Vectors for Gene Expression in Mammalian Cells PDF
Document Details
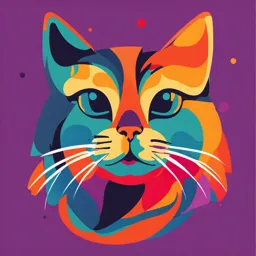
Uploaded by WinningHoneysuckle
UCLan
Dr Temba Mudariki
Tags
Summary
This document provides an overview of vectors for gene expression in mammalian cells. It covers learning outcomes, definitions, functions, and different types of vectors. The document details specific aspects, such as viral and non-viral vectors, and their mechanisms of delivery.
Full Transcript
Vectors for Gene Expression in Mammalian Cells Dr Temba Mudariki Learning Outcomes Define vectors for gene expression in mammalian cells and explain their role in molecular biology. Identify the main components of vectors for gene expression, including promoters, coding sequ...
Vectors for Gene Expression in Mammalian Cells Dr Temba Mudariki Learning Outcomes Define vectors for gene expression in mammalian cells and explain their role in molecular biology. Identify the main components of vectors for gene expression, including promoters, coding sequences, and selection markers. Understand the significance of vectors in facilitating the expression of foreign genes in mammalian cells. Describe the different types of vectors used for gene expression in mammalian cells, such as viral vectors (e.g., lentiviral, adenoviral) and non-viral vectors (e.g., plasmids). Explain the mechanisms by which vectors deliver genes into mammalian cells, including viral- mediated transduction and non-viral methods (e.g., lipofection, electroporation). Learning Outcomes Recognize the advantages and disadvantages of different vector types for gene expression in mammalian cells. Analyse the regulatory elements within vectors that control gene expression, such as enhancers and terminators. Evaluate the factors influencing the choice of vector for specific gene expression experiments, including cell type, gene size, and desired expression level. Understand the importance of selection markers in identifying cells that have successfully incorporated and expressed the gene of interest. Apply knowledge of vectors for gene expression in mammalian cells to design and construct appropriate vectors for specific research or biotechnological applications. Introduction to Vectors for Gene Expression Definition and Role of Vectors in Molecular Biology Vectors in Molecular Biology DNA molecules used to carry and deliver foreign DNA sequences into host cells. Crucial in genetic engineering and gene expression studies. Function of Vectors Serve as vehicles for transporting foreign DNA into host cells. Act as carriers for efficient delivery of desired DNA sequences. Contain specific genetic elements for replication, propagation, and expression in host cells. Importance of Vectors for Gene Expression in Mammalian Cells DNA Delivery 1. Vectors efficiently deliver foreign DNA into mammalian cells. 2. Overcome the challenge of introducing DNA directly into cells due to complex cellular membranes. 3. Utilize specific mechanisms like viral-based vectors or non-viral delivery systems. Regulatory Elements 1. Vectors contain promoters, enhancers, and transcription termination signals to ensure proper gene expression. 2. Promoters initiate gene transcription, while enhancers modulate promoter activity. 3. Inclusion of appropriate regulatory elements allows for precise control of gene expression levels and cell-specific expression. Importance of Vectors for Gene Expression in Mammalian Cells Selection Markers 1. Vectors incorporate selectable markers like antibiotic resistance genes or fluorescent proteins. 2. Enable identification and selection of cells that have taken up the vector and expressed the desired gene. 3. Provide a way to distinguish between integrated and non-integrated cells, allowing for isolation and enrichment of cells with desired gene expression. Expression Systems 1. Vectors can be designed to work with specific expression systems in mammalian cells. 2. Engineered for inducible expression systems, allowing precise temporal and spatial control of gene expression through the addition or removal of specific inducers. Importance of Vectors for Gene Expression in Mammalian Cells Versatility 1. Offer versatility in expressing different sizes and types of DNA sequences in mammalian cells. 2. Accommodate small genes, large genes, multiple genes in the same vector, or entire gene clusters. 3. Enable the study of complex biological processes, investigation of gene function, and development of gene therapies. Components of Vectors for Gene Expression Promoters: Importance and Types Crucial Role Determine when and where a gene is expressed. Located upstream of the coding sequence. Serve as binding sites for RNA polymerase, initiating transcription. Importance Regulate gene expression levels and direct expression in specific cell types or conditions. Varying strengths influence mRNA and protein expression levels. Strong promoters generate high mRNA and protein levels, while weak promoters result in lower expression. Types of Promoters Used in Vector Design Constitutive Promoters 1. Drive gene expression consistently and at relatively high levels in most cell types. 