Quality Control in Aeronautical Engineering PDF
Document Details
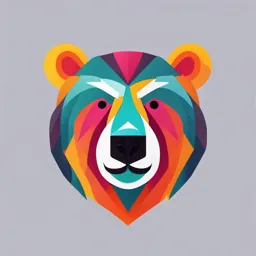
Uploaded by PoisedGladiolus3131
Tags
Related
- Philippine Aeronautical Engineering Decree PDF
- Fundamentals of Aerospace Engineering PDF 2014
- Weight & Balance (Aeronautical Engineering) PDF
- Engineering Materials II (ME2301) Notes - SEM2 (2) PDF
- Philippine Aeronautical Engineering Decree PDF
- Philippine Aeronautical Engineering Rules & Regulations (PDF)
Summary
This document provides an introduction to quality control in aeronautical engineering. It covers key processes such as design review, manufacturing control, functional testing, and inspections, along with nondestructive evaluations. The document also discusses statistical process control and continuous improvement strategies.
Full Transcript
Quality Control in Aeronautical Engineering Quality control plays a crucial role in the aeronautical industry, ensuring the safety and reliability of aircraft. Every component, from the smallest rivet to the most complex system, must undergo rigorous quality checks to meet stringent standards. Impo...
Quality Control in Aeronautical Engineering Quality control plays a crucial role in the aeronautical industry, ensuring the safety and reliability of aircraft. Every component, from the smallest rivet to the most complex system, must undergo rigorous quality checks to meet stringent standards. Importance of Quality Control 1 Safety 2 Reliability Quality control is paramount in aeronautical engineering, ensuring Ensuring the aircraft operates reliably and efficiently, reducing the safety of passengers, crew, and ground personnel. maintenance costs and delays. 3 Performance 4 Compliance Maintaining optimal performance and fuel efficiency, which Adherence to regulatory standards and certifications, essential for directly impacts operating costs and environmental impact. obtaining flight clearance and operating within legal frameworks. Key Quality Control Processes Design Review 1 Thorough evaluation of the aircraft design, ensuring compliance with safety standards and aerodynamic principles. 2 Material Inspection Verifying the quality and integrity of materials used in aircraft construction, ensuring they meet specified properties and Manufacturing Control 3 standards. Monitoring and verifying the manufacturing process to ensure consistent and accurate production of aircraft components. 4 Assembly Inspection Rigorous inspection of aircraft assembly, ensuring proper fitment, alignment, and functionality of all components. Functional Testing 5 Testing various aircraft systems and functionalities to ensure their proper operation under different conditions. 6 Pre-Flight Inspection A comprehensive inspection of the aircraft before each flight, ensuring the safety of the aircraft and passengers. Inspection and Testing Procedures Visual Inspection Dimensional Inspection Functional Testing A thorough visual examination of Measuring critical dimensions of Testing the functionality of various components for defects, such as components to ensure they meet aircraft systems, such as the engine, cracks, dents, or corrosion, using specified tolerances using precision hydraulics, and avionics, to ensure their various tools and techniques. measuring tools and equipment. proper operation. Nondestructive Evaluation Techniques Ultrasonic Testing Using sound waves to detect internal flaws and defects, commonly used for inspecting metal components. Eddy Current Testing Detecting surface defects and changes in metal properties, used for inspecting components made of conductive materials. Radiographic Testing Using X-rays or gamma rays to create images of internal structures, revealing hidden cracks, voids, or inclusions. Magnetic Particle Testing Detecting surface cracks in ferromagnetic materials, using magnetic fields and iron particles to reveal defects. Statistical Process Control Statistical Process Control (SPC) Monitoring and controlling manufacturing processes to ensure consistency and reduce variations. Control Charts Visual representations of process data, used to track trends, identify potential issues, and take corrective actions. Data Analysis Analyzing process data to identify root causes of variations, improve efficiency, and enhance product quality. Continuous Improvement Strategies Root Cause Analysis Process Optimization Investigating the underlying causes of Continuously improving processes and quality issues, identifying and procedures to enhance efficiency, addressing the root problems to reduce waste, and improve product prevent recurrence. quality. Employee Training Data Collection and Analysis Providing employees with training and development opportunities to enhance Systematically collecting and analyzing their skills and knowledge in quality data to identify areas for improvement, control practices. monitor trends, and assess the effectiveness of quality initiatives. Regulatory Compliance and Certification Safety Regulations Airworthiness Certification Adherence to rigorous safety regulations and standards, ensuring the aircraft meets specific Obtaining certification from regulatory safety criteria. bodies, ensuring the aircraft meets required standards for safe flight. Periodic