Biosafety, Intellectual Property Rights (2016/2017) PDF
Document Details
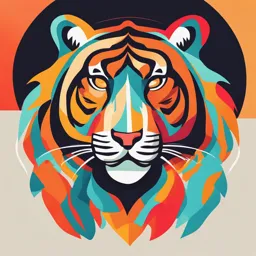
Uploaded by DeliciousFreeVerse
2017
Tags
Summary
These class notes cover biosafety and intellectual property rights, specifically focusing on issues within food and agricultural systems in Africa. The document also delves into transgenic crops and associated risks and regulatory systems.
Full Transcript
TITLE: SBC 844 – BIOSAFETY AND INTELLECTUAL PROPERTY RIGHTS 2016/2017 – TRI-SEM CLASS: MSC (BIOTECHNOLOGY) – TRI-SEM LECTURER: DR. T. O. MAGOMERE Topic...
TITLE: SBC 844 – BIOSAFETY AND INTELLECTUAL PROPERTY RIGHTS 2016/2017 – TRI-SEM CLASS: MSC (BIOTECHNOLOGY) – TRI-SEM LECTURER: DR. T. O. MAGOMERE Topic Assessment 1 Introduction 2 Biosafety issues in food and agricultural systems in Africa 3 Laboratory Level Biosafety 4 Transgenic crops in Africa: Current status and future prospects 5 Environmental biosafety issues associated with GE crops in Africa 6 Food safety issues associated with foods derived from GE crops in CAT 1 (10 marks) Africa 7 Legislative and policy issues associated with GE crops in Africa 8 Socio-economic issues associated with GE crops in Africa 9 Biosafety regulatory systems in Africa 10 Intellectual property rights 11 Industrial property 12 Plant breeders rights 13 Trips agreements 14 Transfer of intellectual property rights CAT 2 (10 marks) 15 Intellectual property Term paper “Case study + presentation” (5 marks) Term paper “Risk assesment” ; “ Environmental biosafety”, (5 marks) “Seralini” Exam (70 marks) Reading materials: 1. James, C. 2013. Global Status of Commercialized Biotech/GM Crops: 2014. ISAAA Brief No. 46. ISAAA: Ithaca, New York. 2. David P. Keetch, Diran Makinde, Cholani K. Weebadde and Karim M. Maredia. 2014. Biosafety in Africa: Experiences and best practices.Michigan State University; East Lansing. 3. Craig, W., M. Tepfer , G. Degrassi, and D. Ripandelli. 2008. An overview of general features of risk assessments of genetically modified crops. Euphytica 164: 853-880. 4. Gatehouse, A.M.R., N. Ferry, M.G. Edwards, and H.A. Bell. 2011. Insect-resistant biotech crops and their impacts on beneficial arthropods. Philos Trans R Soc Lond B Biol Sci 366: 1438–1452. 5. Ellstrand, N.C. 2003. Current Knowledge of gene flow in plants: implications for transgene flow. Philosophical Transactions B 358:1163-1170. 6. Kshitij Kumar Singh. 2015. Biotechnology and Intellectual Property Rights: Legal and Social Implications. Springer New Delhi Heidelberg New York Dordrecht London. DOI 10.1007/978- 81-322-2059-6. 7. Chosewood LC, Wilson DE. Biosafety in microbiological and biomedical laboratories, 5th Ed., 2009. http://www.cdc.gov/biosafety/publications/bmbl5/bmbl.pdf. Chapter 1. Biosafety issues in food and agricultural systems in Africa DENNIS NDOLO OBONYO, MONICA RACOVITA, DECIO RIPANDELLI AND WENDY CRAIG INTRODUCTION The term biosafety has been defined as ‘the avoidance of risk to human health and safety, and to the conservation of the environment, as a result of the use for research and commerce of infectious or genetically modified organisms’ (FAO 2001). Genetically engineered organisms (GEOs/GMOs) are created by transferring genetic material from one organism to another through a process called genetic engineering (GE)4. The protein encoded by the introduced gene will confer a particular trait or characteristic to the recipient organism. Across the millennia, selective breeding and other such techniques have been used by humans to transfer genetic material within species complexes. New technologies such as GE permit more controlled gene transfers, and also allow for transfers among completely unrelated species (Philips 2008). The transferred genes or gene sequences are referred to as transgenes, and biotech plants are therefore also known as transgenic plants (the terms GE and transgenic are therefore used interchangeably in this chapter). GE has been used in agriculture to develop crops with increased crop yields; reduced needs for pesticides; enhanced nutrient composition and food quality; enhanced resistance to pests and diseases, and reduced costs for food or drug production, etc. (Takeda & Matsuoka 2008). While the commercial production of biotech crops with various agronomic beneficial traits has opened a new dimension for meeting food security challenges, it has also aroused tremendous debate and concern worldwide (Pretty 2001). Over the last few years, there has been an increase in research and development in Africa aimed at developing transgenic crops to address constraints to agricultural productivity on the