TH Lecture Notes on Nutrition and Energy Expenditure (PDF)
Document Details
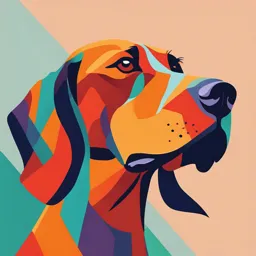
Uploaded by WellEstablishedStarfish
TH
Tags
Summary
These lecture notes detail nutrition and energy expenditure, examining the fed and fasting states, carbohydrate, protein, and fat metabolism. The notes also cover topics like ATP generation, glycolysis, and the role of glucose transporters. Key terms like glycogen, gluconeogenesis, and beta-oxidation are discussed in the context of energy regulation.
Full Transcript
TH lecture 1 nutrition and energy expenditure ============================================= Introduction - - - - - - - - 1. Fed State (Absorptive state): - - Fast State (Post-absorptive state): - - 2. Fed state - Carbs - Broken down into glucose, stor...
TH lecture 1 nutrition and energy expenditure ============================================= Introduction - - - - - - - - 1. Fed State (Absorptive state): - - Fast State (Post-absorptive state): - - 2. Fed state - Carbs - Broken down into glucose, stored as glycogen in liver and muscles, or converted to fat for storage in adipose tissue. Proteins - Broken down into glucose, stored as glycogen in liver and muscles, or converted to fat for storage in adipose tissue. Fats - Stored as triglycerides in adipose tissue. Fasting state - Carb - Liver glycogen is broken down into glucose for energy; gluconeogenesis produces glucose from amino acids and fats. Protein - Proteins broken down into amino acids to be used for gluconeogenesis or energy production. Fats - Broken down into fatty acids and glycerol, used for energy (via beta-oxidation). 3. Triacylglycerol (fat) in adipose Glycogen (carbohydrate) mainly in muscle, liver. - - Protein in muscles 4.  main purpose of fuel is to produce ATP by the oxidation of the fuel - ATP needed for: - 5. 1. 2. 3. 4. 5. 6. 1. 2. 3. 4. 5. 6. 7. 1. 2. 3. 4. 5. 6. 8. - - - - - 9. Fat: Higher energy density (9 kcal/g vs 4 kcal/g for carbohydrates). Stored in adipose tissue and can provide long-term energy. Slower to mobilize and utilize. Carbohydrates: Quick source of energy. Stored as glycogen, which is limited in quantity. Easily mobilised, especially during intense exercise. Insulin and glucagon when glucose rises - when amino acid rise - 10. liver glycogen - maintains blood glucose levels during fasting muscle glycogen - provides glucose for muscle contraction - - 11. Total energy used by the body per day, including basal metabolic rate (BMR), physical activity, and the thermic effect of food (TEF). 12. 13. - - - Essential amino acids - - - 14. Nitrogen balance : difference between the daily uptake of nitrogen by body (protein) and the daily loss of nitrogen by body (urea) - - used to make proteins - growing children, pregnant women - 15. A substance that produces an electrically conducting solution when dissolved in water. Examples include sodium (Na+), potassium (K+), calcium (Ca2+), and chloride (Cl-). 16. Vitamins - organic molecules needed in small amounts minerals - non organic compounds that are needed in large amounts - - - - GPT 1. 2. 3. 4. 5. 6. 7. 8. TH lecture 2 and 3 - Glycolysis - aerobic and anaerobic ======================================================= **OVERVIEW - KNOW THIS BY THE END OF UNIT**  1. Catabolic pathways: the breakdown of complex molecules into simpler building blocks needed for the synthesis of other molecules. - Anabolic pathways form complex products from simple precursors, for example the synthesis of glycogen from glucose. - NOTE: In metabolism - multistep pathways - different pathways intersect forming a large network of reactions 2. 3. The [high-energy phosphate bonds] of ATP are a cell's primary source of energy. ATP is generated through - cellular respiration, the oxidation of fuels to CO2 and H20 - How is ATP regenerated -  Energy available from ATP hydrolysis can be used for: - - - Facts of life 1. 2. 3. 4. - Fuel Oxidation has a negative DG0, energy is released. (exergonic reactions) - ATP synthesis has a positive DG0 energy required (endergonic). - NOTE - all reactions have an energy barrier between R and P = activation energy It is also the energy difference between the reactants and a high energy intermediate (transition state) - Exergonic Endergonic  +-----------------------------------+-----------------------------------+ | The product has a lower free | A reaction will proceed | | energy (G) | spontaneously in a forward | | | direction losing energy (-DG) | | than the reactant | until DG is zero and an | | | equilibrium is established. | +-----------------------------------+-----------------------------------+ **[The ATP-ADP cycle]** ATP is hydrolysed to ADP and inorganic phosphate (Pi). - - How is ATP generated and then used? ATP is generated: 1. 2. ATP energy is used: - - - - Energy from food not used for work is converted into heat. **CLINICAL RELEVANCE: ATP and the Heart** Cardiac muscle cells are specialists in converting ATP bond energy (from oxidative phosphorylation) into work energy. BEC - Each single heartbeat uses 2% of the heart's ATP If the heart cannot regenerate its ATP, all its ATP will be hydrolysed in less than a minute. **Cardioplegia during open heart surgery:** Cardioplegia: (combined with hypothermia) is a pharmacological therapy administered during cardiac surgery to intentionally and temporarily arrest the heart - - - **What happens in the heart when ATP is used up, producing ADP?** The myocardium responds to the a drop in ATP/ADP concentration ratio in two ways: 1. 2. **High ADP → elevated phosphate → activates:** 1. 2. - **What are the effects of Adenosine?** 1. Adenosine moves out of cells, causing vasodilation of arterioles, - - 2. Intracellular adenosine opens K+ channels, - - - 3. Adenosine opens K+ channels at the sinoatrial node, - 4. In the glycolytic pathway - glucose is broken down to provide: 1. 2. - - - → oxidised to glucose - **Glucose and Glycolysis** Glucose fact list - - - - Glycolysis facts list - - - - - **How does glucose enter the cell?** Glucose enters the cell by: +-----------------------------------+-----------------------------------+ | **Na+ independent facilitated | **Na+ Monosaccharide | | diffusion** | cotransporter** | +===================================+===================================+ | Glucose Transporters (GLUT 1-14) | Carrier-mediated (SGLT) | | | | | With concentration gradient | Against concentration gradient | | | | | Energy Independent | Energy dependent | | | | | | Coupled to Na+ transport | | | | | | Small intestine, renal tubules & | | | choroid plexus | +-----------------------------------+-----------------------------------+ NOTE : The **Km** of an enzyme is the substrate concentration at which the enzyme operates at half of its maximum velocity (Vmax), reflecting the enzyme\'s affinity for the substrate;  - - **GLUT transporters** Found on the plasma membranes of different tissues: - - **GLUT2** - When does the liver want to take up glucose? - What makes the GLUT2 adaptive to this function? - Liver and pancreatic beta cells have glucose transporters with very high Km, - The glucose transporters in the beta pancreatic cells - **GLUT4** - They have glucose transporters with a Km of about 5mM which - allows these cells to store glucose when it is in excess. **Liver glucose transporters** The liver expresses high levels of GLUT2 and low levels of GLUT 1 transporters. GLUT1 - ensures a constant supply of glucose, especially when glucose levels are low or when energy demand is high. - - 5. 6.  +-----------------------------------+-----------------------------------+ | Oxygen is needed | Oxygen is not needed | +===================================+===================================+ | End product = pyruvate converted | pyruvate is converted into | | to acetyl CoA which enters the | lactate | | TCA cycle | | | | Can occur without Mit (RBC) | +-----------------------------------+-----------------------------------+ | | IMP for cells with limited supply | | | of Oxygen | | | | | | - | | | | | | IMP for no or few mitochondria | | | | | | - | +-----------------------------------+-----------------------------------+ | More energy produced | Less energy produced | +-----------------------------------+-----------------------------------+ | | Tissue under pathological hypoxia | | | can produce a large amount of | | | lactic acid causing - lactic | | | acidaemia | +-----------------------------------+-----------------------------------+ 7. Glycolysis consists of 10 reactions divided into 2 phases: 1\] Preparative phase (5 reactions). - - 2\] ATP-generating phase. (5 reactions). - - - -  8. Phase 1 - ATP is used 1. Glucose is phosphorylated by ATP to form Glucose-6-phosphate. Phosphorylation catalysed by *hexokinase* - - Glucose-6-phosphate metabolism - regulated step - determined by energy requirements Glucose-6-phosphate can be produced from: Gluconeogenesis and Glycogenolysis It is an important BRANCH POINT 1. 2. 3. NOTE: Branching is important as it acts as a safety valve - as when glucose increases Glucose-6-phosphate is converted to several products so that it does not slow down glycolysis while also producing several side products. **Hexokinase and Glucokinase (Compare and contrast kinetic prop wrt physiology)** Hexokinase and glucokinase are isozymes. **Hexokinase** **Glucokinase** ----------------------------------------------------------------------- ------------------------------------------------------------------------------- **Location**: Most tissues (muscle, brain, RBC). **Location**: Liver and pancreatic beta cells. **Km**: Low (high affinity) for glucose; active at low blood glucose. **Km**: High; requires high glucose concentration for activity. **Saturation**: Fully saturated at low substrate levels. **Activation**: Most active after carbohydrate-rich meals. **Inhibition**: Inhibited by glucose-6-phosphate (G6P). **Inhibition**: Not inhibited by G6P. **Vmax**: Low **Vmax**: High; effectively removes excess glucose, minimising hyperglycemia. Even at very low glucose levels (below fasting, 5mM), RBC hexokinase can still phosphorylate glucose at rates near Vmax. Liver glucokinase will phosphorylate glucose when levels are high, after a high carbohydrate meal. Consequently the high Km of glucokinase promotes the synthesis of glycogen and fat when glucose is present in excess. NOTE RBC uses hexokinase - even at low conc but only slow Liver uses glucokinase - only at higher conc but converts more Vmax: Maximum rate of an enzyme-catalysed reaction at full substrate saturation. Km: Substrate concentration at which the reaction rate is half of Vmax; indicates enzyme affinity for substrate (low Km = high affinity). 