Inspections Documentation and Records Regular inspections and maintenance to ensure ongoing compliance with regulations Maintaining detailed documentation of and maintain the aircraft's airworthiness. quality control activities, including inspection records, test results, and corrective actions. Design of Experiments in Aeronautical Engineering Design of Experiments (DOE) is a powerful methodology used to optimize and improve aircraft design and performance. This presentation explores the principles, strategies, and applications of DOE in aeronautical engineering. by Sara El-Bahloul preencoded.png Introduction to Design of Experiments (DOE) 1 Systematic Approach 2 Variable Manipulation DOE is a structured It involves deliberately methodology for changing input variables planning, conducting, to observe their effects analyzing, and on output responses. interpreting experiments. 3 Data-Driven Insights DOE helps identify optimal combinations of factors to maximize desired outcomes and minimize undesirable ones. preencoded.png Experimental Design Principles and Strategies Randomization Replication Blocking Randomizing the order of Repeating experiments multiple Grouping experiments based on experimental runs helps minimize times enhances the reliability and similar conditions reduces the impact of uncontrolled precision of the results. variability and improves the variables. efficiency of analysis. preencoded.png Factorial Experiments in Aeronautics Multiple Factors Factor Levels Investigate the combined Each factor is set at effects of two or more different levels to observe design variables. their influence on the response. Systematic Combinations All possible combinations of factor levels are tested to understand interactions between variables. preencoded.png Full Factorial Design Example The goal is to study the effect of two factors: 1. Wing angle of attack (AoA) 2. Airfoil shape on the Lift generated by an aircraft wing. The experiment will help determine the best combination of wing angle and airfoil shape to maximize lift. preencoded.png Full Factorial Design Example preencoded.png Full Factorial Design Example preencoded.png Full Factorial Design Example preencoded.png Full Factorial Design Example preencoded.png Full Factorial Design Example preencoded.png Full Factorial Design Example preencoded.png Full Factorial Design Example preencoded.png Full Factorial Design Example preencoded.png Faculty of Engineering New Mansoura University MEC321 DOF & QUALITY CONTROL 1 Accuracy Measurement There are various ways of accuracy measurement such as: 1. Mean (arithmetic average): This is simply the average of the observations, the sum of all the measurements divided by the number of the observations. The ‘Grand’ or ‘Process Mean’ (X̿) is the average of all the sample means, is a good estimate of the population mean. 2 Accuracy Measurement There are various ways of accuracy measurement such as: 2. Median: If the measurements are arranged in order of magnitude, the median is simply the value of the middle item. 5 2 7 9 3 2 3 5 7 9 3 Accuracy Measurement There are various ways of accuracy measurement such as: 3. Mode: When data are grouped into a frequency distribution, the mid-point of the cell with the highest frequency is the modal value. 4 Accuracy Measurement Mean ̶ Mode = 3 (Mean ̶ Median) 5 Precision or Spread Measurement Measures of the extent of variation in process data can be performed by many methods: 1. Range: is the difference between the highest and the lowest observations. The range is usually given the symbol Ri. The mean range R̅ is the average of all the sample ranges. 2. Standard deviation (σ): it takes all the data into account and is a measure of the ‘deviation’ of the values from the mean. 6 Mean & Standard Deviation 7 Example 100 steel rod lengths as 25 samples of size 4 8 Example 100 steel rod lengths as 25 samples of size 4 For sample number 1: Mean Range (R) is the difference between the longest (154 mm) and the shortest (144 mm), so equal to 10 mm. 9 Example 100 steel rod lengths as 25 samples of size 4 For sample number 1: Squared deviation Variance Standard Deviation 10 Example 100 steel rod lengths as 25 samples of size 4 For sample number 1: Estimated Standard Deviation (S): 11 12 DOE Classification 13 DOE Classification DOE Classification DOE Solved Example Response: Fuel Efficiency (Km/L) 16 DOE Solved Example Full Factorial Design 17 DOE Solved Example Fractional Factorial Design 18 DOE Solved Example TAGUCHI Method 19 DOE Solved Example TAGUCHI Method 20 DOE Solved Example TAGUCHI Method 21 DOE Solved Example Response Surface Methodology 22 23 Faculty of Engineering New Mansoura University MEC321 DOF & QUALITY CONTROL 1 Control Chart 2 Control Chart for Variables Mean Chart Range Chart (X̅-Chart) (R-Chart) Estimated Standard Deviation Chart (S-Chart) 3 Example How to construct the 100 steel rod lengths as 25 samples of size 4 Mean and Range control charts from these data? 4 Example Range: is the difference between the highest and the lowest observations. 