2. Examples: CMV (cytomegalovirus) promoter, SV40 (simian virus 40) promoter. 3. Commonly used for constant expression in basic research or when generating stable cell lines. Tissue-Specific Promoters 1. Drive gene expression in specific cell types or tissues. 2. Derived from genes normally expressed in those specific cells. 3. Allow targeted expression, useful in gene therapy or developmental biology studies. Inducible Promoters 1. Allow control over gene expression by responding to specific inducers or environmental stimuli. 2. Regulate gene expression based on the presence or absence of the inducer. 3. Examples: tetracycline-inducible promoter (Tet-On/Tet-Off system), ecdysone-inducible promoter. 4. Offer temporal and spatial control over gene expression, facilitating studies requiring precise regulation or dynamic expression patterns. Coding Sequences: Designing for Efficient Gene Expression Codon Usage 1. Refers to the frequency of specific codons in the DNA sequence. 2. Using host organism-preferred codons enhances translation efficiency. 3. Optimizing codon usage can improve protein expression levels. mRNA Stability 1. mRNA stability impacts protein expression levels. 2. Designing stable secondary structures or avoiding degradation-prone sequences increases mRNA stability and enhances protein expression. Kozak Sequence 1. Specific sequence surrounding the start codon (usually AUG) that enhances translation initiation. 2. Including an optimized Kozak sequence improves translation efficiency and protein expression levels. Fusion Tags 1. Added to aid in protein purification, detection, or localization. 2. Common fusion tags: polyhistidine (His-tag), glutathione S-transferase (GST), green fluorescent protein (GFP). Selection Markers: Identifying Transformed Cells Purpose Enable identification and selection of cells that have taken up the vector and expressed the gene of interest. Distinguish transformed cells from non-transformed cells or those that have not integrated the vector. Commonly Used Selection Markers Antibiotic Resistance Genes 1. Encode proteins providing resistance to specific antibiotics (e.g., kanamycin, ampicillin, neomycin). 2. Transformed cells expressing these genes survive in the presence of corresponding antibiotics, while non-transformed cells are eliminated. Fluorescent Proteins 1. Genes encoding fluorescent proteins like green fluorescent protein (GFP) serve as selection markers. 2. Cells expressing fluorescent proteins can be easily identified and sorted using fluorescence-activated cell sorting (FACS) or fluorescence microscopy. Reporter Genes 1. Encode enzymes (e.g., β-galactosidase, luciferase) producing detectable signals like colour change or luminescence when expressed. 2. Enable visualization or quantification of gene expression in transformed cells. Types of Vectors for Gene Expression Viral Vectors for Gene Expression Definition Derived from viruses and extensively used in gene expression studies and gene therapy applications. Efficient delivery of genetic material into host cells due to their natural cell- infecting ability. Common Types Lentiviral Vectors Widely used for their ability to infect both dividing and non-dividing cells. Adenoviral Vectors Known for their high transduction efficiency and ability to carry large DNA inserts. Lentiviral Vectors: Features and Applications Derived from lentiviruses, capable of infecting both dividing and non- dividing cells. Integration into host genome Results in stable, long-term gene expression. Large cargo capacity Can accommodate large DNA sequences, including full-length genes or gene clusters. Applications Gene therapy for inherited disorders, cancer therapies, and genetic diseases. Transgenic animal production. Basic research for studying gene function, regulation, and protein expression in various cell types. Lentiviral Vectors: Features and Applications Adenoviral Vectors: Features and Applications Derived from adenoviruses, efficient in infecting dividing cells but have limited infectivity in non-dividing cells. High transduction efficiency Suitable for delivering genes into a wide range of cell types. Transient gene expression Usually do not integrate into the host genome, resulting in transient gene expression. Applications Vaccine development to stimulate an immune response against pathogens or tumour cells. Gene therapy, particularly in cancer treatments and genetic disorders affecting non-dividing cells. In vivo gene delivery for delivering genes to specific tissues or organs in gene therapy. Non-Viral Vectors for Gene Expression Non-viral vectors are DNA-based delivery systems that do not involve viral components. They offer advantages such as safety, ease of production, and low immunogenicity. Plasmid Vectors: Features and Applications Definition: Circular DNA molecules capable of independent replication within host cells, widely used in molecular biology and gene expression studies. Features and Applications Easy manipulation and production: Easily manipulated in the