2. This reaction is reversible and is not a regulated step. 3. - - - - - **Regulation of phosphofructokinase 1 (PFK-1)** PFK-1 is inhibited by: 1. PFK-1 is allosterically inhibited by ATP. Glycolysis slows down when cellular ATP concentrations are high. ATP binds to a site on PFK-1 distinct from its active site, causing a conformational change. 2. Citrate (a TCA cycle intermediate) inhibits PFK, indicating abundant biosynthetic precursors. - citrate is one of the only intermediates that leave the mitochondria, so it can regulate glycolysis in the cytoplasm. Regulation by pH: - - - Biosynthetic Role: - Fructose-2,6-bisphosphate: - - - - NOTE - Glyceraldehyde-3 phosphate is the favoured product - Dihydroxyacetone phosphate is used for a side reaction to make glycerol NOTE: citrate usually increases during the well fed state but it may also increase during a fast due to the breakdown of fat → Acetyl CoA 4. Fructose 1,6-phosphate is cleaved into 2 molecules: - - This reaction is reversible and is not regulated. 5. 2 mol of glyceraldehyde 3-phosphate are formed from fructose 1,6-bisphosphate. - The glyceraldehyde 3-phosphate continues the glycolytic pathway - PHASE 2 - Energy is produced 6. - - In this reaction - - NOTE: The NADH is used to make ATP by the electron transport chain and oxidative phosphorylation. NOTE: Oxidation is the loss of electrons. - - Reduction is the gain of electrons. - - 7. - The phosphate bond synthesised in the previous reaction (step 6) is hydrolysed - - The reaction is catalysed by *phosphoglycerate kinase.* **2,3-Bisphosphoglycerate (2,3-BPG)**: - - - **Oxygen Transport**: - - The concentration of 2.3BPG is raised in smokers, due to CO - Commonly increased in patients with: - - - - 8. - - - 9. 10. - - - - Glucose + 2NAD+ +2Pi + 2ADP 2 pyruvate + 2NADH+ 4 H+ +2ATP + 2 H2O **RBC Energy Supply**: - - **Impact on RBC Integrity**: - - **Hemolytic Anemia Development**: - - 9. 10. 11. **The Glycolytic pathway is regulated in several ways:** 1. **Example**: High glucose concentration increases glycolysis rate. 2. **Example**: Phosphofructokinase-1 (PFK-1) levels are regulated based on metabolic needs. 3. **Example**: PFK-1 is activated by AMP and fructose-2,6-bisphosphate; inhibited by ATP and citrate. 4. **Example**: Pyruvate kinase can be inactivated by phosphorylation in response to glucagon. **Hormonal regulation of rate-limiting reactions:** **Insulin** Insulin increases the activity of key glycolytic enzymes to enhance glucose breakdown. - - **Glucagon** Glucagon decreases the activity of glycolytic enzymes to conserve glucose. - - - - - NOTE: Insulin - Glucagon - NOTE - some enzymes are activated by phosphorylation others by dephosphorylation Ex - PFK2 - Insulin - dephosphorylation - activated - 12. **Anaerobic glycolysis** the lactate dehydrogenase reaction - - - Note- thus only 2 ATP produced from anaerobic glycolysis. **Glycogen Degradation in Muscle Cells** Exercising skeletal muscle cells and hypoxic cells rapidly degrade glycogen to produce glucose-1-phosphate substrate for glycolysis. **ATP Production** -Aerobic respiration produces at least 15 times more ATP than anaerobic glycolysis. \- For RBCs to produce this amount of energy, anaerobic glycolysis must be 15 times faster than aerobic respiration. \- Certain cells achieve this by expressing high levels of glycolytic enzymes. **Hemolytic Anaemia** \- Deficiency in glyceraldehyde 3-phosphate dehydrogenase and pyruvate kinase leads to hemolytic anaemia. As RBCs depend on glycolysis for ATP. **Fate of NADH** NADH produced needs to be reoxidised to NAD+ - NADH may be reoxidised by one of two methods: 1. 2. NOTE: The conversion of pyruvate to lactate is mainly to regenerate NAD+ Some Lactate is found in the body for 2 reasons - - 13.  In lactic acidosis, the levels of lactic acid may increase Thus → drop in blood pH. - Lactic acidosis results from an increase in NADH/NAD+ ratio in tissues. - **Blood Lactate Concentration:** - - - Pyruvate enters the mitochondria via pyruvate transporters. In the mitochondria pyruvate is converted to acetyl CoA which enters the TCA cycle. NOTE- that a halt in the TCA cycle will cause Acetyl coA to accumulate → causing pyruvate to accumulate → causing lactate to accumulate. 14. 15. Red blood cell enzymopathies of glycolytic pathway Inherited diseases due to deficiency of RBC glycolytic enzymes are rare causes of hereditary non spherocytic haemolytic anaemia. This is serious as the RBC is entirely dependent on glycolysis, for: 1\] the production of ATP 2\] the production of 2,3-BPG. (increase O2 release to tissues by stabilising deoxyHb.) 1 What will happen to the cellular level of 2,3-BPG if there is a deficiency in hexokinase or pyruvate kinase? If there is a deficiency in hexokinase or pyruvate kinase, the cellular level of 2,3-BPG will likely increase due to a reduced flux through glycolysis, leading to a buildup of glycolytic intermediates. 2 Why does a high NADH/NAD+ ratio result in lactic acidemia? A high NADH/NAD+ ratio inhibits the conversion of pyruvate to acetyl CoA, causing pyruvate to be redirected toward lactate production, which results in lactic acidemia. 3 What is the role of heart phosphofructokinase-2 during ischaemia? During ischemia, heart phosphofructokinase-2 activates glycolysis by increasing fructose-2,6-bisphosphate levels, enhancing ATP production in the absence of sufficient oxygen. 4 Explain the function of 2,3-bisphosphglycerate in RBCs during adaption to high altitude and anaemia In red blood cells, 2,3-bisphosphoglycerate (2,3-BPG) decreases hemoglobin\'s affinity for oxygen, facilitating oxygen release to tissues during adaptation to high altitude and anemia. 5 A 64 year old woman suffers from myocardial infarction. The ischaemic area in her heart had a limited supply of oxygen and blood-borne fuels. Explain the effect this has on intracellular pH. The limited supply of oxygen and blood-borne fuels during myocardial infarction leads to increased anaerobic metabolism, resulting in lactate accumulation and a decrease in intracellular pH. Explain the effect this change in pH has on glycolysis.This lower pH inhibits glycolytic enzymes, slowing down glycolysis and further exacerbating energy deficits. **GPT Glycolysis** \#\#\# Step 1: Phosphorylation of Glucose 1\. \*\*Hexokinase or Glucokinase\*\*: \- Glucose is phosphorylated to form \*\*glucose 6-phosphate (G6P)\*\*. \- \*\*Hexokinase\*\* is the main enzyme in most tissues, while \*\*glucokinase\*\* is predominant in liver and pancreatic β-cells. \- Hexokinase has a low Km (high affinity) for glucose and is inhibited by its product, G6P. Glucokinase has a higher Km (requires more glucose for activation) and a high Vmax, allowing it to efficiently phosphorylate glucose during high glucose levels (e.g., after a carbohydrate-rich meal). \#\#\# Step 2: Isomerization of Glucose 6-Phosphate 2\. \*\*Phosphoglucose Isomerase\*\*: \- G6P is isomerized to \*\*fructose 6-phosphate (F6P)\*\*. \- This reaction is reversible and not a rate-limiting step. \#\#\# Step 3: Phosphorylation of Fructose 6-Phosphate 3\. \*\*Phosphofructokinase-1 (PFK-1)\*\*: \- F6P is phosphorylated to \*\*fructose 1,6-bisphosphate (F1,6BP)\*\*. \- This is the rate-limiting step of glycolysis and is regulated by ATP (inhibitor) and AMP (activator). Fructose 2,6-bisphosphate is a potent activator of PFK-1. \#\#\# Step 4: Cleavage of Fructose 1,6-Bisphosphate 4\. \*\*Aldolase\*\*: \- F1,6BP is cleaved into two triose sugars: \*\*dihydroxyacetone phosphate (DHAP)\*\* and \*\*glyceraldehyde 3-phosphate (G3P)\*\*. \- This reaction is reversible and not regulated. \#\#\# Step 5: Isomerization of Dihydroxyacetone Phosphate 5\. \*\*Triose Phosphate Isomerase\*\*: \- DHAP is converted into \*\*glyceraldehyde 3-phosphate (G3P)\*\*. \- The isomerization results in a net production of two G3P molecules from one F1,6BP. \#\#\# Step 6: Oxidation of Glyceraldehyde 3-Phosphate 6\. \*\*Glyceraldehyde 3-Phosphate Dehydrogenase\*\*: \- G3P is oxidized to \*\*1,3-bisphosphoglycerate (1,3-BPG)\*\*, and NAD+ is reduced to NADH. \- This is the first oxidation-reduction reaction in glycolysis. The oxidation of G3P generates a high-energy phosphate in 1,3-BPG. \#\#\# Step 7: Synthesis of 3-Phosphoglycerate 7\. \*\*Phosphoglycerate Kinase\*\*: \- 1,3-BPG donates a phosphate group to ADP, forming \*\*3-phosphoglycerate (3-PG)\*\* and producing ATP via substrate-level phosphorylation. \- This step replaces the ATP consumed in earlier steps. \#\#\# Step 8: Shift of the Phosphate Group 8\. \*\*Phosphoglycerate Mutase\*\*: \- The phosphate group in 3-PG is shifted from carbon 3 to carbon 2, forming \*\*2-phosphoglycerate (2-PG)\*\*. \- This reaction is reversible. \#\#\# Step 9: Dehydration of 2-Phosphoglycerate 9\. \*\*Enolase\*\*: \- 2-PG is dehydrated to form \*\*phosphoenolpyruvate (PEP)\*\*, which contains a high-energy enol phosphate. \- This reaction is reversible despite the high-energy nature of the product. \#\#\# Step 10: Formation of Pyruvate 10\. \*\*Pyruvate Kinase\*\*: \- PEP is converted to \*\*pyruvate\*\*, producing ATP through substrate-level phosphorylation. \- This is the second irreversible step in glycolysis. Pyruvate kinase is activated by fructose 1,6-bisphosphate and inhibited by ATP and glucagon. \#\#\# Final Steps: Fate of Pyruvate \- \*\*Aerobic Conditions\*\*: Pyruvate is further oxidized in the TCA cycle after conversion to acetyl-CoA. \- \*\*Anaerobic Conditions\*\*: Pyruvate is reduced to \*\*lactate\*\* via \*\*lactate dehydrogenase\*\*, regenerating NAD+ for glycolysis to continue. \#\#\# Energy Yield from Glycolysis \- \*\*Anaerobic Glycolysis\*\*: Produces 2 ATP and 2 lactate per glucose molecule. \- \*\*Aerobic Glycolysis\*\*: Also produces 2 ATP, but generates 2 NADH, which can yield an additional 5 ATP via oxidative phosphorylation, resulting in a total of approximately 7 ATP per glucose molecule.  1. Pyruvate Dehydrogenase Reaction Pyruvate - end product of glycolysis - - NOTE → The pyruvate dehydrogenase reaction joins the TCA cycle to the glycolytic pathway. 2. 3. **Glycolysis:** Takes place in the cytosol. **TCA Cycle:** Takes place in the mitochondrial matrix. 