continent. These include projects aimed at developing, amongst many others: Insect-resistant (IR) maize in Kenya; IR cotton in Ghana, Kenya, Nigeria, South Africa and Uganda; Virus-resistant (VR) cassava in Kenya and Uganda; Fungus-resistant banana in Uganda; VR banana in Egypt; Drought-tolerant maize in Kenya, Mozambique, South Africa, Tanzania and Uganda, and; Nutritionally-enhanced sorghum in Burkina Faso, Kenya and South Africa (Karembu et al. 2009). There are also countries that have approved the commercial release of biotech crops, for example IR maize in South Africa and Egypt, and IR cotton in Burkina Faso and South Africa. However, despite the fact that a growing body of evidence continues to document increased crop yields, increased farm income, health and environmental benefits associated with the cultivation of biotech crops (Adenle 2011), there has still been significant debate regarding possible risks to human and animal health, and to the environment, along with associated issues that could arise out of the adoption of such crops. An understanding of biosafety and its related issues is therefore important in helping to make correct decisions when facing and dealing with GE and biotechnology and their products (Lu 2008). This introductory chapter outlines some of the broad biosafety issues pertaining to these technologies that may be pertinent to food and agricultural systems in Africa. 4 In this book the term GE (genetic engineering) is used instead of GM (genetic modification), GEO (genetically engineered organism) instead of GMO (genetically modified organism) and biotech crop or plant instead of GM crop or plant. ~6~ BIOSAFETY ISSUES/CONCERNS REGARDING GE CROPS Most concerns about biotech crops can be placed into four broad categories - environmental, food safety, legal/policy, and socio-economic - although there are cross-cutting issues which may span more than one of these categories. Environmental concerns Environmental concerns relate to the potential impacts on the ecosystem from the adoption of GEOs. These could include the development of resistance/tolerance by target organisms; consequences of gene flow; negative impacts on non-target organisms (NTOs), etc. (Thies and Devare 2007). Development of resistance/tolerance by target organisms One of the concerns, with regard to IR transgenic crops, is the likelihood of the development of resistance/tolerance by insect pests to the transgenic compound in the host crops (Thies and Devare 2007), which may result in loss of usefulness of the control strategy. Pests are considered to have developed resistance if they become able to survive on a transgenic insecticidal plant from egg to adult and produce viable offspring (Andow 2008). A number of strategies to delay resistance development have been proposed, including: The use of GE varieties expressing very high toxin levels such that any individuals that may develop a degree of tolerance are still killed by the toxin upon consumption; Stacking different insect-resistance transgenes together in the same GE variety such that individuals that may not be susceptible to one toxin are killed by a companion toxin with a different mode of action; and Strategically planting non-resistant crops or plants nearby as ‘refugia’ to allow any resistant pest individuals that might develop to mate with non-resistant individuals in order to reduce the frequency of resistance genes in the pest population (Bates et al. 2005). There are concerns that in traditional low input agricultural systems in Africa, whereby farmers save seed, resistance could develop relatively quickly. This is because the plants obtained from the saved seeds of IR transgenic crops may have lower toxin concentrations as compared to the parental generations, and hence permit insects with resistance genes to survive while those lacking the genes are eliminated, gradually resulting in an increase in the number of resistant individuals in the population, thereby hastening the development of resistance. In addition, seed-saving could result in heterogeneous mixtures of GE and non- GE plants in subsequent crops (Fitt et al. 2004), thus exposing the target organisms once more to sub-lethal toxin concentrations and hastening the development of resistance. In principle, however, the high crop diversity which characterises traditional low input agricultural systems in Africa (Boon 2004) should provide suitable refugia for insect-resistant biotech crops. In such systems, it is possible to have a number of non- transgenic crop species in close proximity to GE counterparts. Structured refugia (where areas under