Nutrition, Metabolism, and Energy Balance PDF
Document Details
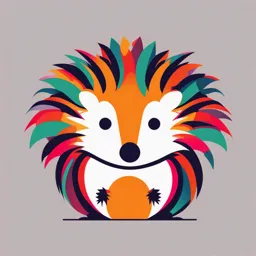
Uploaded by EfficientFreesia
Tags
Summary
This document discusses the process of glycolysis, a crucial metabolic pathway for energy production. It details the three stages of glycolysis and the subsequent fate of pyruvic acid, highlighting the importance of oxygen availability. The document also connects glycolysis to other metabolic processes within the body.
Full Transcript
938 UNIT 4 Mai ntenance of the Body Phase 1. Suga r activa tion. In phase I, glucose is phosphor- ylated twice and converted to fructos...
938 UNIT 4 Mai ntenance of the Body Phase 1. Suga r activa tion. In phase I, glucose is phosphor- ylated twice and converted to fructose- 1,6-bisphosphate. These reactions use t\vo ATP rnolecules (to be recouped later), O Carbon atom which provide the activation energy needed to prime the later O Phosphate stages of the pathway. Phase I can be thought of as the energy invest,nent phase. Ph ase 2. Suga r cleavage. During phase 2, fructose- 1,6- bisphosphate is split into two 3-carbon fragments that exist Glucose (interconvertibly) as one of two isomers: glyceraldehyde ~ (glis"er-al 'de-hTd) 3-phosphate or dihydroxyacetone (di"h i- Phase 1 drok''se-as'e-ton) phosphate. Sugar activation: Phase 3. Sugar oxidation and ATP formation. In phase 3, two Phosphorylation major events happen. First, the two 3-carbon fragments are activates glucose. oxidized by the removal of hydrogen, which NAO picks up. Glucose is 2 ADP converted to In this way, some of glucose's energy is transferred to NAO. fructose-1, 6· Second, inorganic phosphate groups (Pi) are attached to each bisphosphate. 2 ATP molecules oxidized fragment by high-energy bonds. Later, when these Fructose-1,6· terminal phosphates are split off, e nough energy is captured are used. bisphosphate -~ to form four ATP molecules. As we noted earlier, fonnation of ATP this way is called substrate-level phosphorylation. Phase 2 The final products of glycolysis are two molecules of pyr uvic Sugar a cid , two molecules of reduced NAO+ (NADH), and two H. cleavage: There is a net gain of two ATP molecules per glucose molecule. Fructose- 1,6- bisphosphate Four ATPs are produced, but remember that two are consumed is split into in phase I to activate the reactions. Each pyruvic ac id molecule two 3-carbon has the formula C3 H4 0 3 , and glucose is C 6 H 120 6. Be tween fragments. Dihydroxyacetone....,,, Glyceraldehyde phosphate ~ 3 -phosphate them the two pyruvic acid molecules have lost four hydro- gen atoms, whose electrons are now bound to two molecules Phase3 of NAO. NAD carries a positive charge (NAO ), so when it Sugar oxidation accepts a hydrogen pair, NAOH + H is the resulting reduced and ATP fonnation: product. Although a small amount of ATP has been harvested, The 3-carbon fragments are the other two products of glucose ox idation (H2 0 and C0 2) oxidized (by __..._ 2 NADH + H+ have yet to appear. removing 4ATP The fate of pyruvic acid, which still contains most of glu- hydrogen) and 4 ATP molecules cose's chemical energy, depends o n the availabil ity of oxygen are formed. 2 Pyruvic acid at the time the pyruvic acid is produced. Because the supply of QIN NAD is lim ited, glycolysis can continue o nly if the reduced 2 NADH + H+ coenzymes (NADH, together with its H+) formed during glycol- ysis are relieved of their extra hydrogens. Only then can they continue to act as hydrogen acceptors. 2 NAD+ When oxygen is readily available, this is no problem. NAOH 2 Lactic acid + H+ delivers its burden of hydrogen atoms to the enzyrnes of To citric 000 the electron transport chain in the mitochondria, which deliver acid cycle (aerobic them to 0 2 , forming water. However, when oxygen is not pathway) present in sufficie nt amounts, as might occur during strenuous exercise, NADH + H+ unloads its hydrogen atoms back onto pyruvic acid, reducing it. This addition of two hydrogen atorns to pyruvic acid yields lactic a cid (see bottom right of Figure 24.6). Figure 24.6 The three major p hases of g lyco lysis. The fate of Some of this lactic acid diffuses out of the cells and is trans- pyruvic acid depends on whether or not molecular 0 2 is available. ported to the liver for processing. When oxygen is again available, lactic acid is oxidized back to pyruvic acid and enters the a erobic pa th,vays (the oxygen- The three maj or phases of glycolysis shown in Figure 24.6 requiring citric acid cycle and electron transport chain withi n the are described next. Appendix D