4. Acetyl CoA - Generated - - Pyruvate is oxidised to acetyl CoA by the pyruvate dehydrogenase complex. **Explain how pyruvate dehydrogenase is regulated** Pyruvate Dehydrogenase Complex. The pyruvate dehydrogenase complex is composed of three enzymes: - - - Moreover, it is associated with 2 regulatory enzymes: - - And a number of coenzymes: Thiamine pyrophosphate, lipoic acid and CoA, FAD and NAD+. Regulation of the reaction - oxidative decarboxylation reaction 1. - Both inhibit the dephosphorylated form of pyruvate dehydrogenase allosterically. 2. (phosphorylation → inhibition) Pyruvate dehydrogenase kinase (PDH kinase) phosphorylates pyruvate dehydrogenase - PDH is inhibited. Increase in Acetyl CoA, ATP and NADH activate pyruvate dehydrogenase kinase. - 3. (dephosphorylation → activation) Ca2+ ions are released from muscle cells during contraction. - PDH phosphatase - dephosphorylates and thus activates PDH - 5.  6. 1. Acetyl CoA and oxaloacetate -- condensation → citrate. - NOTE - When oxaloacetate binds to citrate synthase, a conformational change occurs in the enzyme forming → a binding site for acetyl CoA. Regulation - Citrate synthase is inhibited by: 1. 2. 3. 4. Citrate is an important intermediate of metabolism. 2. 3. 4. 2. 3. Citrate is isomerised to isocitrate - Isocitrate is converted to a-ketoglutarate. This is an irreversible oxidative decarboxylation of isocitrate is - NOTE - **Fluoroacetate**, - -  Oxidative reaction as the H of isocitrate is used to reduce NAD+ Imp Bec 1. 2. 3. - - Inc Ca2+ - muscle contraction - thus indicates that ATP is needed Inc ATP and NADH - energy surplus - thus ATP is not needed. 4. The conversion of a-ketoglutarate to succinyl CoA - This reaction: 1\] releases the second CO2 of the TCA cycle. 2\] produces the second NADH of the TCA cycle. 3\] is inhibited by ATP, NADH, GTP, succinyl CoA, 4\] is activated by Ca2+ NOTE: Needs coenzymes → thiamine pyrophosphate, CoA, lipoic acid ,NAD+ and FAD. NOTE: Alpha-ketoglutarate is also produced in a different reaction from the amino acid glutamate. 5. The conversion of succinyl CoA to succinate - 1\] This cleaves the high energy thioester bond of succinyl CoA releasing CoA. 2\] This coupled to the substrate level phosphorylation of GDP to form GTP. 6. Succinate is oxidised to fumarate Catalysed by succinate dehydrogenase. - **NOTE:** Succinate dehydrogenase is the only enzyme of the TCA cycle that is embedded in the inner mitochondrial membrane. **NOTE:** Succinate dehydrogenase functions as Complex II on the Electron Transport Chain (ETC). **NOTE:** NADH plays a regulatory role in balancing energy metabolism that FAD(H2) cannot because FAD(H2) remains attached to its enzyme. TCA cycle and other pathways of fuel oxidation are regulated by the NADH/NAD+ ratio - so it controls fuel oxidation and the rate of ATP synthesis. - 7. 8. Fumarate is hydrated to malate in a reversible reaction - Malate is oxidised to oxaloacetate - This reaction produces the third NADH of the TCA cycle. NOTE: Fumarate is produced by other metabolic reactions - - - Oxaloacetate is also produced from the amino acid aspartic acid.  **Energy produced by the TCA cycle** 2 carbon atoms enter as acetyl CoA and leave as 2 CO2 molecules. There is no net consumption of oxaloacetate. 4 pairs of electrons are transferred during one turn of the TCA cycle. - - **Anaplerosis 'fill-in'** - Anaplerotic - Pathways/reactions that replenish the intermediates of TCA cycle Anaplerosis - the process of replenishing TCA cycle intermediates that are depleted during metabolic reactions, - The use of TCA intermediates by other reactions reduces the amount of oxaloacetate produced.  TCA Intermediates as Precursors: Serve as precursors for other metabolic pathways. 1. 2. 3. 4. 5. - - 6. 7. **Pyruvate carboxylase** IMP bec it catalyses the addition of carbon dioxide to pyruvate to make oxaloacetate. - NOTE: a carboxylase always uses Biotin as a coenzyme If Oxaloacetate levels decrease due to efflux if TCA intermediates - - - -  The rate of ATP hydrolysis by the cell controls the rate of ATP synthesis The rate at which e form NADH enters the ETC - - - Inc in NADH concentrations inhibit Isocitrate dehydrogenase, alpha-ketoglutarate dehydrogenase and malate dehydrogenase The NADH/NAD+ ratio changes the concentration of oxaloacetate. Citrate is the a product inhibitor of citrate synthase. ADP is an allosteric activator of isocitrate dehydrogenase. During muscular contraction, increased Ca++ concentration activates citrate dehydrogenase and alpha-ketoglutarate dehydrogenase and pyruvate dehydrogenase. Alcohol and heart failure An alcoholic is hospitalised after suffering heart failure. Explain the link between the man's excessive alcohol intake and his condition. **Increased NADH Levels:** Alcohol metabolism generates high levels of NADH, disrupting the NADH/NAD+ ratio and inhibiting TCA cycle enzymes. **Oxaloacetate Depletion:** Elevated NADH can lead to reduced levels of oxaloacetate, slowing down the TCA cycle and energy production. **Fatty Acid Accumulation:** Impaired TCA cycle function can result in the accumulation of fatty acids, contributing to alcoholic cardiomyopathy. **Metabolic Acidosis:** Increased lactate production due to disrupted energy metabolism can lead to metabolic acidosis, further stressing the heart. **Nutritional Deficiencies:** Alcoholism may lead to deficiencies in key nutrients (e.g., thiamine) that are critical for TCA cycle function and overall heart health. Leigh's disease 1\) Name the enzymes whose deficiency causes Leigh's disease. Pyruvate dehydrogenase (PDH), Alpha-ketoglutarate dehydrogenase (α-KGDH), Succinate dehydrogenase (SDH), Fumarase (Fumarate hydratase), Complex I of the electron transport chain 2\) Make a connection between the metabolic role of these TCA enzymes and the types of symptoms associated with this condition. **Energy Production:** Impaired ATP production affects high-energy tissues (e.g., brain, muscles). **Lactic Acidosis:** Reduced conversion of pyruvate leads to increased lactate and metabolic acidosis, causing muscle weakness. **Neurological Symptoms:** Energy deficits in the brain result in developmental delays, seizures, and ataxia. **Failure of Metabolic Pathways:** Disruption causes toxic metabolite accumulation, worsening neurological and muscular symptoms. **Multi-Organ Impact:** Affects various organs, leading to cardiac issues, liver dysfunction, and respiratory problems. 7. A rate-limiting reaction is the slowest step in a metabolic pathway that determines the overall rate of that pathway. 8. - - - 9. NADH: Vitamin B3 (niacin) is needed for NADH production. FADH2: Vitamin B2 (riboflavin) is required for FADH2 production. 10. Citrate synthase is regulated allosterically by acetyl-CoA and oxaloacetate, and is inhibited by citrate and ATP. 11. Isocitrate dehydrogenase is activated by ADP and NAD+, and inhibited by ATP and NADH. 12. Alpha-ketoglutarate dehydrogenase is activated by AMP and NAD+, and inhibited by succinyl-CoA and NADH. 13. Succinate is the TCA intermediate that also serves as a component of the electron transport chain (via succinate dehydrogenase). 14. The TCA cycle generates NADH and FADH2, which donate electrons to the electron transport chain, ultimately driving ATP production through oxidative phosphorylation. 15. NADH: Approximately 2.5 ATP per molecule. FADH2: Approximately 1.5 ATP per molecule Electron Transport Chain and Oxidative phosphorylation ====================================================== **Overview** **Formation of NADH** - **ETC & Oxidative Phosphorylation** - - - - - **Oxidative phosphorylation** is a metabolic pathway that utilises energy released by redox reactions of the ETC to synthesise ATP 1. **The Mitochondria** The ETC is in the - inner mitochondrial membrane. The inner mitochondrial membrane - - - The Mit matrix contains DNA, RNA ,many enzymes, ADP, Pi and NAD+ and FAD. Generation of ATP from oxidative phosphorylation requires: - - - - - - **The outer mitochondrial membrane** has pores that allow the movement of small molecules and most ions into and out of the mitochondria. **The inner mitochondrial membrane** is rich in proteins involved in the electron transport chain and oxidative phosphorylation - folds (cristae) inc SA **The mitochondrial matrix** is a gel-like solution also rich in protein. Many are enzymes needed for the metabolism of pyruvate, amino acids and fatty acids, for the TCA cycle for example. 2. Complex I: NADH is oxidized, reducing ubiquinone. Complex II: FADH₂ is oxidized, reducing ubiquinone. Complex III: Ubiquinol (reduced ubiquinone) is oxidized, and cytochrome c is reduced. Complex IV: Cytochrome c is oxidized, reducing oxygen to water.\ **Overview of the Electron Transport Chain (ETC):** - - - - - - - - **Key Components of the ETC:** - - - - **Electron Transport and Proton Pumping:** - - - - **Free Energy and Electron Transfer:** - - - - - 3. **Proton Gradient and Energy Use:** **Proton gradient:** The energy released from electron transfer is used to pump protons against their concentration gradient, leading to their accumulation in the intermembrane space. - - **Proton impermeability:** The inner mitochondrial membrane is impermeable to protons, preventing them from diffusing back into the matrix. **Energy release:** - - **Role of ATP Synthase:** ATP synthesis: Protons flow back into the mitochondrial matrix through ATP synthase (Complex V), using the energy of the proton gradient to convert ADP and Pi into ATP. **Complexes**  1. Has binding sites for - NADH, FMN, Fe-S and CoQ Electron route: NADH → FeS → CoQ Drop in energy bet FeS and CoQ - results in movement of 4H+ 2. NOTE: also enz in TCA cycle There is no significant drop in energy → thus no energy is released The complex does not span the membrane -- thus no H+ are transferred 3. When oxidised it forms - semiquinone -- free radical -- highly reactive. NOTE: major site for toxic oxygen free radical formation. Semiquinone can accept 2 electrons and 2 protons. Hydrophobic - can diffuse through the membrane - accepts electrons - shuttles electrons between complex 1 and 3 as it donates protons to them. 4. NOTE: Cytochrome contains a Haem prosthetic group, each with a different reduction potential. Comp III has a higher energy level than comp IV thus energy is lost as electrons are transferred. 