laboratory and produced in large quantities using bacterial culture systems. Transient gene expression: Typically do not integrate into the host genome, resulting in transient gene expression. Gene therapy applications: Explored in gene therapy approaches, particularly for ex vivo gene therapy. DNA vaccines: Used to deliver specific genes encoding antigens for stimulating an immune response against pathogens. Gene knockout studies: Used for gene knockout studies by delivering CRISPR/Cas9 components or other gene-editing tools to target specific genes. Other Non-Viral Vector Systems Apart from plasmid vectors, several other non-viral vector systems have been developed for gene expression. These include: Lipid-based vectors Composed of lipid bilayers and can efficiently deliver DNA into cells. Used in gene therapy and drug delivery applications. Polymer-based vectors Offer efficient DNA delivery and can be modified for improved targeting or controlled release of genes. Examples include polyethylenimine (PEI) and poly(lactic-co-glycolic acid) (PLGA). Nanoparticle-based vectors Nanoparticles functionalized with DNA used for gene delivery, imaging, or theranostic applications. Examples include gold nanoparticles or quantum dots. Electroporation Involves the application of electric pulses to cells, creating temporary pores in the cell membrane, allowing DNA to enter the cells. Widely used non-viral method for gene delivery, particularly in research laboratories. Viral-Mediated Transduction Overview of Viral Vector Transduction Mechanisms Utilize the natural ability of viruses to infect cells and deliver genetic material. Steps in Transduction Mechanisms Cell attachment: Viral vector binds to specific receptors on the cell surface, facilitating its entry into the target cell. Internalization: Taken up by the cell through mechanisms like receptor-mediated endocytosis or direct fusion with the cell membrane. Escape from endosomes: If internalized via endocytosis, some viral vectors need to escape from endosomes to avoid degradation in lysosomes, facilitated by pH-sensitive viral proteins or viral proteins that disrupt endosomal membranes. Nuclear localization: Viral vector or its genetic material must reach the cell nucleus for gene expression, facilitated by nuclear localization signals. Gene expression: Genetic material is released and can integrate into the host genome (for integrating viral vectors) or undergo transcription and translation to produce the desired protein (for non-integrating viral vectors). Advantages and Limitations of Viral-Mediated Gene Delivery Advantages of viral-mediated gene delivery include: High transduction efficiency: Viral vectors have evolved to efficiently infect and deliver genes into host cells, resulting in high transduction efficiency. Stable and long-term gene expression: Integrating viral vectors, such as lentiviral vectors, can integrate their genetic material into the host genome, leading to stable and long-term gene expression. Cell type specificity: Some viral vectors can be engineered to target specific cell types by modifying their surface proteins or using cell-specific promoters. Large cargo capacity: Viral vectors can accommodate relatively large DNA sequences, allowing the delivery of full-length genes or gene clusters. Limitations of Viral-Mediated Gene Delivery Immunogenicity Viral vectors can trigger immune responses in the host, potentially leading to inflammation or immune rejection. Safety Concerns Risk of insertional mutagenesis, where the integration of viral DNA into the host genome can disrupt normal gene function, potentially leading to adverse effects. Limited Cargo Capacity Although relatively large compared to non-viral vectors, viral vectors are still limited in the size of DNA they can carry. Manufacturing Challenges More challenging and costly to produce at large scales compared to non-viral vectors. Non-Viral Methods of Gene Delivery Lipofection: Principles and Applications Principles Formation of lipid-DNA complexes: Cationic lipids interact with DNA, forming lipid- DNA complexes or lipoplexes. Cellular uptake: Lipoplexes are taken up by cells through endocytosis or direct fusion with the cell membrane. Endosomal escape: Lipoplexes may need to escape from endosomes to avoid degradation in lysosomes, facilitated by pH-sensitive lipids or endosome-disrupting peptides. Nuclear localization: Lipoplexes must reach the nucleus for gene expression, aided by nuclear localization signals. Gene expression: Once inside the nucleus, the DNA undergoes transcription and translation to produce the desired protein. Non-Viral Methods of Gene Delivery Applications Transient gene expression: Commonly used for transient gene expression experiments. Gene silencing: Delivery of siRNA or shRNA for gene knockdown studies or therapeutic applications. Gene editing: Delivery of gene-editing tools, such as CRISPR/Cas9 components, for targeted genome modifications. Electroporation: Principles and Applications Principles Preparation of cells: Cells are suspended in a conductive buffer and mixed with