shows the complete glycolytic mitochondria), and is completely oxidized to water and carbon pathway. dioxide. The liver may also convert lactic acid all the way back to glucose-6-phosphate (reverse glycolysis). Glucose-6-phosphate Chapter 24 Nutrition, Metabolism, and Energy Bala nce 939 can be either stored as glycogen or freed of its phosphate and Citric Acid Cycle released to the blood if blood sugar levels are low. The citric acid cycle (or Krebs cycle) is the next stage of glucose Except for red blood cells (which typically carry out only oxidation and is named for its first substrate. The citric acid glycolysis), prolonged anaerobic metabolism ultimately results cycle occurs in the mitochondrial matrix and is fueled largely in acid-base problems. Consequently, totally anaerobic condi- by pyruvic acid produced during glycolysis and by fatty acids tions resulting in lactic acid formation provide only a temporary resulting from fat breakdown. route for rapid ATP production. Totally anaerobic conditions Because pyruvic acid is a charged molecule, it must enter the can go on without tissue damage for the longest periods in skel- mitochondrion by active transport with the help of a transport etal muscle, for much shorter periods in cardiac muscle, and protein. Once in the mitochondrion, the first order of business is almost not at all in the brain. Although glycolysis generates ATP a transitional phase that converts pyruvic acid to acetyl CoA. rapidly, each glucose molecule yields only 2 ATP as compared This occurs via a three-step process (Figure 24.7, top): to the 30 to 32 ATP when a glucose molecule is completely oxidized. Figure 24.7 Simplified version of the citric acid (Krebs) cycle. Duri ng each turn of the cycle, two carbon atoms are removed from the substrates as (02 (decarboxylation reactions); four oxidations by removal of hydrogen atoms occur, producing four molecules of reduced coenzymes 9 Carbon atom b I I (3 NADH+ 3 H and 1 FADH2) ; and one ATP is synthesized by su strate- eve O Inorganic phosphate phosphorylation. An additional decarboxylation and an oxidation reaction occur in the transitional phase (at top) that converts pyruvic acid, h the @, Coenzyme A · · product of glycolysis, to acetyl CoA, the molecule that enters t e otnc acid cycle pathway. Cytosol Pyruvic acid from glycolysis ~ Mitochondrion (matrix) Transitiona l iJlco 2 phase ~ Acetyl CoA NJ Oxaloacetic acid c 1111 (!"11 ,llii.. ~ (pickup molecule) , Citric acid ~ (initial reactant) NADH + H ri......_ Malic acid lsocitric acid ~ ~ Citric acid cycle a·Ketoglutaric acid ~ Succinic acid ~ Succinyl-CoA FAD ~ GDP+ 0 ADP 940 UNIT 4 Mai ntenance of the Body 1. Decarboxylation. In this step, one of pyruvic acid's carbons Electron Transport Chain a nd Oxidative is removed a nd released as carbo n dioxide gas, a process Phosphorylat ion called d ecarboxylation. C02 diffuses out of the cells into Like glycolysis, none of the reactions of the citric acid cycle use the blood to be expelled by the lungs. This is the fi rst ti me oxygen di rectly. This is the exclusive function of the electron that C02 is released during cellular respiration. tra nsport chain, which carries out the final catabolic reactions 2. Oxidation. The remaining 2-carbon fragment is oxidized to that occur on the inner mitochondrial membra ne. However, acetic acid by removing hydrogen atoms, which are picked because the reduced coenzymes produced in the c itric acid up by NAD. cycle are the substrates for the e lectron transport chain, these 3. Fonnation of acetyl CoA. Acetic acid is combined with coen- two pathways are coupled, and both are aerobic, meaning they zy111e A to produce the reactive final product, acetyl coenzyme require oxygen. A (acetyl CoA). Coenzyme A is a sulfur-containing coenzyme In the electron transport chain, the hydrogens removed dur- derived from vita1nin B5. ing the ox idation of food fuels are combined with 0 2 to form Acetyl CoA is now ready to e nter the citric acid cycle and be water, a nd the energy released du ring those reactions is har- broken down completely by mitochondrial enzymes. Coenzyme nessed to attach Pi groups to ADP, form ing ATP. As we noted A shuttles the 2-carbon acetic acid to an e nzyme that joins it to earlier, this type of phosphorylation process is called oxidative a 4-carbon acid called oxaloacetic acid (ok"sah-lo"ah-set' ik) to phosphorylation. Let' s peek under the hood of a cell 's power produce the 6-carbon citric acid. plant and see how this rather complicated process works. As the cycle moves through its