5. Passes electrons to oxygen - as O2 binds to it and accepts 4e- Cytochrome oxidase has a much lower Km for oxygen than myoglobin or haemoglobin. Therefore oxygen is pulled from the erythrocyte to myoglobin and from myoglobin to cytochrome oxidase, where it is reduced to water and generates ATP 4. **The Electrochemical potential gradient: Proton motive force** This generates an electrochemical gradient- made up of two components: 1. The cytosolic side of the inner membrane is charged positive relative to the matrix side. 2. The intermembrane space has a higher concentration of protons and is therefore more acidic than the matrix. The electrochemical potential gradient is also called the proton motive force because is provides the potential energy to drive the protons back into the negatively charged alkaline matrix. **Chemiosmotic Hypothesis -** explains how the free energy generated by the transport of electrons by the (ETC) is used to produce ATP from ADP + Pi. This requires 2 components: - - The 3 ETC complexes - complex I, III and IV , there is electron transfer and proton pumping - membrane is impermeable to protons thus they cannot diffuse back into the matrix. As a result a membrane potential - Thus a proton gradient forms - Each complex of the chain is at a lower energy level than the previous complex , so energy is released as electrons pass through each complex. 5. **The proton motive force (PMF)** is the electrochemical gradient generated by the movement of protons across the inner mitochondrial membrane during the electron transport chain, - - - - - - 6.  7. **Complex V** is ATP Synthase aka F1/Fo ATPase. **The chemiosmotic hypothesis** proposed that the protons that have been pumped out of the matrix return to the mitochondrial matrix by passing through the membrane spanning channel component Fo of ATP Synthase. - - NOTE: Since protons have been moved back into the mitochondrial matrix the electrical gradient and the pH gradient dissipate. NOTE: It takes 12 protons to synthesise 3 ATP ATP synthase has 12 subunits - - - - 8. 9. +-----------------------+-----------------------+-----------------------+ | | **Mode of action** | **Site of | | | | inhibition** | +=======================+=======================+=======================+ | **Rotenone (an | Blocks electron | NADH dehydrogenase | | insecticide)** | transport | (Complex I) | | | | | | **Amobarbitol | | | | (barbiturate)** | | | +-----------------------+-----------------------+-----------------------+ | **Antimycin A | Blocks electron | Complex III | | (antibiotic)** | transport | | +-----------------------+-----------------------+-----------------------+ | **Cyanide, azide, | Blocks electron | Complex IV | | CO** | transport | | +-----------------------+-----------------------+-----------------------+ | **Oligomycin | Blocks proton flow | ATP synthase | | (antibiotic)** | through ATP synthase | | +-----------------------+-----------------------+-----------------------+ | **Dinitrophenol** | Uncouples ATP | Nonspecific site | | | synthesis from ETC | | +-----------------------+-----------------------+-----------------------+ | **Atractyloside** | Inhibits ATP-ADP | ATP-ADP translocase | | | exchange | | +-----------------------+-----------------------+-----------------------+ **-Uncouplers (e.g., dinitrophenol)**: Carry protons back to the matrix, bypassing ATP synthase. **-Effect**: Reduces ATP production per mole of oxygen consumed. **-Energy loss:** Heat is produced instead of ATP, leading to hyperthermia. **-Thermogenin:** An uncoupling protein in brown adipose tissue that generates heat. **- Atractyloside:** A poison found in \'glue thistle.\' 10. 11. The TCA cycle occurs in the mitochondria - thus NADH produced from TCA already in the mitochondria matrix for ETC - no need of shuttle Glycolysis occurs in the cytoplasm, generating NADH - these NADHs need shuttle to enter Mitochondrial matrix for ETC NADH cannot pass through the mitochondrial membrane to enter the mitochondria. This is bec - The inner mit membrane does not have an NADH transport protein. - **2 shuttle mechanisms** - - - - NOTE:Heart and liver tissue rely on the malate-aspartate shuttle. NOTE:Many cells including skeletal muscle cells use the glycerol P shuttle.\  **The malate-aspartate shuttle** 1. 2. 3. 4. 5. a. 6. 12. 1. 2. 3. 4. There is a suggestion that the glycerol phosphate shuttle is of particular importance in nervous tissue, where aspartate has a role as an excitatory neurotransmitter in the ventral spinal cord. **3 sites where protons are pumped out of the matrix**: this is at **Comp I, III and IV.** - - - - 13. OXPHOS diseases are disorders that involve components of oxidative phosphorylation. These degenerative diseases may be caused by gene mutations in either mitochondrial DNA or nuclear DNA that encode proteins required by oxidative phosphorylation. One example is Leber's hereditary Optic Neuropathy. Cause: mitochondrial DNA mutation in NADH dehydrogenase subunit. Loss of central vision and eventual blindness due to degeneration of optic nerve. 14. 15. Lactic acidosis Decreased activity of the electron transport chain can result from inhibitors as well as from mutations in mt DNA and nuclear DNA. Why does this impairment of the electron transport chain result in lactic acidosis? \- \*\*ETC impairment\*\*: Caused by inhibitors or mutations in mitochondrial/nuclear DNA. \- \*\*Reduced oxidative phosphorylation\*\*: Limits ATP production from the ETC. \- \*\*Increased glycolysis\*\*: Cells rely on anaerobic glycolysis for ATP. \- \*\*Lactate buildup\*\*: Pyruvate is converted to lactate due to lack of oxygen utilization. \- \*\*Result\*\*: Accumulation of lactate leads to \*\*lactic acidosis\*\*. TH - Glycogen metabolism ======================== 1. Glycogen is a branched-chain homopolysaccharide made only from a-D-glucose. - - Main stores- skeletal muscle and liver. Muscle - reservoir of fuel for ATP synthesis Liver - to maintain blood glucose at early stage of fast NOTE: Glycogen stores increase during the well-fed state and decrease during a fast. NOTE: The branched structure of glycogen allows rapid degradation and rapid syn of glycogen because enzymes can act on several nonreducing ends simultaneously. 2. Functions of muscle and liver glycogen **Liver glycogen** stores increase in the well-fed state and decrease during a fast. **Muscle glycogen** is synthesised to replace stores used during exercise. NOTE: Muscle glycogen is not affected by short periods of fasting and is only moderately decreased during long periods of fasting. NOTE: Glycogen synthesis and degradation occur continuously in the cytoplasm - the difference in rate of synthesis and degradation effect how much is stored 3. Muscle - reservoir of fuel for ATP synthesis Liver - to maintain blood glucose at early stage of fast 4. General characteristics - - - - - - - Note: Glycogen synthase cannot join 2 free glucose molecules together so it adds glucose-UDP to an existing branch. NOTE: Synthesis and breakdown of glycogen are controlled in an integrated fashion via allosteric and covalent mechanisms. Hormonal control is critical. **ENZYMES of Glycogen overview** **1. Phosphoglucomutase**: Converts glucose-1-phosphate to glucose-6-phosphate, facilitating entry into glycolysis or gluconeogenesis. **2. Glycogen Synthase**: Catalyzes the addition of glucose units to the growing glycogen chain, extending it during glycogen synthesis. **3. Glycogen Phosphorylase**: Breaks down glycogen by cleaving glucose units as glucose-1-phosphate, enabling glycogenolysis. **4. Glycogenin:** Acts as a primer by attaching the first glucose molecules to itself, initiating glycogen synthesis. **5. Branching Enzyme**: Creates branches in the glycogen molecule by transferring segments of glucose chains, enhancing glycogen solubility and energy availability. **Enzymes required:** - - - - **Role of glycogenin:** - - - - - **Branching enzyme:** A different enzyme adds glucose with α(1→6) glycosidic bonds, forming branches; these occur after every 8 glucose residues. **Glycogen synthesis process:** Most glycogen synthesis involves lengthening existing polysaccharide chains of a pre-existing glycogen molecule (glycogen primer). - **Key Points about glycogen synthesis** - - - - - - 5. **Glycogen synthase** is the key enzyme responsible for catalysing the formation of α(1→4) glycosidic bonds, adding glucose molecules to the growing glycogen chain using UDP-glucose as a donor. 6. **Role of glycogenin:** - - - - - 7. **Advantages of branches in glycogen:** Branches provide multiple points for synthesis and degradation processes while enhancing the solubility of the glycogen polymer. 8. Glycogenolysis occurs in the **cytosol** of cells. 9. **Glycogen Degradation: Glycogenolysis** - - - - Key points on **Glycogenolysis** - - - 10. **Conversion of glucose-1-P to glucose-6-P to glucose** - **Muscle** **Liver** ----------------------------------------------------------------------------------------------------------------------------------------------------- -------------------------------------------------------------------------------------------------------------------------------------------- **Muscle** lacks glucose 6 phosphatase so the products of glycogenolysis in muscle will be pyruvate or lactate. **Liver** has glucose 6 phosphatase, The product is therefore glucose. The role of glycogen in **muscle** is to supply ATP The role of glycogen in the **liver** is to maintain blood glucose levels. **Muscle glucose-6-P** cannot be dephosphorylated to glucose. This muscle glucose-6-P enters glycolysis and provides energy for muscle contraction. **Liver glucose-6-P** is converted into glucose by glucose 6-phosphatase. The glucose is released into the blood to maintain blood glucose NOTE: Pyridoxal phosphate is the active form of vitamin B6. NOTE: In glycogenolysis, glucose-6-phosphatase releases free glucose from glucose-6-phosphate. **Glycogen storage disorders** result from deficiencies in enzymes involved in glycogenolysis - Most result in Hypoglycemia Some glycogen is degraded in the cell\'s lysosomes. - - - 11. 12. **Regulation of glycogen metabolism** +-----------------------------------+-----------------------------------+ | **Muscle** | **Liver** | +===================================+===================================+ | **During rest:** Glycogen | **Well-fed state:** Glycogen | | synthesis starts again. | synthesis increases. | | | | | **During exercise:** Glycogen | **Fasting state:** Glycogen | | degradation increases. | degradation increases. | +-----------------------------------+-----------------------------------+  [Regulation is done by three ways:] 1\] Allosteric control of glycogen phosphorylase and glycogen synthase. 