GE and non-GE crops are clearly pre-determined, as opposed to random patches of GE versus non-GE plants) are however recommended but their use could necessitate a change in agricultural practices. In addition, given the small farm sizes in many parts of Africa, there may be issues regarding the availability of spare land for refugia. Seed mixes (where GE and non-GE seed are pre-mixed prior to planting) have been proposed as one possible way to ensure farmers have sufficient area under refugia, but it is likely that this strategy may actually hasten the rate of resistance development, as discussed above. Consequences of gene flow Gene flow refers to the introgression of genes or genetic materials from one plant population into another. There are concerns that the integration of transgenes from a biotech crop into its non-GE counterpart (crop- ~7~ to-crop) and/or wild or weedy relatives (crop-to-wild relative) could trigger a range of possible environmental consequences (e.g. the creation of new weeds, and changing the fitness-related characteristics and loss of genetic diversity in the wild relatives of crop landraces caused by crop-crop and crop-to-wild relative transgene flow) (Lu 2008). Genes introgressing into wild relative populations from crops may accentuate the characteristics of weediness in the wild relatives, leading to greater persistence and invasiveness of the wild relatives. Persistence refers to the tendency of a population to remain in a particular setting, over time after it is introduced. Invasiveness, on the other hand, refers to the ability of a population to spread beyond its introduction site and become established in new locations, where it may out-compete existing populations (Lu 2008). There are concerns, therefore, that if wild or weedy species receive genes which increase their fitness in a given environment then they may become more effective and aggressive weeds. With the advent of herbicide tolerant (HT) crops (those transformed by GE such that they are unharmed when sprayed with a broad-spectrum herbicide whilst crop-infesting weeds are destroyed), there is concern that problematic weeds tolerant to multiple herbicides may develop. In addition, those crop plants that emerge in a field in the following growing season as a result of seed spillage (i.e. volunteers) may develop into aggressive weeds after incorporating such transgenes (Ellstrand 2001). Volunteers may be a special problem in the agricultural crop rotations integral to farming systems in Africa (e.g. in South African cultivation, it is common to have maize-cotton-cowpea rotations). The introgression of genes from one species into the gene pool of another unrelated species may alter the fitness of wild plants and consequently the dynamics of wild populations in two ways: 1) Cause local extinction of the wild population (in the case of reducing the fitness of wild plants) or; 2) Make the wild population more invasive and competitive (in the case of increasing the fitness of wild plants) (Snow et al. 2005). The potential of transgenes to increase the invasiveness of a wild species is therefore reliant upon: 1) The presence of sexually-compatible wild relatives; and 2) The resultant impact of the introduced gene. Prediction of the potential environmental consequences of transgene expression in wild relatives under different circumstances can be done through a systematic risk assessment. From the foregoing it is evident that the potential consequences of gene flow should be the focus of risk assessments, rather than gene flow per se. To minimise the possibility of transgene flow, a number of confinement strategies have been developed or proposed, applying physical or biological approaches. Information concerning the location and inter-fertility of compatible relatives, including those of most African crops, is generally available in the scientific literature; whilst information on invasiveness can be obtained from similar sources as well as indigenous knowledge. The most careful evaluation will be needed for those crops that are already invasive or that have invasive sexually-compatible wild relatives (Hancock 2003). Negative impacts on non-target organisms A non-target organism (NTO) is a plant or animal other than the one against which a specific GEO has been developed to have protection against. For example, a crop may be engineered for resistance to a specific insect pest (the target) and any other insects would then be considered non-target organisms. NTOs can be classified into the following categories: Pollinators and natural enemies of the pest, along with the wider