e ight successive steps, the Just as in a car, what we see is a complicated structure with atoms of ci tric acid are rearranged to produce different inter- many parts. Why are there so many parts? In a car e ngine, we mediate molecules, most called keto acids (Figure 24.7). The burn fuel for e nergy. If released all at once, that energy would acetic acid that e nters the cycle is broken apart carbon by car- result in a big, hot explosion. All of the complex engine parts bo n (decarboxylated) and ox idized, generating NADH + H+ make it poss ible to captu re some of this energy to do useful and FADH 2. At the e nd of the cycle, acetic acid has been work. In the same way, the electron transport chain harvests the totally disposed of and oxaloacetic acid, the pickup molecule, is energy of food fuels a bit at a time in a step-by-step way so we regenerated. can ultimately make ATP. For each turn of the cycle, we get: Most components of the electron transport chain are proteins that bind metal atoms (known as cofactors). These proteins vary l\vo C0 2 molecules that come from two decarboxylations. in composition and form multiprotein complexes that are firmly Four molecules of reduced coenzymes (3 NADH + 3 H+ and embedded in the inner nlitochondrial membrane as shown in Focus I FADH 2). The additio n of water at certain steps accounts on Oxidative Phosphorylation (Focus Figure 24.1). For exrunple, for some of the released hydrogen. some of the proteins, the flavins, contain flavin mononucleotide One molecule of ATP (via substrate-level phosphorylation). (FMN) derived from the vitamin riboflavin, ru1d others contain both sulfur (S) and iron (Fe). Most of these proteins, however, The detailed events of each of the eight steps of the citric acid are brightly colored iron-containing pigments called cytochromes cycle are described in Appendix D. (si' to-kromz; cyto = cell, chro,n = color), including complexes Now Jet's back up and account for the pyruvic acid mol- ill and IV depicted in Focus Figure 24. l. Neighboring carriers are ecules enteri ng the rn itochondria. We need to consider the prod- clustered together to form four respir atory euzy1ne co1nplexes ucts of both the transitional phase and the citric acid cycle itself. that are alternately reduced and oxidized as they pick up electrons Altogether, each pyruvic acid yields three C0 2 molecules and and pass them on to tl1e next complex in tl1e sequence. five molecules of reduced coenzymes-1 FADH2 and 4 NADH As Focus Figure 24. l shows, the first such complex accepts + 4 H+ (equal to removing 10 hydrogen atoms). The products hydrogen atoms from NADH + H , ox idizing it to NAD. of glucose oxidation in the citric acid cycle are tw ice that FADH 2 transfers its hydrogen atoms slightly farther along (remember I glucose = 2 pyruvic acids): six C0 2, ten molecules the chain to the small complex II. The hydrogen atoms that of reduced coenzymes, and two ATP molecules. the reduced coenzymes deliver to the electron transport chain Notice that it is these citric acid cycle reactions that pro- are quickly split into proto ns (H+) plus electrons (e- ). The duce the C02 released during glucose ox idation. The reduced electrons are shuttled along the inner mitochondrial membrane coenzymes, which carry their extra electrons in high-energy from one complex to the next, losing energy wi th each transfer. linkages, must now be oxidized if the citric acid cycle and gly- The protons escape into the watery matrix, only to be picked up colysis are to conti nue. and "pumped" across the inner mitocho ndrial membrane into Although glycolysis is exclusive to carbohydrate oxida- the intermembrane space by o ne of the three major respiratory tion, breakdown products of carbohydrates, fats, and proteins enzyme complexes (I, Ill, or IV). can feed into the citric acid cycle to be ox idized for energy. Ultimately two electrons are delivered to half a molecule of On the other hand, some citric acid cycle intermediates can be 0 2 (in other words, to an oxygen atom), creating oxygen ions siphoned off to make fatty acids and nonessential amino acids. (O") that strongly attract H+ a nd form water, as indicated by In this way, the citric acid cycle is a source of bui lding materials the reaction for anabolic reactions, as well as the final common pathway for oxidizing food fuels. Oxidative phosphorylation has two phases: Phase 1: The electron transport chain creates a proton (H+) gradient across the inner mitochondrial membrane using high- energy electrons (e- ) removed from food fuels. Phase 