DNA. Electroporation pulse application: Cells are subjected to a brief, high-voltage electric pulse, creating temporary pores in the cell membrane. DNA uptake: The temporary pores allow DNA to enter the cells. Cell recovery: After electroporation, cells recover and express the introduced DNA. Applications Electroporation is utilized for various applications, including the delivery of DNA, RNA, proteins, and small molecules into a wide range of cell types for gene expression, gene editing, and drug development studies. Applications of Electroporation Transient gene expression Often used for transient gene expression studies, allowing introduced genes to be expressed for a limited period. Gene editing Delivery of gene-editing tools, such as CRISPR/Cas9 components, for efficient genome modifications. Cell transformation Commonly employed in bacterial and yeast transformation to introduce foreign DNA into these cells. Cell-based therapies Used in ex vivo gene therapy approaches, where cells are removed from the body, transfected with the gene of interest, and then re-introduced into the patient. Comparison of Viral and Non-Viral Vectors Advantages of Viral Vectors Efficient gene delivery into host cells. Long-term gene expression through integration into the host genome. Suitable for in vivo gene therapy applications. Disadvantages of Viral Vectors Immunogenicity and potential safety concerns. Limited cargo capacity and manufacturing challenges. Advantages of Non-Viral Vectors Generally safer with lower immunogenicity. Flexible in cargo capacity and relatively easier to manufacture at large scales. Disadvantages of Non-Viral Vectors Less efficient gene delivery compared to viral vectors. Typically limited to transient gene expression. Viral Vectors: Advantages High Transduction Efficiency Viral vectors efficiently infect and deliver genes into host cells, resulting in high transduction efficiency. Stable and Long-Term Gene Expression Integrating viral vectors, such as lentiviral vectors, can integrate their genetic material into the host genome, leading to stable and long-term gene expression. Cell Type Specificity Some viral vectors can be engineered to target specific cell types by modifying their surface proteins or using cell-specific promoters. Large Cargo Capacity Viral vectors can accommodate relatively large DNA sequences, allowing the delivery of full-length genes or gene clusters. Viral Vectors: Disadvantages Immunogenicity Viral vectors can trigger immune responses in the host, potentially leading to inflammation or immune rejection. Safety Concerns The use of viral vectors carries the risk of insertional mutagenesis, potentially leading to adverse effects by disrupting normal gene function. Limited Cargo Capacity Although relatively large compared to non-viral vectors, viral vectors are still limited in the size of DNA they can carry. Manufacturing Challenges Viral vectors can be more challenging and costly to produce at large scales compared to non-viral vectors. Non-Viral Vectors: Advantages Safety Generally considered safer than viral vectors, as they do not carry the risk of viral- induced immune responses or insertional mutagenesis. Versatility Can deliver a wide range of nucleic acids, including plasmid DNA, mRNA, siRNA, and other small molecules. Ease of Manufacturing Can be synthesized and produced relatively easily and cost-effectively compared to viral vectors. Large Cargo Capacity Some non-viral vectors, such as artificial chromosomes, have the potential to carry large DNA fragments. Non-Viral Vectors: Disadvantages Lower Transduction Efficiency Generally, have lower transduction efficiency compared to viral vectors, which limits their applications in certain contexts. Transient Gene Expression Often result in transient gene expression, meaning that the introduced genes are only expressed for a limited period. Delivery Challenges Face obstacles in efficiently delivering genes to target cells due to issues such as endosomal entrapment or degradation. Limited Cell Type Specificity Typically lack the cell-targeting capabilities inherent in viral vectors, making specific cell targeting more challenging. Factors for Choosing the Appropriate Vector Type When choosing the appropriate vector type for gene delivery, several factors should be considered: Transduction Efficiency If high transduction efficiency is crucial, viral vectors may be preferred due to their natural ability to efficiently infect cells. Duration of Gene Expression For long-term or stable gene expression, integrating viral vectors, such as lentiviral vectors, can be suitable. For transient gene expression, non-viral vectors are often used. Safety Considerations If safety is a major concern, non-viral vectors are generally considered safer since they do not carry the risk of viral-induced immune responses or insertional mutagenesis. Factors for Choosing the Appropriate Vector Type Cargo Capacity If large DNA fragments or gene clusters need to be delivered, viral vectors with their larger cargo capacity might be more appropriate. Target Cell Type Some