2\] Covalent modification 3\] Hormone regulation of glycogen synthesis and glycogen degradation **Allosteric regulation liver** [Glycogen synthesis is stimulated in response to:] A\] Substrate availability ie. high glucose levels and B\] High energy levels (high ATP). [Glycogen degradation is stimulated in response to:] A\] low glucose levels B\] Low energy levels (low ATP levels). [In the well-fed state in the **liver**:] 1\] Glycogen synthase is activated by glucose 6-phosphate while 2\] Glycogen phosphorylase is inhibited by glucose 6-phosphate and ATP. **In the liver**, glucose is an allosteric inhibitor glycogen phosphorylase. Glycogen degradation and synthesis are regulated by the ratio of **insulin to glucagon** and also blood glucose. Glycogen degradation is also regulated by **epinephrine** in response to exercise, hypoglycemia and stress conditions. **Allosteric regulation in muscle cells** During muscle contraction there is an immediate and urgent need for ATP. ATP is made available by the degradation of glycogen to glucose 6-phosphate. 1\] Neural stimulation of muscle cells results in an increase of Ca2+ → activates glycogen phosphorylase and stimulates the release of glucose 1-P from glycogen. 2\] High levels of AMP activate glycogen phosphorylase and stimulate the degradation Muscle glycogenolysis is also regulated by epinephrine. Epinephrine is released in response to exercise, stress. Glycogen phosphorylase - active when phosphorylated Glycogen synthase - active when unphosphorylated  13. Insulin and glucagon regulate liver glycogen metabolism by altering the phosphorylation of glycogen phosphorylase and glycogen synthase. During fasting, 1. 2. 3. 4. 5. 6. 7. Effect of glucose levels on glycogen degradation The cellular response to glucose is rapid. It is an allosteric effector that stimulates dephosphorylation and inactivation of glycogen phosphorylase a. 14. **Epinephrine** and **glucagon** bind to protein receptors (muscle and liver cells) on membrane - this signal triggers glycogen degradation for energy production by inhibiting glycogen synthase. The sequential reactions form a cascade that amplifies the hormonal signal. +-----------------------------------+-----------------------------------+ | **REGULATION OF GLYCOGEN | **REGULATION OF GLYCOGEN | | METABOLISM: KEY POINTS** | METABOLISM: KEY POINTS** | +===================================+===================================+ | - | - | | | | | An increase in glucagon will | Insulin is capable of reversing | | result in the phosphorylation and | all the effects of glucagon. | | activation of glycogen | | | phosphorylase and the | - | | phosphorylation and inactivation | | | of glycogen synthase. | Glucose serves as an allosteric | | | inhibitor of liver phosphorylase | | - | a by stimulating the | | | dephosphorylation of the enzyme. | | By binding to its membrane | As insulin levels rise, protein | | receptor, glucagon transmits a | phosphatases dephosphorylate | | signal via G-proteins and cAMP to | phosphorylase a and glycogen | | protein kinase. The latter will | synthase b. As a result glycogen | | phosphorylate and activate | degradation is inhibited and | | phosphorylase kinase which will | glycogen synthesis is activated. | | in turn phosphorylate and | | | activate glycogen phosphorylase | - | | a. | | | | Epinephrine is released in | | - | response to a demand in glucose. | | | Regulation is similar to that of | | Protein kinase activated by | glucagon where | | glucagon signalling, will | | | | glycogen phosphorylase is | | phosphorylate and deactivate | activated by phosphorylation | | glycogen synthase. | | | | and glycogen synthase is | | - | inactivated by phosphorylation. | | | | | Glucagon inhibits protein | | | phosphatases that dephosphorylate | | | phosphorylase a and glycogen | | | synthase. | | +-----------------------------------+-----------------------------------+ **REGULATION OF GLYCOGEN METABOLISM IN MUSCLE CELLS : KEY POINTS** Need of ATP for contraction regulates glycogen metabolism - - - **Differences between liver and muscle glycogen metabolism:** Glucagon has no effect on muscle - AMP is an allosteric effector of muscle glycogen phosphorylase but not of liver glycogen phosphorylase. Glucose is not a physiological inhibitor of glycogen phosphorylase in muscle. Glycogen is a stronger feedback inhibitor of muscle glycogen synthase than of liver glycogen synthase thus→ smaller amount of glycogen stored per gram weight of muscle tissue. \*\*Foetal Glucose Levels:\*\* 1\. \*\*Glucose regulation in newborns:\*\* \- Newborn's glucose drops after birth due to loss of placental supply. \- Liver breaks down glycogen (glycogenolysis) to produce glucose. \- Gluconeogenesis is activated. \- Feeding helps stabilize glucose levels. 2\. \*\*Hypoglycemia in newborns of undernourished mothers:\*\* \- Reduced glycogen stores in the infant. \- Limited glycogen reserves impair glucose regulation. \- Poor maternal nutrition may hinder gluconeogenesis in the newborn. Individuals with type 1 diabetes have only a small glycogen store in the liver due to the following reasons: Lack of insulin: Type 1 diabetes is characterized by an absence or severe deficiency of insulin, a hormone that promotes glycogen synthesis in the liver. Impaired glycogen storage: Without insulin, the liver cannot efficiently convert glucose into glycogen for storage. Constant glycogen breakdown: In the absence of insulin, the liver is often in a state of increased glycogen breakdown (glycogenolysis), further depleting glycogen stores. Chronic hyperglycemia: High blood glucose levels in untreated or poorly managed type 1 diabetes can prevent proper glycogen storage, as glucose is not effectively taken up by liver cells for conversion into glycogen. TH - Gluconeogenesis ==================== 1. Glucose is stored as glycogen in the **liver**. - - NOTE: The amount of glycogen stored in the liver can provide glucose for 10 to 18 hours of fasting. 2. Gluconeogenesis - - - - - **Blood glucose concentrations at various times after a meal** - - - - Sources of carbon for gluconeogenesis: - - - Importance of blood glucose regulation: - - 3. 4. **Gluconeogenic precursors** - - - - **Gluconeogenesis** - requires that non-glucose precursors need to be mobilised and transported → NOT a rapid response **Lactate** - produced by anaerobic glycolysis in tissues - exercising muscles or RBCs **Glycerol** - released from adipose triacylglycerol. **Amino acids** - from amino acid pool in muscle. 5. **1\] Glycerol** Released from adipose tissue - delivered to the liver (via blood) → glycerol is then converted to **dihydroxyacetone phosphate** (intermediate of glycolysis) Glycerol --(glycerol kinase)→ glycerol-3-phosphate --(glycerol 3 phosphate dehydrogenase)→ **dihydroxyacetone phosphate**  **Gluconeogenesis requires** 1\] a source of energy for biosynthesis and 2\] a source of carbons for the formation of the backbone of glucose - C from: - - - **2\] Amino acids** Glucose from proteins - major source during fast a-keto-acids : such as oxaloacetate and a-ketoglutarate - derived from glucogenic AA - **Lactate dehydrogenase** oxidises lactate to pyruvate generating NADH. **Alanine aminotransferase** converts alanine to pyruvate. NOTE: figure above **3\] Lactate (The Cori cycle)** Found in exercising skeletal muscle cells + cells that lack mitochondria (RBC) - - - **Energy cost** Cori cycle uses 6 high-energy phosphate bonds (liver) for every 2 produced in muscle during anaerobic glycolysis. - **In cancer,** - if blood vessels doesn\'t keep up with tumour needs--? the Cori cycle also activates due to low oxygen, triggering anaerobic glycolysis. - **[The three regulated reactions]** **1\] Pyruvate to phosphoenolpyruvate** **2\] Fructose 1,6-bisphosphate to fructose 6-phosphate** **3\] Glucose 6-phosphate to glucose** NOTE: correspond to the 3 irreversible and regulated steps in glycolysis - BUT use different enzymes 1. 1\] Pyruvate produced by glycolysis enters the mitochondrial matrix. 2\] Pyruvate → oxaloacetic acid (pyruvate carboxylase) - carboxylase - Biotin required 3\] OAA → malate (reduction) - bec OAA cant move through membrane 4\] Malate is transported to the cytosol, Malate → OAA 5\] OAA → phosphoenolpyruvate (phosphoenolpyruvate carboxykinase) -GTP used  **Regulation of reaction - Pyruvate to Phosphoenolpyruvate** - - - - NOTE: **fasting** we need gluconeogenesis - we need **Pyruvate → Phosphoenolpyruvate** **Inactivation of Pyruvate dehydrogenase** - - - - - **Activation of Pyruvate carboxylase** - - - - NOTE: - Opposite reaction happens - At low acetyl CoA levels, **Pyruvate carboxylase** is inhibited and pyruvate is converted to acetyl CoA by PDH for the TCA cycle. **Activation of Phosphoenolpyruvate carboxykinase (PEPCK)** - - - - - **Inactivation of Pyruvate kinase** - - - **Pyruvate Dehydrogenase Complex** The pyruvate dehydrogenase complex is composed of three enzymes: - - - In addition, it is associated with 2 regulatory enzymes: - - Number of coenzymes: thiamine pyrophosphate, lipoic acid and CoA, FAD and NAD+. **Regulation of the pyruvate dehydrogenase complex** PDH - - The reaction is regulated in a number of ways: 1\] product inhibition 2\] Covalent modification by phosphorylation. **Product Inhibition** - **Covalent Modification** - - - **[Gluconeogenesis precursors: Glycerol:]** This is released from triacylglycerols in adipose tissue and delivered by the blood to the liver. (Glycerol → glycerol 3 phosphate via glycerol kinase) (glycerol 3 phosphate → dihydroxyacetone phosphate via Glycerol 3 phosphate dehydrogenase) In the liver glycerol is converted by two reactions to dihydroxyacetone phosphate, an intermediate of glycolysis. 