2: Chemiosmosis uses the energy of the proton gradient to synthesize ATP. Ell!C:trOn ttansport ) Cllai'I ancs o:idda1ille Gl~ i.s phOsj)t'IOr'ytalion Outer mitochondrial Phase 1: membrane Electron transport creates the proton gradient. Phase 2: Chemiosmosis uses.. l!JJI'-.., ·:ii:··....... the proton gradient to synthesize ATP. lntermembrane space.... + + Inner + + + + + + + mitochondrial + + + + + + + + + + + + + + + ++ + + + + + + + + membrane ++ (crista) + + + + I + + + ATP - - synthase V G) Reduced coenzymes @The electrons are transferred @ At respiratory enzyme © ATP synthase (compl ex V) (NADH + H+ and FA DH2) from one complex to another in complex IV, two electrons liarnesses t he energy of the deliver electrons to the membrane. combine with two protons proton gradient to synthesize respiratory enzyme Each complex is reduced and (H+) and a half molecule ATP. As H+ flows back across complexes I and II. then oxidized. of 0 2 , forming water. the membrane through ATP The energy released pumps H+ synthase, t he synthase rotor into the intermembrane space, spins, causing P; to attach creating an electrochemical to ADP, forming ATP. gradient between the matrix and the intermembrane space. Mitochondrial Coenzyme Q (ubiquinone) and matrix cytochrome c sh uttle electro ns between the larger complexes. 941 942 UNIT 4 Maintenance of the Body Virtually all the water resulting from glucose oxidation is progressively lower energy levels until they are finally delivered formed during ox idative phosphorylation. Because NADH + H+ to oxygen, which has the greatest affinity of all for electrons. and FADH2 are oxidized as they release their cargo of hydrogen You could say that oxygen "pulls" the electrons down the chain atorns, the net reaction for the electron transport chain is (Figure 24.8). The electron transport chain functions as an energy converter Coenzyme-2H + !02 -+ coenzyme + H20 by usi ng the stepwise release of electronic energy to pump pro- reduced oxid ized coenzyme coenzyme tons from the rnatrix into the intermembrane space. Because the Each successive carrier has a greater affi nity (b ind ing inner mitochondrial membrane is nearly impermeable to H , strength, or "pull") for electrons than those preceding it. For this this chemiosmotic process creates an electrochemical proton reason, the electrons cascade "downh ill" frorn NADH + H+ to (H ) gradien t across that membrane, a gradient that has poten - tial energy and the capaci ty to do work.......... ttansport el'lain a"(!" Oltiealive pl'IOsp"Or aliotl NADH + H+ 50 FADH2 2 e- 40 - 0 ~ B.. :, 30 0 g., 2: 1ii !!! l;, 20 t.,c ~ u. Energy lost as heat 2~ 10 (originally from NADH or FADH2 ) 0 Figure 24.8 Energy is harvested at each step in the elect ron transport chain. The electron transport chain takes t he large overall energy change between NADH + H or FADH2 (from food fuels) and oxygen and harvests smaller, more manageable amounts of energy at each step. Chapt er 24 Nutrition, Metabolism, a nd Energy Bala nce 943 The proton gradient: Creates a pH gradient, with the H+ concentration in the matrix much lower than that in the intermembrane space Generates a voltage across the membrane that is negative on the matrix side and positive between the mitochondrial mernbranes Both co nditions strongly attract H+ back into the matrix. But how can they get there? The only areas of the inner mitochondrial membrane freely permeable to H+ are large e nzyme-protein complexes (complex V) called ATP synth ases (Figure 24.9). These complexes Jay claim to being nature 's smallest rotary motors. Each of these motors drives a molecular mill that fuses AD P and P; together into ATP. Ho\v this molecular complex works is shown in Figure 24.10. Notice someth ing here: The ATP synthase works like an ion pump running in reverse. Recall from Chapter 3 ('4 p. 75) that ion pumps use ATP as their energy source to transport ions against an electrochemical gradient. Here \Ve have AT P synthases using the energy of a proton gradient to power ATP synthesis. The proton gradient also suppl ies energy to pump needed Figure 24.9 Atomic force microscopy reveals the structure metaboli tes (ADP, pyruvic acid, inorganic phosphate) and cal- of energy-converting ATP synthase rotor rings. ciu m ions across the relatively impermeable inner mitochon- drial membrane. The supply of e nergy from ox idation is not limitless, however, so when more of the gradie nt e nergy is used to drive these transport processes, Jess is available to make ATP. lntermembrane space (!) Protons (H+) flow down their.J HOMEOSTATIC ~--alt!""'