viral vectors can be engineered to specifically target certain cell types, making them advantageous for cell-specific gene delivery. Non-viral vectors may be more versatile but lack inherent cell targeting capabilities. Manufacturing Scalability and Cost Non-viral vectors are generally easier and more cost-effective to produce at large scales compared to viral vectors, which may influence the choice depending on the application. Regulatory Considerations The choice of vector type may also be influenced by regulatory guidelines and approvals specific to gene therapy or other applications. Regulatory Elements in Vectors Enhancers: Enhancing Gene Expression Role: Enhancers are regulatory elements found in DNA sequences that play a crucial role in enhancing gene expression. Function: They increase the transcriptional activity of the genes of interest by interacting with transcription factors and other proteins to promote gene expression. Location: Typically located upstream or downstream of the gene's promoter region. Main Functions of Enhancers Increasing Transcriptional Activity Facilitate the recruitment of transcription factors and RNA polymerase II to the promoter region, leading to increased transcriptional activity of the gene. Cell-Specific Gene Expression Confer cell-type specificity to gene expression, allowing the targeting of gene expression to desired cell populations. Enhancing Expression Levels Increase the transcriptional activity of the gene, leading to higher levels of gene expression, which may be important for achieving therapeutic effects in gene therapy applications. Enhancing Temporal Expression Some enhancers are regulated by specific signals or conditions, allowing for temporal control of gene expression, which can be useful in applications requiring precise timing of gene expression. Terminators: Ensuring Proper Transcriptional Termination Role: Terminators, also known as polyadenylation signals or poly(A) signals, are regulatory elements that ensure proper transcriptional termination of genes. Location: Typically located downstream of the coding sequence in DNA. Function: Essential for the processing and stability of mRNA molecules, playing a crucial role in gene delivery vectors to ensure accurate gene expression and prevent read- through transcription. Main Functions of Terminators mRNA Processing Contain specific sequences that signal the end of the gene's coding region, recognized by molecular machinery involved in mRNA processing, including cleavage and polyadenylation factors. Proper mRNA processing is essential for the stability and functionality of the mRNA molecule. Transcriptional Termination Facilitate the termination of transcription by helping to release the RNA polymerase complex from the DNA template, ensuring that transcription stops at the appropriate site and prevents read-through transcription into neighbouring genes. mRNA Stability Contribute to mRNA stability by proper termination and polyadenylation, protecting it from degradation. The addition of a poly(A) tail at the 3' end of mRNA provides stability and aids in efficient translation of the mRNA into protein. Factors Influencing Vector Choice Cell Type: Considerations for Different Mammalian Cell Lines When choosing a vector for gene delivery, the specific cell type in which the gene will be expressed is an important consideration. Different mammalian cell lines have varying characteristics and requirements for successful gene delivery. Here are some factors to consider when selecting a vector based on the target cell type: Receptor Expression Some cell types may express specific receptors on their surface that can be targeted by viral vectors. For example, adenoviral vectors can efficiently infect a wide range of cell types due to the ubiquity of the coxsackievirus and adenovirus receptor (CAR) on many cell surfaces. Entry Mechanisms Different cell types may have distinct mechanisms for internalizing vectors, influencing the choice of vector type and modifications required for efficient delivery. Immune Response The immune response of the target cell type should be considered, as some vectors can induce immune reactions that may impact gene expression. Viral vectors, in particular, may trigger immune responses, leading to inflammation or compromised transduction efficiency in specific cell types. Factors Influencing Vector Choice Transduction Efficiency The transduction efficiency of different vectors can vary depending on the target cell type, impacting gene expression levels in the desired cell population. Cell-Specific Promoters Incorporating cell-specific promoters in vectors allows for targeted and specific gene expression, potentially minimizing off-target effects. Culture Requirements Compatibility with the vector system should be considered to ensure successful gene delivery without compromising cell viability or function, taking into account specific culture requirements of different cell lines. Gene Size and Expression Level: Matching with Vector Capacity The size of the gene of interest and its expected expression