2. Fructose 1,6-bisphosphate → fructose 6-phosphate + inorganic phosphate. (dephosphorylation, via fructose 1,6-bisphosphatase) - - **Fructose 2,6- bisphosphate** is an allosteric effector - signals a well fed state - - - - - - **PFK-2 Dual Activity** - **Glucagon Effect (Fasting)** - - **Insulin Effect (Fed State)** - - **Liver vs. Muscle Regulation** - - 3. Dephosphorylation of glucose 6-phosphate produces free glucose - - - - - NOTE: glucokinase - phosphorylates glucose → glucose uptake - liver and pancreas → glycolysis and glycogen synthesis NOTE: Only liver and kidney can release free glucose from glucose-6-phosphate. - -  NOT FREE FREE **Summary of the reactions of glycolysis and gluconeogenesis,** - **1\]** Gluconeogenesis is not a simple reversal of glycolysis, - **2\]** The gluconeogenic pathway begins in the mitochondrion and ends in the cytoplasm. - **3\]** The carbon skeletons come from **amino acids**, - 6. The synthesis of one molecule of glucose via gluconeogenesis requires 6 ATP molecules. 7. ATP is used in two gluconeogenic reactions: pyruvate carboxylase (converting pyruvate to oxaloacetate) phosphoglycerate kinase (converting 3-phosphoglycerate to 1,3-bisphosphoglycerate) 8. Gluconeogenesis occurs primarily in the cytoplasm, with some steps in the mitochondria and endoplasmic reticulum. 9. - - - 10. 11. 12. **Hormone regulation of the glycolytic pathway and the gluconeogenic pathway.**  → Inc Glucose → Dec Glucose Fasting - inc glucagon → dec fructose 2,6- bisphosphate → dec rate of glycolysis Well fed - inc insulin → inc fructose 2,6- bisphosphate → inc rate of glycolysis **Glucagon** - - **Insulin** - - Insulin level increases after → a high carbohydrate meal. Glucagon level decreases after a high carbohydrate meal. Glucagon level increases after a high protein meal. After a mixed meal (CHO, protein and fat), glucagon remains constant while insulin increases. **Regulation of gluconeogenesis** 1. - - - 2. - 3. - 4. - - **[CONCEPTUAL OVERVIEW ]** 1. 2. 3. 4. 5. 6. 7. 1. Glucose is oxidised to produce energy - - - Insulin stimulates the synthesis of glycogen and the synthesis of triacylglycerols. 2. **Glucose Dependence** - **Insulin Actions (Fig A, B, C)** - - - **Neurons and Glucose** - **Effect of insulin:** 3. - - - The blood glucose level is maintained by - - - - 4.  **1. Hormone Changes** - **2. Liver** - - - **3. Adipose Tissue** - **4. Muscle and RBCs** - - **5. Exercise and Fasting** - 5. Hypoglycemia is defined as a plasma concentration of glucose of 2.5 mmol/l or lower. Most symptoms are caused by the **excess secretion of catecholamines** to stimulate glycogenolysis to maintain blood glucose levels. - Other symptoms may be due to **insufficient glucose** - energy requirements of the brain: - Hormones secreted in response to hypoglycemia include: - **Situations that give rise to hypoglycaemia** A\] Stimulation of rate of glucose utilisation and inhibition of the rate of glucose release due to high insulin levels - - - - B\] Rate of Utilisation of glucose exceeds release of glucose by liver. - - - C\] Low rates of gluconeogenesis - - - 6. Newborns have a critical need for gluconeogenesis as glucose as supply by the placenta is interrupted The genes for gluconeogenic enzymes are stimulated at birth. When this activation does not occur the newborn must be fed glucose solution to prevent hypoglycemia. 7. Type 1 and type 2 diabetes Increased glucagon levels increases rate of gluconeogenesis, increasing blood glucose. Chronic hyperglycemia may cause damage to the retina, lens, kidneys, peripheral nerves, **\#\#\# Child Born of a Malnourished Mother and Hypoglycemia** 1\. \*\*Maternal Nutrient Deficiency\*\*: Malnourished mothers may have inadequate glucose and energy sources during pregnancy. 2\. \*\*Low Glycogen Stores\*\*: The infant may have reduced glycogen stores in the liver due to insufficient nutrient supply. 3\. \*\*Limited Gluconeogenesis\*\*: Decreased substrates for gluconeogenesis in the infant leads to reduced capacity to maintain blood glucose levels after birth. 4\. \*\*Increased Risk of Hypoglycemia\*\*: Postnatally, the infant may experience hypoglycemia due to inadequate energy reserves and impaired metabolic response. **\#\#\# Large Child Born of a Diabetic Mother and Hypoglycemia** 1\. \*\*Maternal Hyperglycemia\*\*: Diabetic mothers often have high blood glucose levels, leading to increased fetal insulin production. 2\. \*\*Fetal Insulin Overproduction\*\*: The fetus compensates for high maternal glucose by producing excess insulin, leading to macrosomia (large size). 3\. \*\*Postnatal Glucose Supply Cut-off\*\*: After birth, the high maternal glucose supply is abruptly cut off. 4\. \*\*Excess Insulin\*\*: The infant\'s elevated insulin levels continue to promote glucose uptake, leading to rapid depletion of blood glucose. 5\. \*\*Resulting Hypoglycemia\*\*: This combination results in hypoglycemia shortly after birth as the infant\'s glucose levels drop rapidly. TH - Pentose phosphate pathway - Fructose and galactose pathway =============================================================== 1. Fructose - -  The metabolism of fructose parallels that of glucose - - Phosphorylation of fructose - fast - as it has a high Vmax Then - degradation → aldolase B → 3 potential products - - 2. Essential fructosuria and Hereditary Fructose intolerance - disorders of fructose - - - Results - - - - less fructose-1-phosphate → hypoglycemia - - NOTE: In hereditary fructose intolerance, sucrose as well as fructose must be removed from the diet to prevent liver failure and possible death. 3. The high levels of F1P inhibit enzymes of glycogenolysis (glycogen phosphorylase) and gluconeogenesis (synthesis of glucose) → leading to hypoglycaemia - - - NOTE: The inhibition of gluconeogenesis results in lactic acidosis as cells undergo anaerobic respiration (without oxygen). 4. Fructose may be synthesised from glucose by the polyol pathway. Glucose → reduced to sorbitol (sugar) → oxidised to fructose - -  5. **[Hyperglycemia and sorbitol accumulation]** Hyperglycemia → glucose enters cells → high amount of glucose in cell → glucose converted to sorbitol → sorbitol cannot pass across membrane → increase in oncotic pressure inside cell → water retention and swelling Thus diabetes → Cataract formation Enz - Aldose reductase Sorbitol accumulation → lens, retina, Schwann cells liver, kidney , placenta, ovaries, seminal vesicles, RBCs,. NOTE: liver, seminal vesicles and ovaries - have a 2nd enz that converts sorbitol to fructose (Sorbitol dehydrogenase) - -  6. In patients with classical galactosemia, uridyltransferase is deficient.  - 7. The pentose phosphate pathway provides: - - There are 2 parts to the pentose phosphate pathway: - - 8. **Ribose-5-phosphate** may be used to produce the glycolytic intermediates, (glycolysis) - - **In oxidative phase** - - - NOTE: In this phase two molecules of NADP+ are reduced to NADPH. 9. Pathways that require **NADPH** **Detoxification** NADPH provides reducing power to neutralise harmful reactive oxygen species, aiding in cellular detoxification. - - **Reductive Synthesis** - - - - - 10.  The RBC protein cytoskeleton requires **ATP** and **NADH** for stability and function. NADPH, produced by the **pentose phosphate pathway**, is essential for reducing glutathione disulfide (**GSSG**) to reduced glutathione (**GSH**). - **Haemolytic stress -** In healthy RBCs, **nonenzymatic generation of superoxide** from haemoglobin oxygen produces a source of reactive oxygen species (ROS). **Mechanical stress -- Heinz bodies** are aggregates of cross-linked haemoglobin that form on the RBC membrane, causing mechanical stress as they pass through small capillaries. → Mechanical stress combined with ROS leads to hemolysis of the RBC. **Methemoglobin (Met-Hb)** is a form of haemoglobin - - 11. 12. **The respiratory burst** - - - **Synthesis of nitric oxide** Arginine, molecular oxygen and NADPH are substrates for cytosolic nitric oxide. - - - - Glucose-6-phosphate dehydrogenase deficiency - - - - - - - - Factors that precipitate haemolytic anemia in G6PD deficiency - - - NOTE: Babies with G6PD deficiency may exhibit neonatal jaundice because of an increased production of bilirubin. Give the metabolic explanation for the hypoglycaemia, lactic acidosis and hyperuricemia observed in patients suffering from hereditary fructose intolerance **Metabolic Explanation for Symptoms in Hereditary Fructose Intolerance (HFI)** **Hypoglycemia:** Fructose-1-phosphate accumulates, trapping phosphate (Pi), reducing ATP production. Low ATP inhibits glucose production pathways, leading to hypoglycemia. **Lactic Acidosis:** ATP depletion reduces gluconeogenesis, shunting pyruvate to lactate. Excess fructose-1-phosphate drives glycolysis, increasing lactate. **Hyperuricemia:** Low ATP triggers adenine nucleotide breakdown, increasing uric acid. Phosphate trapping also activates AMP breakdown to uric acid. Explain why essential fructosuria is benign while hereditary fructose intolerance can be fatal. **Essential Fructosuria vs. Hereditary Fructose Intolerance** **Essential Fructosuria:** Caused by fructokinase deficiency, leading to benign fructose excretion in urine. No toxic metabolites; does not affect ATP or phosphate levels. **Hereditary Fructose Intolerance:** Caused by aldolase B deficiency, leading to toxic fructose-1-phosphate buildup. Disrupts ATP and phosphate balance, causing severe symptoms and potential fatality. Fatty acid oxidation Ketone bodies ================================== 1. 2. 3. **Release of fatty acids from triacylglycerols** **Lipases** - used to release fatty acids from triacylglycerols - **Activity of this lipase is regulated by hormones - epinephrine and glucagon** - - - - - **Transport of Fatty acids till they enter the cell cytoplasm** - - - - 4. **The fate of glycerol and fatty acids** - - - - - **Fatty acid facts** - - - - - - - **Metabolic pathways for long chain fatty acids** -  5. **Breakdown of Fatty acids** Catabolic pathway - B-oxidation - - - Once inside the cell, fatty acids are converted to CoA derivatives. - - Thus - LCFA are transported from the cytosol to the mit matrix via special carrier - 6. The carnitine shuttle [transports long-chain fatty acids into the mitochondrial matrix] by converting them into acyl-carnitine derivatives, - Mechanism - BUT cannot cross the Inner mitochondrial membrane (impermeable to CoA) - - - 2 IMP enzymes - - **Regulation of the carnitine shuttle** The carnitine shuttle is inhibited by malonyl CoA - - **Inherited disorders involving carnitine** The classical condition is CPT II deficiency. - - - - - CPT II Deficiency Overview - - NOTE: **1.The carnitine shuttle** is a transport system that moves long-chain fatty acids into the mitochondria for beta oxidation, (enzymes carnitine palmitoyltransferase I (CPT I), carnitine-acylcarnitine translocase, and CPT II.) 2\. **Beta oxidation**: The process of breaking down fatty acids in the mitochondria to produce acetyl-CoA, NADH, and FADH₂ for energy. 