- IMBALANCE 24.1 CLINICAi! electrochemical gradient through Studies of metabolic poisons support the chemiosmotic model of the stationary st ator. oxidative phosphorylation. For example, cyanide (the gas used in gas chambers) disrupts oxidative phosphorylation by binding to @ Each H+ binds cytochrome oxidase and blocking electron flow from cornplex rv + + + + + + + + to a subunit of the to oxygen (see Figure 24.8). Poisons called "uncouplers" abolish rotor, causing it to the proton gradient by making the inner mitochondrial membrane change shape and making the rotor permeable to H. Consequently, although the electron transport spin. chain continues to deliver electrons to oxygen at a furious pace and oxygen consumption rises, no ATP is made. @rhe spinning ---li!--- ---1-l rotor turns the connecting rod. Su mmary of ATP Prod uction The average perso n at rest uses e nergy at the rate of roughly @ As the rod spins, it activates catalytic LOO kcal/hour, \vhich is equal to 116 \vatts, or sl ightly more sites in the knob than an oJd.fashioned lightbulb. This may seem a tiny amount, that join P; to but from a biochernical standpoint it places a staggering power ADP to make ATP. demand on our mitochondria. Luckily, they are up to the task. ADP When 0 2 is present, cellular respiration is rernarkably efficient. + Of the 686 kcal of energy present in I mole of glucose, as much as 262 kcal can be captured in ATP bonds. (The rest is liberated as heat.) This corresponds to an e nergy capture of about 38o/o, Mitochondrial matrix making cells far more efficient than any human-made machines, which capture only l 0-30o/o of the energy available to them. Figure 24.10 Structure a nd function of ATP synthase. During cellular respiration, most energy flows in this sequence: Glucose ~ NADH + H+ ~ electron transport chain ~ proton gradient energy ~ ATP 944 UNIT 4 Mai ntenance of the Body Cytosol Glycolysis - Electron transport chain and oxidative Glucose =::ti> : ;;~ vie phosphorylation - (4 ATP - 2 ATP -1 O NADH + H+ x 2.5 ATP used for activation energy) Net +2 ATP + 2ATP + about 28 ATP by substrate-level by substrate-level by oxidative phosphorylation phosphorylation phosphorylation -2 ATP (average shuttle cost) Typical ATP yield per glucose Figure 24.1 1 Energy yield during cellular respiration. Let's do a li ttle bookkeeping to summarize the net energy So, if we deduct 2 ATP to cover the average "fare" for the gain from one glucose molecule (Figure 24.11). shuttle, our bookkeeping comes up wi th a grand total of 30 ATP 1. Substrate-level phosphorylation. Subs trate-level phos- per glucose as the typical energy yield. (Actual ly our figures phorylation gives us a net gain of 4 ATP (2 during glycolysis are probably sti ll too high because the proton gradient energy is and 2 during the citric acid cycle). also used to do other work and so the electron transport chain 2. Oxidative phosphorylation. NADH + H+ and FADH2 feed- cannot recover all of the energy in a glucose molecule.) ing into oxidative phosphorylation give us about 28 ATP. For each NADH + H+, the proto n gradient generates Glycogenesis, Glycogenolysis, and abou t 2'h ATP molecules. The 2 NADH + H+ generated Gluconeogenesis during glycolysis yield 5 ATP. The 8 NADH + H+ produced Although most glucose is used to generate ATP molecules, un- during the transit ion reaction and citric acid cycle generate limi ted amounts of glucose do not result in unl irnited ATP syn- 20ATP. thesis, because cells can not store large amounts of ATP. Aside The oxidatio n of FA DH 2 is Jess efficient because it from cellular respiration, the goal of carbohydrate metabol ism doesn't donate electrons to the "top" of the electron trans- is to make sure that just the right amount of glucose is present port chain as does NADH + H+, but to a lower energy level in the blood. Three processes with similar soundi ng names are (at complex Il). Each FADH 2 generates only about IV2 ATP, required. Figure 24.12 wi ll help you keep them straight. and so the 2 FADH2 from the citric acid cycle are "worth" a total of 3 ATP. Overall, complete ox idation of l glucose molecule to C02 and H2 0 by both substrate-level phosphorylation and oxidative phosphorylation yields a maximum of 32 molecules of ATP. However, there is uncertainty about how much ATP is generated from the NADH + H+ that comes from glycolysis that occurs outside the mitochondria. The inner mitochondrial membrane is Glycolysis: Converts glucose to pyruvic acid not permeable to reduced NADH, so NADH + H+ formed