category of beneficial species; Soil organisms; Non-target herbivores; ~8~ Endangered and other species of conservation concern, and; Species which contribute to local biodiversity (Craig et al. 2008). The potential impacts of GEOs on NTOs could be: 1) Direct toxicity through ingestion of a toxin produced by the biotech plant, or 2) Indirect via multi-trophic food chains, involving, for example, organisms not directly consuming the biotech plant but predating on prey that consume the transgenic plants (Gatehouse et al. 2011). Possible effects of a biotech crop on NTOs should only be an issue if: 1) The crop has been engineered with a toxin that makes it insect-resistant and there are other organisms present that might be sensitive to the toxin; and 2) The toxin-sensitive organisms can encounter the toxin. NTOs are not expected to be affected by a biotech crop engineered with a trait such as tolerance to herbicide, virus or drought. Food/Feed safety concerns Other than the improvements which are intentionally introduced by the genetic modification, concomitant unintended differences may also occur. The latter have been defined as those differences which go beyond the primary expected effect(s) of introducing the target gene(s) (EFSA 2011). Potential adverse effects of GE food/feed which have raised concerns may include: toxicity of GE food/feed; allergenicity to GE food/feed; changes in nutritional value of GE food/feed; and emergence of resistant strains of bacteria (Key et al. 2008). Toxicity There is a remote possibility that GE could unintentionally introduce a toxic substance, for example a newly- expressed protein, or elevate the expression of an endogenous toxic substance(s) (Key et al. 2008). Conventional non-GE foods already contain a large number of toxic and potentially toxic products, and so the key question is whether a specific GEO could result in a new hazard. The potential toxicity of the protein expressed in a GE food is an essential component of the requisite safety assessment carried out during product development (Malarkey 2003). The safety assessment requires that the amino acid sequence of a novel protein is demonstrated to be sufficiently dissimilar to known protein toxicants, and that the new protein is rapidly digested under simulated mammalian gastric conditions. Animal bioassays may also be conducted on individual proteins to reveal any potential toxicity. Allergenicity Allergic reactions are hypersensitivity responses of the immune system that may occur in sensitive individuals after exposure to certain substances, usually proteins (Bush and Hefle 1996). Concern pertaining to allergenicity relates to: 1) The possibility that genes from known allergens may be inserted into crops not typically associated with allergenicity; and 2) The possibility of creating new, unknown allergens by either inserting novel genes into crops or changing the expression of endogenous proteins (Key et al. 2008). A number of different organisations have produced guidelines and decision trees to experimentally evaluate allergenic potential (Metcalfe 2003). Iteration of nutritional value An unintended effect during the development of a biotech plant may be the lowering of its nutritional quality as compared to its traditional counterpart. This could occur by making nutrients unavailable or ~9~ indigestible to humans through the interference of key metabolic pathways and consequently affecting the production of nutritional components, hence compromising the nutritional quality of the product. Antibiotic resistance marker genes To facilitate the transformation process, a selectable marker gene conferring, for example, resistance to an antibiotic (e.g. kanamycin, which will kill a normal non-GE plant cell during in vitro culture), is often co- transferred with the gene of interest to allow the discrimination of GE tissue and regeneration of GE plants. There have been concerns that this approach presents a route to increasing the spread of antibiotic resistance to bacterial populations either in the soil or in the human gut after ingestion of GE food (Key et al. 2008). This could then render these antibiotics ineffective and/or make some strains of bacteria untreatable in human therapies. The increase of antibiotic resistance in the human population as a result of GE has yet to be demonstrated empirically. In addition, antibiotic resistance genes were originally isolated from bacteria and are already widespread in the bacterial population. Selection strategies that do not rely on antibiotic resistance have however been developed (Goldstein et al. 2005), and