level can influence the choice of vector, as different vectors have varying capacity and efficiency for delivering large or small genes and achieving high or low expression levels. Consider the following factors: Cargo Capacity Viral vectors generally have limited cargo capacity, and the size of the gene may exceed the maximum capacity of certain vectors. Non-viral vectors, such as plasmids, can accommodate larger DNA sequences. Alternative strategies like splitting the gene into multiple fragments or utilizing viral genome engineering techniques may be considered if the gene exceeds the capacity of available vectors. Gene Size and Expression Level: Matching with Vector Capacity Promoter Strength The choice of promoter can influence the expression level of the gene. Strong promoters can drive high levels of gene expression, which may be desirable for certain applications. Viral vectors often have strong promoters within their genome, while non-viral vectors allow for more flexibility in promoter selection. Regulatory Elements The inclusion of enhancers and other regulatory elements in the vector can influence gene expression levels. Enhancers can enhance gene expression, while other elements like insulators can modulate or suppress expression. Matching the regulatory elements with the desired expression level is crucial for achieving the desired outcome. Other Considerations: Safety, Stability, and Long- Term Expression In addition to cell type and gene characteristics, several other factors should be considered when choosing a vector for gene delivery: Safety Viral vectors carry the risk of immunogenicity and potential insertional mutagenesis. Non-viral vectors are generally considered safer but may have lower transduction efficiency. The balance between efficiency and safety should be carefully evaluated based on the application. Stability The stability of the vector in the target cell type is important for long-term gene expression. Some viral vectors, like lentiviral vectors, integrate their genetic material into the host genome, providing stable and long-term expression. Non-viral vectors generally result in transient gene expression. Other Considerations: Safety, Stability, and Long- Term Expression Long-Term Expression Vectors that can establish persistent expression, such as integrating viral vectors or episomal vectors, may be preferred for long-term gene expression. Transient expression may be sufficient for some applications. Manufacturing Considerations The scalability and cost of vector production should be taken into account. Viral vectors can be more challenging and costly to produce at large scales compared to non-viral vectors, which may influence the choice depending on the intended application and production requirements. Regulatory Approvals Compliance with regulatory requirements is essential when considering the clinical translation of gene delivery systems, and the choice of vector may be influenced by regulatory guidelines and approvals specific to gene therapy or other applications. Importance of Selection Markers in Identifying Transformed Cells Selection markers play a crucial role in identifying and isolating cells that have successfully taken up and expressed the gene of interest delivered by a vector. These markers provide a selectable phenotype to transformed cells, allowing for their isolation and enrichment. The use of selection markers is particularly important in cases where the efficiency of gene delivery is low, and only a small fraction of cells acquire and express the gene. Reasons for Incorporating Selection Markers in Vectors Enrichment of Transformed Cells Enables the identification and enrichment of cells that have taken up and expressed the vector, crucial when the efficiency of gene delivery is low. Discrimination from Untransformed Cells Provides a clear distinction between transformed cells and untransformed cells in a mixed population, especially when the gene of interest does not confer a readily distinguishable phenotype. Confirmation of Successful Gene Transfer Serves as an indicator of successful gene transfer and expression, confirming that the gene of interest has been delivered and is functional. Types of Selection Markers and Their Mechanisms Selection markers used in vectors can be classified into different types based on their mechanisms of action. Here are some commonly used selection markers: Antibiotic Resistance Genes Provide resistance to specific antibiotics, allowing transformed cells to survive in the presence of the antibiotic while untransformed cells die. Examples include neomycin resistance gene (neo), puromycin resistance gene (pac), and hygromycin resistance gene (hyg). Fluorescent Proteins Such as green fluorescent protein (GFP) or red fluorescent protein (RFP), are detectable by fluorescence microscopy or flow cytometry, enabling the identification and isolation of transformed cells based on their fluorescence. Types of Selection Markers and Their Mechanisms Genetic Complementation Markers Rely on the restoration of a specific cellular function in transformed