dur- Glycogenesis: Polymerizes glucose to form glycogen ing glycolysis uses a shuttle nu>lecule to deliver its electron pair Glycogenolysis: Hydrolyzes glycogen to glucose monomers to the electron transport chai n. Some shuttles (for example, the Gluconeogenesis: Forms glucose from noncarbohydrate precursors glycerol phosphate shuttle) have an energy cost of l ATP per NADH, whereas other shuttles seem to provide a "free ride." Figure 24.12 Quick summary of carbohydrate reactions. Chapt er 24 Nutrition, Metabolism, a nd Energy Balance 945 converted to glucose-6-phosphate, a form that can enter the gly- Glycogenesis Glycogenolysis colysis pathway to be oxidized for energy. Synthesizes glycogen Breaks down glycogen to In muscle cells and most other cells, the glucose-6-phosphate from glucose release glucose resulting frorn glycogenolysis is trapped because it cannot cross Occurs when glucose Stimulated by low blood supplies exceed demand glucose the cell membrane. However, hepatocytes (and some kidney for ATP and intestinal cells) contain glucose-6-phosphatase, an e nzyme Cell... ·-·- --- exterior Blood glucose that removes the terminal phosphate, producing free glucose. Because glucose can then readily diffuse from the cell into the blood, the liver can use its glycogen stores to provide blood sugar for other organs when blood glucose levels drop. Liver glycogen is also an important energy source for skeletal muscles Hexokinase that have depleted their own glycogen reserves. (present in Glucose-6-phosphatase all cells) Gluconeogenesis 0 (present in liver, kidney, and intestinal cells) When too little glucose is available to stoke the " metabolic fur- ~ ADP..-,;1, - - - --' nace," glycerol and amino acids are converted to glucose. Glu- W Glucose-6-phosphate coneogenesis, the process of forming new (neo) glucose from noncarbohydrate molecules, occurs in the liver. Glucose-1 -phosphate Gluconeogenesis takes place whe n dietary sources and glu - cose reserves have been used up and blood glucose levels are begi nning to drop. Gluconeogenesis protects the body, espe- Glycogen cially the nervous system, from the damaging effects of low Glycogen phosphorylase blood sugar (hypoglyce,nia) by e nsuring that ATP synthesis can synthase conti nue. ~ Glycogen Cell interior.:. Check Your Understanding 12. What happens in glycolysis if oxygen and pyruvic acid are absent and NADH + H cannot transfer its cargo of hydrogen Figure 24.13 Glycogenesis and glycogenolysis. to pyruvic acid? 13. What two major kinds of chemical reactions occu r in the citric acid cycle, and how are these reactions indicated symbolically? 14. What name is given to the chemical reaction in wh ich When more glucose is available than can immediately be glycogen is broken down to its glucose subunits? oxidized, ris ing intracellular ATP concentrations eventuall y 15. j,);M In the drawing of a cell and mitochondrion below, label inhibit glucose catabolism and cause glucose to be stored as the inner mitochondrial membra ne, the outer mitochondrial glycogen or fat. Because the body can store much more fat than membra ne, the intermembrane space, and the matrix of glycogen, fats account for 80-85o/o of stored energy. the mitochondrion. Point to a location where each of the following processes takes place: glycolysis, citric acid cycle, Glycogenesis oxidative phosphorylation. Identify the region where you When high ATP levels begin to "turn off'' glycolysis, glucose would fi nd the lowest pH. molecules are combined in Jong chains to form glycogen, the animal carbohydrate storage product. This process is called glycogenesis (glyco = sugar; genesis = origin) (Figure 24.13, left side). Glycogenesis begins as glucose e ntering cells is phosphory- lated to glucose-6-phosphate and then converted to its isomer, glucose-1-phosphate. The terminal (last) phosphate group is split off as the enzyme glycogen synthase catalyzes the attachment of glucose to the growing glycogen chai n. Liver and skeletal muscle cells are most active in glycogen synthesis and storage. Glycogenolysis On the other hand, when blood glucose levels drop, glycogen lysis (splitti ng) occurs. This process is known as glycogenoly- sis (gli"ko-j e-nol'i-sis) (Figure 24. 