3\. **Ketogenesis**: The metabolic pathway in the liver that produces ketone bodies from acetyl-CoA during periods of low glucose availability. **4. Ketone bodies:** Water-soluble molecules (acetoacetate, beta-hydroxybutyrate, and acetone) produced during fat metabolism as an alternative energy source. 7. **B-oxidation** A sequence of 4 reactions result in shortening the fatty acid chain by two carbons. - First cycle of fatty acid oxidation - - - These steps are repeated for saturated fatty acids of even numbered carbon chains - - NOTE: The final reaction of the last cycle - **Overview of beta-oxidation** [Breakdown of fatty acids to form acetyl CoA] Oxidation of the beta carbon - then cleavage of the alpha beta bond → releasing acetyl CoA and fatty acyl (2C shorter than the original) Successive spirals of beta oxidation completely cleave an even chain fatty acyl CoA to acetyl CoA. **Medium chain length fatty acids** - - - - note - the reaction occurs 7 times and 8 molecules of acetyl co A produced note - -2 ATP bec these are used in the initial step to attach coA 8. 9. The beta oxidation of of a molecule of palmitoyl CoA to CO2 and H2O produces - - - - The initial activation of palmitate to palmitoyl CoA requires 2 ATP. - 10.  **Odd chain fatty acids** The propionyl CoA is converted in a multistep manner to succinyl CoA by a vitamin B12 dependent reaction. - Note: anaplerotic route - replenishes intermediates of the citric acid cycle - - **Explain how fatty acid oxidation is regulated.** **Hormonal control of fatty acid oxidation** 1\] **hormone sensitive lipase** fatty acid oxidation → regulated by insulin/glucagon/ epinephrine - Active lipoprotein lipase → hydrolysis of triacylglycerides - produce free fatty acids and glycerol - - Malonyl CoA - inhibits carnitine shuttle - thus prevents oxidation of newly formed fatty acids Beta Oxidation (How fatty acids are broken down → acetyl co A, NADH and FADH2) **[Regulation of Beta oxidation]** regulated → By cells energy requirements (ATP and NADH in the blood) 1\] **Hormones** in the blood 2\] acetyl CoA carboxylase - produces **malonyl CoA** - inhibits carnitine palmitoyl transferase NOTE: ATP utilisation - determines rate of ETC - determines B oxidation (oxidative enzymes) + TCA **peroxisomal B oxidation** - - **Ketone bodies** In liver - B oxidation → acetyl CoA - - - - - - Note - Ketone bodies can also be produced from AA - - - **Role of fatty acids and ketone bodies in fuel homeostasis** - - - - - - Ketonaemia: high ketone bodies in blood Ketonuria: high ketone bodies in urine Ketosis: the overall condition of high ketones is called ketosis. - **Oxidation of ketone bodies** - - - - **Regulation of ketone oxidation** - - Thus - when energy is high - (high NADH/NAD, acetyl CoA, ATP/ADP ratios) - - - Thus high rate of ketone oxidation has the above effects  Fasting - insulin/glucagon - low - glucose low and we want to use it - - - - Fatty acid and triacyglyceride synthesis ======================================== **Objectives** 1. 2. **Fatty acid synthesis** - - - Fatty acid syn - carried out in 2 cellular compartments - - Acetyl CoA - precursor - found in mit - must be transferred to cytoplasm Fatty acids for oxidation - must be transported to mit matrix Note: PDH - only in mit → thus in cytosol pyruvate -X-\> acetyl CoA - 3. Acetyl CoA carboxylase (ACC) catalyzes carboxylation reaction imp in fatty acid syn - - **When active** - **fatty acid syn** - **increases triacylglycerol storage in adipose** **When inactive - fatty acid oxidation - increase fatty acid release in blood** Malonyl CoA (3C) reacts with acetyl CoA (2C) , elongating the chain to 4C and releasing CO₂. → this cycle is repeated 7 times → 16 C fatty acid (palmitic acid) - - - -   **Desaturation of Fatty Acids**: Enzymes in the ER introduce cis double bonds into fatty acids (desaturation). Humans have desaturases at carbon 9, 6, 5, and 4, but cannot introduce double bonds beyond carbon 10, omega end. This is why linoleic and linolenic acids are essential fatty acids in the diet. 4. **[Regulation of acetyl CoA carboxylase ACC: short-term]** **Acetyl CoA carboxylase ACC** - **Well fed state - activates - ACC** **Fasting state - inactivates -ACC** +-----------------------------------------------------------------------+ | **Citrate** → allosterically activates protomers, | | | | - | +=======================================================================+ | **Long-chain fatty acyl-CoA** inhibits ACC by causing | | depolymerization. | +-----------------------------------------------------------------------+ | **AMPK** (Adenosine Monophosphate-Activated Kinase) | | | | - - - | +-----------------------------------------------------------------------+ | **Insulin** | | | | - - | +-----------------------------------------------------------------------+ | **Palmitate** | | | | - - | +-----------------------------------------------------------------------+ | **Diet: long term regulation** | | | | - | +-----------------------------------------------------------------------+ **[Regulation of beta oxidation]**  **[B - oxidation]** **Regulated by cell energy needs (levels of ATP and NADH)** **Activated fasting state** **Inhibited - well fed state** **1\] Hormonal regulation** - **2\] Carnitine Palmitoyltransferase I (CPT1):** - - **3\] AMP-activated Protein Kinase (AMPK):** - **4\] ATP and NADH Levels:** - - - 5. **Triacylglycerol (TAG) Synthesis**: Fatty acids are stored as triacylglycerols (TAG) in the body. One, two, or three molecules of fatty acids are esterified to a molecule of glycerol. - - - **Properties of TAG:** - - Synthesis of triacylglycerols - - - - 6. **Production of glycerol phosphate in liver and adipose tissue** - 2 pathways for glycerol phosphate production **From glucose**: In both liver and adipose tissue (during glycolysis) - **Liver** - - **Adipose tissue** - - - - Both **liver** and **adipose tissue**: Glyceraldehyde-3-phosphate (G3P) from glycolysis is converted to dihydroxyacetone phosphate (DHAP), - then reduced by glycerol-P-dehydrogenase to glycerol phosphate for TAG synthesis.  7. **Mode of Action of Metformin:** 1. 2. 3. 4. Overall, it lowers blood glucose by reducing production and enhancing utilization. Alcohol metabolism ================== **Ethanol - Properties** - - - - XS alcohol intake → liver disease: alcoholic hepatitis, steatosis due to fat deposition and cirrhosis )\--\> liver failure **[Major Pathways of Alcohol Metabolism: (oxidative)]** 2 pathways - 1. 2. **1\] Alcohol Dehydrogenase (ADH) Pathway:** - - - - Note: Hepatic ADH - cytosol, ALDH - mit Note: Acetaldehyde is toxic and may enter the blood. Note: ATP produced from NADH (oxidative phosphorylation.) **ADH: alcohol dehydrogenase.** **ALDH: acetaldehyde dehydrogenase.** **ACS: acetyl CoA synthetase** **2\] Microsomal Ethanol Oxidizing System (MEOS):** Involves cytochrome P450 enzymes, primarily CYP2E1, - -  Alcohol dehydrogenase (ADH), acetaldehyde dehydrogenase (ALDH), and MEOS Enzymes exist as **isozymes**. - At low ethanol concs, ADH is most imp due to its lower Km compared to MEOS. Note: even if no alcohol is ingested - colon bacteria produce little amount by microbial fermentation\ **[1\] Alcohol Dehydrogenase (ADH) Pathway:]**  **Alcohol Dehydrogenase (ADH) enzyme:** - - - - - **Acetaldehyde** - - - - - **Acetaldehyde dehydrogenase (ALDH) enzyme:** - - - - - Acetaldehyde accumulates due to the slow regeneration of cytoplasmic NAD+. - **Acetaldehyde Dehydrogenase Variant (ALDH2\*2):** [Reduced Acetaldehyde Metabolism:] - [Symptoms and Effects]: Acetaldehyde accumulation → nausea and vomiting, leading to a distaste for alcohol. - **Alcoholism treatment → ALDH Inhibitors (Disulfiram):** - - **Acetate** - - - - - **[2\] Microsomal ethanol oxidising system (MEOS)]**  **[MEOS]** Member of the cytochrome P450 superfamily - **CYP2E1** - - - - - This route - for 10 to 20 % of the alcohol consumed by a moderate drinker. - - Note: Again -variation in isoenzymes →variation in susceptibility to development of alcohol induced liver disease. **Induction of P450 enzymes - (CYP2E1 )** [High alcohol levels → induce CYP2E1 10-fold.] Induction occurs at the level of - - - Enzymes and mRNA are stabilized and resistant to degradation in heavy drinkers. Negative consequences of induction: - - **Induction of P450 enzymes - (CYP2B2)** High levels of Phenobarbital → induces high level of - P450 enzyme CYP2B2 CYP2B2 inactivates phenobarbital - Leads to drug tolerance. - Note: if a lot of ethanol and phenobarbital are ingested - levels may accumulate to toxic levels **[Overall facts of alcohol metabolism]** **Factors Affecting Ethanol Metabolism:** **Genotype**: Polymorphisms in ADH/ALDH affect metabolism rate. **Drinking History**: Chronic drinking decreases gastric ADH and increases CYP2E1, raising acetaldehyde and free radicals. **Gender**: Women have lower gastric ADH and higher blood alcohol levels. **Ethanol Quantity**: - - - **Health Impact**: Affects risk of alcoholism and alcohol-related cancers (liver, lung, breast). **Alcohol induced liver disease** - - - **The acute effects of ethanol on health arise from:** 1\] Increased NADH/NAD+ ratio 1. 2. 3. 4. 5. 2\] Acetaldehyde toxicity **1\] Increased NADH/NAD+ ratio** 1. - - - 2. - - - - 3. - - - **[Toxic effects of ethanol]** Effects of Ethanol on Metabolism: 1. - 2. - 3. - 4. - 5. - 6. - 7. - **Acetaldehyde and Hepatitis**: - - - **Acetaldehyde and Free Radical Damage**: - - - - **Development of alcohol induced hepatitis**  1. 2. 3. 4. 5. 6. 1. 2. 3. 4. 5. 6. 7. 8. 9. 10. 11. 12. Amino Acid metabolism: Transamination and Urea Cycle ==================================================== Overview - - - - - **[Digestion of protein to amino acids]** 1\] Stomach - acid → denatures + pepsin 2\] Pancreatic juice → trypsin, chymotrypsin, elastase and carboxypeptidase **[Absorption of amino acids]** - - - **[Fate of amino acid carbon and nitrogen]** AA **carbons** used - - AA **nitrogen** used - - AAs travel around different tissues during metabolism **The amino acid nitrogen is transferred to urea in 3 steps:** 1. 2. 3. **1\]Transamination reactions** - - - - - Transamination pairs - - **[2\]Formation of ammonia]** - - - -  **Other sources of ammonia for the urea cycle** 1. 2. 3. 4. 5. 6. 