cells. For example, the herpes simplex virus thymidine kinase (HSV-TK) gene can be used as a complementation marker. Auxotrophic Markers Exploit the inability of transformed cells to synthesize certain essential molecules, allowing only cells expressing the auxotrophic marker to survive and grow. Reporter Genes Indicate gene expression levels or specific cellular events. For example, the β- galactosidase (lacZ) gene can be used as a reporter marker, allowing the detection of transformed cells through the enzymatic activity of β-galactosidase. Other reporter genes include luciferase and alkaline phosphatase. Designing and Constructing Vectors for Gene Expression When designing and constructing vectors for gene expression, it is important to have a good understanding of the different components and characteristics of vectors. This knowledge allows researchers to make informed decisions and optimize vector design for their specific gene expression goals. Here are some key considerations: Vector Backbone Serves as the structural framework for the gene of interest and other components, including origins of replication, selectable markers, and cloning sites. The choice depends on factors such as the desired host organism, stability, copy number, and compatibility with downstream applications. Promoters DNA sequences that drive gene expression, determining timing, strength, and cell type specificity. Consider activity level, tissue specificity, and response to specific conditions when selecting a promoter. Designing and Constructing Vectors for Gene Expression Enhancers and Regulatory Elements Enhancers can increase promoter activity, while regulatory elements like insulators can modulate or suppress gene expression, allowing precise control. Polyadenylation Signal Crucial for mRNA stability and proper termination of transcription, ensuring the production of stable and functional mRNA transcripts. Selection Markers Important for identifying and enriching transformed cells based on the mechanism of action and target cell type. Sequencing and Cloning Sites Essential for vector construction and manipulation, allowing for insertion, verification of the gene of interest, and other modifications. Considerations for Vector Design in Specific Applications Gene Therapy Requires careful consideration of safety, stability, and long-term expression. Integration-deficient viral vectors or non-viral vectors and cell-specific and regulated expression promoters may be desirable. Protein Production Requires strong and inducible promoters, efficient translation initiation signals, appropriate secretion signals, optimization of codon usage, and tags or fusion partners for purification or detection. Functional Genomics Requires specific elements for gene silencing or genome editing, such as shRNA or gRNA sequences, and the necessary machinery for RNA interference or CRISPR-Cas9- mediated gene editing. Considerations for Vector Design in Specific Applications Cell Lineage Tracing Utilizes reporter genes and requires regulatory elements for lineage-specific expression to visualize and track specific cell populations. Inducible Gene Expression Involves incorporating inducible promoters, such as tetracycline-inducible systems, for controlled gene expression. Tissue-Specific Expression Requires tissue-specific promoters or enhancers to achieve the desired expression pattern and minimize off-target effects. Summary Importance of Selection Markers: Selection markers are essential for identifying and isolating transformed cells that have successfully taken up and expressed the gene of interest. They allow for the enrichment of transformed cells, discrimination from untransformed cells, and confirmation of successful gene transfer. Types of Selection Markers and Their Mechanisms: Antibiotic resistance genes provide resistance to specific antibiotics, allowing transformed cells to survive in the presence of the antibiotic. Fluorescent proteins enable the identification and isolation of transformed cells based on their fluorescence. Genetic complementation markers restore specific cellular functions in transformed cells. Auxotrophic markers exploit the inability of transformed cells to synthesize certain essential molecules. Reporter genes indicate gene expression levels or specific cellular events. Summary Vector Design Considerations: Vector Backbone: Choose an appropriate vector backbone based on the host organism, stability, copy number, and compatibility with downstream applications. Promoters: Select promoters with desired activity levels, tissue specificity, and response to specific conditions. Enhancers and Regulatory Elements: Incorporate enhancers to increase promoter activity and regulatory elements to modulate gene expression. Polyadenylation Signal: Include an appropriate polyadenylation signal for mRNA stability and proper transcription termination. Selection Markers: Choose selection markers based on the target cell type and downstream applications. Sequencing and Cloning Sites: Incorporate suitable sites for vector construction and manipulation.