13, right side). The e nzyme glycogen phosphorylase oversees phosphorylation and split- - - - - - - - - - - For answers, seeAnswers Appendix. ting of glycogen to release glucose- I-phosphate, which is then 946 UNIT 4 Maintenance of the Body Lipid metabolism is key for Triglycerides long-term energy storage and release -- Lipase Learning Outcomes II- Descri be the process by which fatty acids are oxidized for energy. - '' II- Define ket one bodies, and indicat e t he stimu lus for their Glycerol Fatty acids f ormation. Fats are the body's most concentrated source of e nergy. They contain very little water, and the e nergy yield from fat catab- olism is more than twice that from either glucose or protein ', catabol ism-9 kcal per gram of fat versus 4 kcal per gram of Glyceraldehyde carbohydrate or protein. Most products of fat digestion are 3-phosphate (a glycolysis intermediate) transported in lymph in the form of fatty-protein droplets called chylomicrons (see Chapter 23). Eventuall y, enzymes on capil- lary endothelium hydrolyze the lipids in the chylomicrons, and Glycolysis ,. - - - FAD the resulti ng fatty acids and glycerol are taken up by body cells and processed in various ways. Figure 24.14 summarizes the c Pyruvic acid c key metabolic reactions for lipids. 0 Enzyme "'~ Oxidation of Glycerol and Fatty Acids snips off ·g 2C fragments S Of the various lipids, only triglycerides(... p. 45) are routinely oxidized for energy. Their catabolism involves the separate ox i- ! Acetyl CoA dation of their two different building blocks: glycerol and fatty acid chains (Figure 24.15). Most body cells easily convert glycerol to glyceraldehyde 3-phosphate (a glycolysis interrnediate) and eventually to acetyl CoA, which e nters the citric acid cycle. Glyceraldehyde is equal to half a glucose molecule, and so the ATP e nergy harvest from its complete oxidation is approximately half that of glucose ( 15 ATP/glycerol). Beta oxida tion, the initial phase of fatty acid oxidat ion, occurs in the mitochondria. The net result is that the fatty acid Figure 24.15 lipid oxidation. chains are broken apart into two-carbon acetic acid fragments, and coenzymes (FAD and NAD+) are reduced (Figure 24.15, right side). Each acetic acid molecule is fused to coenzyme A, Lipogenesis formi ng acetyl CoA. The term "beta oxidation" reflects the fact that the carbon in the beta (third) position is oxidized each time There is a co ntinuous turnover of triglycerides in adipose tis- sue. New fats are stored for later use, and stored fats are bro- a two-carbo n fragment is broken off. Acetyl CoA then enters ken dow n and released to the blood. That bulge of fatty tissue the citric acid cycle where it is oxidized to C02 and H2 0. you see today does ,wt contai n the same fat molecules it d id a month ago. Glycerol and fatty acids from dietary fats not immediately needed for energy are recombined into triglycerides and stored. About 50% ends up in subcutaneous tissue, and the balance is stockpiled in other fat depots of the body. Triglyceride synthesis, or lipogenesis, occurs when cellu- lar ATP and glucose levels are high (Figure 24.16, magenta Beta oxidation: Converts fatty acids to aoetyl CoA arrows). Excess ATP leads to an accumulation of acetyl CoA Lipolysis: Breaks down lipids to fatty acids and glycerol and glyceraldehyde 3-phosphate, l\vo intermediates of glucose Lipogenesis: Forms lipids from acetyl CoA and metabolism that would otherwise feed into the citric acid cycle. glyceraldehyde 3-phosphate When these two metabolites are present in excess, they are channeled into triglyceride synthesis pathways. Figure 24.14 Quick summary of lipid reactions. Acetyl CoA molecules are joined together, form ing fatty acid chains that grow two carbons at a time. (This accounts for Chapt er 24 Nutrit ion, Metabolism, and Energy Balance 947 Glycolysis Glucose Stored fats in adipose tissue.......... Triglycerides Glycerol Lipogenesis !l Glyceraldehyde 3-phosphate Dietary fats / Fatty acids it Pyruvic acid Certain P o~ictq am ino acids Ketone Ketogenesis (in liver) fio" 1 Acetyl CoA bodies Steroids ~ iI __E _l_ ect _ro_n_ _. ~ ? Bile salts ~ 1---"""-,-1! Cholesterol. t ransport ~ chain + --till~ Catabolic reactions C02 + H20 --till~ Anabolic reactions Figure 24.16 Lipid met abolism. When needed for energy, fats enter catabolic pat hways (lipolysis). Excessive amounts of carbohydrates and amino acids are converted to triglycerides (lipogenesis) and stored. the fact that almost all fatty acids in the body contain an even Via a process called ketogenesis, the liver converts acetyl number of carbon atoms.) Because acetyl CoA, an intermediate CoA molecules to ke tone bodies, or ketones, which are in glucose catabolism, is also the starting point for fatty acid released into the blood. Ketone bod ies include acetoacetic acid, synthesis, glucose is easily converted to fat. Glyceraldehyde 