5 Example Mean Chart Control Limits Calculations for Mean Chart Upper Control Limit UCL & Lower Control Limit LCL: The value of A can be derived directly from Appendix A 6 Example Mean Chart 7 Example Mean Chart 8 Example Mean Chart 155 Sample Mean 150 5 10 15 20 25 145 Sample Number 9 Example Mean Chart UCL 155 UWL Sample Mean 150 5 10 15 20 25 145 LWL LCL Sample Number 10 Example Mean Chart Example Mean Chart UCL 155 UWL Sample Mean 150 5 10 15 20 25 145 LWL LCL Sample Number 12 Example Mean Chart UCL 155 UWL Sample Mean 150 5 10 15 20 25 145 LWL LCL Sample Number 13 Example Mean Chart UCL 155 UWL Sample Mean 150 5 10 15 20 25 145 LWL LCL Sample Number 14 Example Mean Chart Out of control UCL 155 UWL Sample Mean 150 5 10 15 20 25 145 LWL LCL LCL Sample Number 15 Example 16 Control Chart Zones 17 18 19 20 Selecting the correct nozzle diameter is essential for achieving the balance between fuel efficiency, performance, emission control, and durability of a jet engine. 21 22 Faculty of Engineering New Mansoura University MEC321 DOF & QUALITY CONTROL 1 Control Chart for Variables Mean Chart Range Chart (X̅-Chart) (R-Chart) Estimated Standard Deviation Chart (S-Chart) 2 Example Range Chart 20 Sample Range 10 5 10 15 20 25 0 Sample Number 3 Example Range Chart 4 Example Range Chart 20 Sample Range 10 5 10 15 20 25 0 Sample Number 5 Example Range Chart 20 Sample Range 10 5 10 15 20 25 0 Sample Number 6 Example Range Chart Control Limits Calculations for Range Chart Upper Control Limit UCL & Lower Control Limit LCL: Appendix B provides the constants D’0.001 and D’0.999 7 Example Range Chart 8 Example Range Chart UCL = 9 Example 10 R Control Chart 11 Example 12 X̅ and R Control Chart Steps 13 Guidelines for selecting ‘n’ ✓ The sample size should be at least 2 to give an estimate of residual variability, but a minimum of 4 is preferred. ✓ As the sample size increases, the mean control chart limits become closer to the process mean. This makes the control chart more sensitive to the detection of small variations in the process average. ✓ As the sample size increases, the inspection costs per sample may increase. ✓ The sample size should not exceed 12 if the range is to be used to measure process variability. With larger samples the resulting mean range (R̅) does not give a good estimate of the standard deviation and sample standard deviation charts should be used. ✓ When destructive testing is being used, a small sample size is desirable and satisfactory for control purposes. ✓ The technology of the process may indicate a suitable sample size. ✓ A sample size of n = 5 is often used because of the ease of calculation of the sample mean. However, with the advent of inexpensive computers and calculators, this is no longer necessary. 14 15 Faculty of Engineering New Mansoura University MEC321 DOF & QUALITY CONTROL 1 Meeting the Requirements In managing variables the usual aim is not to achieve exactly the same length for every steel rod, but to reduce the variation of products and process parameters around a target value. This means that, in controlling a process, it is necessary to establish first that it is in statistical control, and then to compare its centering and spread with the specified target value and specification tolerance. 2 Meeting the Requirements In order to manufacture within the specification, the distance between the upper specification limit (USL) or upper tolerance (T) and lower specification limit (LSL) or lower tolerance (T), i.e. (USL–LSL) or 2T must be equal to or greater than the width of the base of the process bell, i.e. 6σ. 3 Meeting the Requirements High Relative Precision, where the tolerance band is very much greater than 6σ (2T >> 6σ) Medium Relative Precision, where the tolerance band is just greater than 6σ (2T > 6σ) Low Relative Precision, where the tolerance band is less than 6σ (2T < 6σ) 4 Process Capability Indices Cp Process Variation Cpk Centering 5 Process Capability Indices Clearly, if the value of Cp is below 1 that means the process variation is greater than the specified tolerance band so the process is incapable. For increasing values of Cp the process becomes increasingly capable. 6 Process Capability Indices Cp can’t take account of centering. So there is a need for another index which takes account of both the process variation and the centering. 7 Process Capability Indices Another index which takes account of centering is Cpk. 8 Process Capability Indices The overall process Cpk is the lower value of Cpku and Cpkl. A Cpk of 1 or less means that the process variation and its centering is such that at least one of the tolerance limits will be exceeded and the process is incapable. As in the case of Cp, increasing values of Cpk correspond to increasing capability. The Cpk can be used when there is only one specification limit, upper or lower. 9 Process Capability Indices Incapable Critical Capability Low Capability Medium Capability High Capability 10 Example In tablet manufacture, the process parameters from 20 samples of size n = 4 are: 11 Example 12 Example Conclusion: The process is centered (Cp = Cpk) and of low capability since the indices are only just greater than 1. 13 Example In tablet manufacture, the process parameters from 20 samples of size n = 4 are: 14 Example Conclusion: The Cp at 1.89 indicates a potential for higher capability, but the low Cpk shows that this potential is not being realized because the process is not centered. 15 16