7. **Role of glutamate in the metabolism of amino acid nitrogen** - **Glutamate role in production** **Urea - needs 2 Nitrogens** - - **Transport of Nitrogen - via Glutamine and Alanine** **The glucose/alanine cycle** - - **1\] Glutamine** In most tissues: **glutamate + ammonia → glutamine** - Then - Glutamine acts as a carrier of ammonia in the blood. In the liver - **glutamine** → **[NH4+]** + **glutamate** - - **2\] Alanine** In muscle: **Pyruvate + amino gps → alanine** - - In liver: **alanine + a-ketoglutarate → glutamate + pyruvate** - The alanine that travels to liver is used as a gluconeogenic precursor. Note: Protein degradation in muscle cells leads to the transfer of nitrogens to alpha ketoglutarate and pyruvate. **[3\]The formation of urea.]** - - - **Urea cycle** **Function** - Converts toxic ammonia into nontoxic urea for excretion. **Sources:** **Ammonia**: From amino acid metabolism, purine metabolism, and intestinal bacteria **Aspartate**: Provides the second nitrogen in the urea molecule. **Hyperammonemia** Definition: Elevated blood ammonia levels due to impaired liver function. Effects: Toxic to CNS: Causes acute encephalopathy. Mechanisms: - - - Indicator: Measured as BUN (blood urea nitrogen). **Urea cycle** - Steps 1. 2. 3. 4. 5.  **Regulation of the Urea Cycle:** **Short-term - After high-protein meal:** - - - **Long-term - During starvation:** - - **Overview of urea** 1. 2. 3. 4. 5. 6. 1. 2. 3. 4. 5. 6. 7. 8. 9. 10. 11. 12. Amino acid carbon skeleton ========================== **Amino acids** - - **The 7 intermediates of amino acid degradation** +-----------------------------------+-----------------------------------+ | 1. 2. 3. 4. 5. | 6. 7. | +-----------------------------------+-----------------------------------+ **Glucogenic amino acids** - produce pyruvate or other TCA intermediates. - - **Ketogenic amino acids -** produce acetoacetate or acetyl CoA. - **[Metabolic intermediates formed from amino acids degradation]** 1. 2. 3. 4. 5. 6.  **[1\] Oxaloacetate: Asparagine and Aspartate]** Reaction: **Asparagine → aspartate → oxaloacetate** - - Clinical note Some leukemia cells cannot syn enough asparagine, - - Note - **Asparagine → aspartate** links to urea lecture - **[2\] a-Ketoglutarate: Glutamine, Proline, Arginine, Histidine]** **Glutamine: Glutamine → glutamate → a-ketoglutarate** - - - **Proline: proline → glutamate → a-ketoglutarate** - **Arginine: arginine → ornithine → oxaloacetate → a-ketoglutarate** - - **Histidine: Histidine → FIGLU → Glutamate** - - - - **[3 \]Pyruvate: Alanine, Serine, Glycine, Cysteine, Threonine]** **Alanine: alanine → pyruvate** - **Serine: serine → pyruvate** - **Glycine: glycine → serine → pyruvate** -  **[4\] Fumarate and Acetoacetate: Phenylalanine and Tyrosine]** Phenylalanine and tyrosine are both glucogenic and ketogenic. **Tyrosine: tyrosine → fumarate and acetoacetate** - **Phenylalanine: Phenylalanine → tyrosine → fumarate and acetoacetate** - Inherited deficiencies in enzymes of phenylalanine and tyrosine metabolism lead to diseases such as **phenylketonuria (PKU)** and **alkaptonuria** **[5\] Succinyl CoA: methionine]** **Methionine: Methionine → S-adenosylmethionine (SAM) → homocysteine** - Imp - SAM - methyl gp donor of methyl groups in the synthesis of hormones, nucleotides and membrane lipids. **Homocystinuria** - - **[6\] Acetyl CoA]** Leucine and lysine are exclusively ketogenic. - - Note: Isoleucine and tryptophan are both ketogenic and glucogenic. **Key Points About Amino Acid Degradation** - - - **[Amino Acid Metabolism in Different States]** **Fed State:** Dietary amino acids are converted into - - - - **Starvation:** - - - **Hypercatabolic State (Trauma/Surgery/Cancer):** - - - **[Amino Acid metabolism in the brain]** **Neurotransmitters:** Glycine, glutamate, aspartate. **Precursors for neurotransmitters:** - - - - Note: Aspartate and glutamate are synthesized in the brain. **Glutamine:** Part of the amino acid shuttle maintaining glutamate in presynaptic terminals. **The glutamine/glutamate shuttle in brain** **Glutamate Neurotransmitter Cycle (Point Form):** - - - - - -  1. - - 2. - - 3. - 4. - 5. - 6. - 7. - 8. - 9. - 10. - 11. - 12. - 13. - Biosynthesis of amino acids and amino acid derivatives ====================================================== **Overview** List of **Non essential amino acids** - **How are non essential amino acids synthesized** - - - - - - - - - The carbons of 10 nonessential AA from glucose (intermediates of glycolysis) - - **[Synthesis of amino acids from:]** **[1\] Amino acids produced by transamination: ]** - Transamination reactions **[2\] From glycolytic intermediates of TCA, from: a-ketoglutarate]** - **Glutamate:** From a ketoglutarate (transamination) - - - - -  **Note: Alpha-ketoglutarate → malate (gluconeogenesis) → glucose.** **Note 2: Histidine to a-ketoglutarate from carbon skeleton notes**  **Histidase** is an enzyme deficient in **histidinemia.** Common symptoms of histidinemia include hyperactivity, speech impediment, developmental delay, learning difficulties, and sometimes mental retardation. **[3\] From glycolytic intermediates of TCA, from: Oxaloacetate]** - **Aspartate:** from oxaloacetate (transamination) - **[Inborn errors of metabolism]** - - - - -  Pathologies: - - - - - - +-----------------------------------+-----------------------------------+ | | **[1\] Phenylketonuria | | | (PKU)]** | +===================================+===================================+ | **Deficiency** | Autosomal recessive | | | | | | **Phenylalanine hydroxylase** | | | (enzyme) | | | | | | Tetrahydrobiopterin (BH4) - | | | coenzyme (2\^ PKU) (in some | | | cases) | +-----------------------------------+-----------------------------------+ | **Normal function** | Phenylalanine hydroxylase: | | | converts phenylalanine → tyrosine | | | | | | In amino acid metabolism. | | | | | | Tyrosine imp in syn of → | | | neurotransmitters (dopamine, | | | epinephrine) and melanin. | +-----------------------------------+-----------------------------------+ | **Result of Deficiency** | Accumulation of phenylalanine in | | | blood and tissues. | | | | | | - | | | | | | Elevated phenylpyruvate and its | | | derivatives | | | | | | Reduced tyrosine levels → | | | deficient neurotransmitter and | | | melanin production. | | | | | | Phenylalanine - detected in | | | blood, (tissues and urine) | | | | | | Phenylpyruvate - detected in | | | urine - musty mousy smell | +-----------------------------------+-----------------------------------+ | **Symptoms** | Mental retardation (NT) | | | | | | Hypopigmentation (melanin) | +-----------------------------------+-----------------------------------+  +-----------------------------------+-----------------------------------+ | | **[2\] Maple Syrup Urine Disease | | | (MSUD)]** | +===================================+===================================+ | **Deficiency** | Autosomal recessive, rare | | | | | | **Branched-chain α-keto acid | | | dehydrogenase (BCKD)** complex | +-----------------------------------+-----------------------------------+ | **Normal function** | Catalyzes the decarboxylation of | | | (**leucine, isoleucine, | | | valine**): | | | | | | - | | | | | | Enables energy production and | | | synthesis of other molecules. | +-----------------------------------+-----------------------------------+ | **Result of Deficiency** | Accumulation of branched-chain | | | amino acids and their toxic | | | α-keto acids | | | | | | - | | | | | | Impaired energy metabolism from | | | these amino acids. | +-----------------------------------+-----------------------------------+ | **Symptoms** | Feeding problems, dehydration, | | | vomiting, metabolic acidosis, dec | | | muscle tone, coma. | | | | | | - | | | | | | Treatment: a synthetic amino acid | | | preparation with limited amounts | | | of these AA | | | | | | - | +-----------------------------------+-----------------------------------+ +-----------------------------------+-----------------------------------+ | | **[3\] Albinism]** | +===================================+===================================+ | **Deficiency** | **Tyrosinase enzyme** | | | | | | Note: this gives complete | | | albinism, there are other | | | incomplete types | | | | | | - | +-----------------------------------+-----------------------------------+ | **Normal function** | Catalyzes: tyrosine → melanin, | | | | | | - | +-----------------------------------+-----------------------------------+ | **Result of Deficiency** | Absence or significant reduction | | | in melanin production. | | | | | | Accumulation of tyrosine without | | | effective conversion to melanin. | +-----------------------------------+-----------------------------------+ | **Symptoms** | Hypopigmentation of skin, hair, | | | and eyes. | | | | | | Increased sensitivity to UV | | | radiation leading to sunburn and | | | higher skin cancer risk. | | | | | | Vision problems, including | | | nystagmus, photophobia, and | | | reduced visual acuity. | +-----------------------------------+-----------------------------------+ Note: Separate tyrosine enzyme used in brain tyrosine hydroxylase (DOPA-producing enzyme → syn of catecholamine neurotransmitters,) - this is not effects - thus albinos usually don\'t have mental issues - -  +-----------------------------------+-----------------------------------+ | | **[4\] Tetrahydrobiopterin | | | metabolism | | | deficiency]** | +===================================+===================================+ | **Deficiency** | **Tetrahydrobiopterin (BH4)** - | | | coenzyme | +-----------------------------------+-----------------------------------+ | **Normal function** | Tetrahydrobiopterin is a coenzyme | | | for the synthesis of various AA | | | derivatives: | | | | | | - | | | | | | Phenylalanine → Tyrosine. | | | | | | Tyrosine → DOPA (precursor for | | | dopamine, norepinephrine, and | | | epinephrine). | | | | | | Tryptophan → 5-hydroxytryptophan | | | (precursor for serotonin). | +-----------------------------------+-----------------------------------+ | **Result of Deficiency** | Accumulation of phenylalanine | | | (similar to classical PKU). | | | | | | Impaired synthesis of | | | neurotransmitters (dopamine, | | | serotonin, norepinephrine). | +-----------------------------------+-----------------------------------+ | **Symptoms** | Severe CNS symptoms: | | | developmental delay, intellectual | | | disability, seizures. | | |