13-hydroxybutyric ac id, and acetone. (The keto acids cycling 3-phosphate is converted to glycerol, which is joined with fatty through the citric acid cycle and the ketone bodies resulting from acids to form triglycerides. Consequently, even with a low-fat fat metabolism are qu ite different and should not be confused.) diet, carbohydrate intake can provide all the raw ,naterials needed to make triglycerides. When blood sugar is high, lipo- , HOMEOSTATIC genesis is the maj or activi ty in adipose tissues and is also an ~-""A,r-- IMBALANCE 24.2 important liver fu nction. When ketone bodies accumulate in the blood, ketosis results and large amou nts of ketone bodies are excreted in the urine. Lipolysis Ketosis is a common consequence of starvatio n and diabetes L ipolysis (JI-pol 'T-sis; "fat splitting"), the breakdown of stored mellitus, and also occurs during carbohydrate restriction. fats into glycerol and fatty acids, is essentially lipogenesis in re- Because most ketone bodies are organic acids, ketosis leads verse (Figure 24.16, blue arrows). The fatty acids and glycerol to 111etabo/ic acidosis. The body's buffer systems cannot tie up are released to the blood, helping to ensure that body organs the acids (ketones) fast enough, and blood pH drops to danger- have conti nuous access to fat fuels for aerobic respiration. (The ously low levels. T he person's breath smells fruity as acetone liver, cardiac muscle, and resting skeletal muscles actually pre- vaporizes from the lu ngs, and breathing becomes more rapid fer fatty acids as an energy fuel.) as the respiratory system tries to reduce blood carbonic acid by T he adage "Fats burn in the flame of carbohydrates" blowing off C02 to force the blood pH up. In severe untreated becomes clear when carbohydrate intake is inadequate. Under cases, the person may become comatose or even die as the acid such conditions, lipolysis accelerates as the body attempts to pH depresses the nervous system. fill the fuel gap with fats. However, the ability of acetyl CoA to enter the citric acid cycle depends on having sufficient car- bohydrate intermediate molecules (see Figure 24.7). When Synthesis of Structural Materials carbohydrates are deficient, these intermediates are converted All body cells use phospholipids and cholesterol to build their to glucose (to fuel the brain). Without them, fat oxidation is membranes. Phospholipids are important components of the incomplete, and acetyl CoA accumulates. myelin sheaths of neurons, and the ovaries, testes, and adrenal 948 UNIT 4 Mai ntenance of the Body cortex use cholesterol to synthesize their steroid hormones. In addit ion, the liver: Amino acids are used to build Synthesizes lipoproteins to transport cholesterol, fats, and proteins or for energy other substances in the blood (see pp. 956-957) Learning Outcomes Synthesizes cholesterol from acetyl CoA.... Describe how amino acids are metabolized f or energy. Uses cholesterol to form bile salts ("4 p. 897).... Describe the need f or p rotei n synthesis in bod y cells. Like all other biolog ical molecules, prote ins have a lim ited Check Your Understanding life span and must be broken down and replaced before they 16. Which part of triglyceride molecules enters glycolysis? deteriorate. As proteins are broken down, their amino acids are 17. What is the central molecule in fat metabolism? recycled and used to build new proteins or modified to form a 18. What are the products of beta oxidation? different N-containing compound. Cells actively take up newly -==-------__.. For answers, see Answers Appendix. ingested amino acids from the blood and use them to replace tissue proteins at the rate of about 100 grams each day. Althoug h popular opin ion has it that excess prote in can be stored by the body, nothi ng is farther from the truth. When more protein is available than is needed for anabolic purposes, G) Transaminat ion: An amine group is switched from an amino acid to a keto acid. Transamination Amino acid + Keto aci d - -+ + Keto acid + Amino acid @ oxidat ive (a·keto- (glutamic acid) deaminatio n: The amine glutaric acid) group of glutamic acid is Liver removed as ammonia Oxidative deaminatio n and combined w ith C02 NH 3 (ammonia) to form urea. Keto acid modificatio n @ Keto acid modification: Urea The keto acids formed during transamination are altered so Modified keto acid they can easily enter t he cit ric acid cycle. Blood Enter citric acid cycle in body oells Urea Excreted in urine Figure 24.17 Processes that o ccu r when amino acids are ut ilized f or energy.