, SECRETION, AND UTILIZATION OF DIETARY LIPIDS An adult ingests about 60 to 150 g of lipids per day, of which more than 90 % is normally triacylglycerol (TAG). The remainder of the dietary lipids consists primarily of cholesterol, cholesteryl esters, phospholipids, and unesterified ("free") fatty acids. in the stomach, acid stable lingual and gastric lipases digest TAG, particularly those containing fatty acids of short- or medium-chain length (< 12 carbons, such as are found in milk fat), Emulsification of dietary lipids occurs by the bile salts, and mechanical mixing due to peristalsis. The dietary triacylglycerol, cholesteryl esters, and phospholipids are enzymatically degraded ("digested") by pancreatic enzymes, whose secretion is hormonally controlled Triacylglycerol degradation: pancreatic lipase preferentially removes the fatty acids at carbons 1 and 3. The primary products of hydrolysis are thus a mixture of 2- monoacylglycerol and free fatty acids Cholesteryl ester degradation: pancreatic cholesterol esterase, produces cholesterol plus free fatty acids Phospholipid degradation: Pancreatic Phospholipase A2 removes one fatty acid from carbon 2 of a phospholipid, leaving a lysophospholipid. The remaining fatty acid at carbon 1 can be removed by lysophospholipase, leaving a glycerylphosphoryl base Control of lipid digestion: 1.Cholecystokinin: Cells in the mucosa of the jejunum and lower duodenum produce a small peptide hormone, cholecystokinin (CCK) in response to the presence of lipids and partially digested proteins CCK acts on the gallbladder (causing it to contract and release bile), and on the exocrine cells of the pancreas (causing them to release digestive enzymes). 2.Secretin: in response to the low PH of the chyme entering the intestine. Secretin causes the pancreas and the liver to release a watery solution rich in bicarbonate that helps neutralize the PH of the intestinal contents, bringing them to the appropriate pH for digestive activity by pancreatic enzymes. Absorption of lipids by intestinal mucosal cells (enterocytes) Free fatty acids, free cholesterol, and 2-monoacylglycerols are the primary products of dietary lipid degradation in the jejunum. These, together with bile salts, form mixed micelles Short- and medium-chain length fatty acids do not require the assistance of mixed micelles for absorption by the intestinal mucosa. The mixture of lipids absorbed by the enterocytes migrates to the endoplasmic reticulum where biosynthesis of complex lipids takes 73 Lipid Transport & Storage Fat absorbed from the diet and lipids synthesized by the liver and adipose tissue must be transported between the various tissues and organs for utilization and storage. Since lipids are insoluble in water, so it is transported by formation of water miscible lipoproteins. Plasma lipids consist of triacylglycerols (16%), phospholipids (30%), cholesterol (14%), and cholesteryl esters (36%) and a much smaller fraction of unesterified long-chain fatty acids (4%). This latter fraction, the free fatty acids (FFA), is metabolically the most active of the plasma lipids. Because fat is less dense than water, the density of a lipoprotein decreases as the proportion of lipid to protein increases (Table 3). In addition to FFA, four major groups of lipoproteins have been identified. These are (1) Chylomicrons (CM), derived from intestinal absorption of triacylglycerol and other lipids (2) Very low density lipoproteins (VLDL, or pre-β-lipoproteins), derived from the liver for the export of triacylglycerol (3) Low-density lipoproteins (LDL, or β-lipoproteins), representing a final stage in the catabolism of VLDL (4) High-density lipoproteins (HDL, or α-lipoproteins), involved in VLDL and chylomicron metabolism and also in cholesterol transport. 74 Table: 3 Composition of the lipoproteins in plasma of humans. Lipoprotein Source Main Lipid Components Apolipoprotein CM Intestine Triacylglycerol A, B-48, C, E Chylomicron Triacylglycerol, CM B-48, E remnants phospholipids, cholesterol VLDL Liver Triacylglycerol B-100, C, E IDL VLDL Triacylglycerol, cholesterol B-100, E LDL VLDL Cholesterol B-100 Liver, intestine, HDL VLDL, phospholipids, Cholesterol A, C, E chylomicrons Albumin/free Adipose tissue Free fatty acids fatty acids Table: 4 function of apoproteins Apoprotein Function Activates LCAT A-1 Structural in HDL Receptor binding B-100 Structural in LDL & VLDL B-48 Structural in chylomicrons