procedures to eliminate the selectable marker from the plant genome once its selection purpose has been fulfilled have also been designed (Hare and Chua 2002). These may be recommended for use in commercial GEOs. In light of the food/feed safety issues outlined above, it is necessary that GEOs are assessed to determine the biological relevance and potential to cause harm of any intended or unintended differences. This is especially so given that differences between GE and non-GE crops (whether intended or not) may not necessarily be indicative of an adverse effect. Bodies like the Codex Alimentarius Commission, International Life Science Institute, and the Organization of Economic Cooperation Development have developed guidelines to assist the assessment of the safety of GE foods (Malarkey 2003). Generally, the safety assessment of GE plants and derived food and feed follows a comparative approach, i.e. the food and feed are compared with their non- GE counterparts (comparators). The comparators are crops that already have a so-called “history of safe use”. In the comparative safety assessment, the biotech crop and the comparators are assessed in both phenotypic as well as analytical terms, with the aim to identify differences between the two (types of) crops. Subsequent safety assessment steps then focus on any differences that have been identified, to determine whether these detected differences have any (unintended) toxicological and/or nutritional consequences. In practice, if differences have been identified, the subsequent steps of the food and feed safety assessment procedure are decided on a case-by-case basis, depending on the nature of identified difference(s) (Kok et al. 2010). Legal/policy issues A biosafety regulatory system should ensure the safe use of GEOs, with no significant risks to humans, animals, and the environment, while acknowledging that zero risk technologies are impossible. Assessing the risks of GEOs is regularly done by national regulatory systems strictly following scientific principles, with some authorities also including socio-economic and even political issues in their biosafety regulation. This last approach has been criticised as being outside of the scope of the risk assessment process, and at the same time, opening the GEO authorisation process to interference by special interest groups (Falck-Zepeda and Zambrano 2011). National biosafety regulatory systems are the desired outcome of national biosafety laws and policies and attempts to harmonise them (or otherwise) with international agreements. Directly involved in the international regulation of GEOs are two international organisations: the Convention on Biological Diversity (CBD) and the World Trade Organization (WTO). The first specific international legal framework for biosafety regulation came from the Cartagena Protocol on Biosafety (CPB or “the Protocol”) adopted in 2000 as a supplement for the CBD. The Protocol has specifications concerning the safe use and transport of GEOs (or LMOs,] as the Protocol defines them), with the exception of those GEOs used for pharmaceutical production ~ 10 ~ and those products derived from GEOs that are not intended either for food or feed. The main provisions of the Protocol concern: The establishment of procedures for: advanced informed agreement (AIA) between trading Parties; Risk assessment; Handling, transport, packaging and identification of geos; A biosafety clearing house; Capacity building, especially for Parties from the developing world; Public awareness and participation; socio-economic considerations in decision-making; liability and redress; and Compliance (Gupta et al. 2008). Under the WTO, the agreement on the application of sanitary and phytosanitary (SPS) measures, although not specifically focused on biosafety, concerns food safety as well as animal and plant health, while the technical barriers to trade (TBT) agreement concerns product standards more generally. There are also trade- related aspects of intellectual property rights (TRIPS) agreement setting minimum standards for intellectual property. These WTO agreements allow the member states to set the appropriate level of protection on a case-by-case basis, as long as trade discriminations on similar products are avoided. Similar to the Protocol, the WTO agreements can allow for socio-economic measures, but in very narrowly-defined instances (e.g. damage of production or sales, spreading of pests or diseases). The WTO agreements recommend the use of international standards developed by the Codex Alimentarius Commission, the World Organization for Animal Health (OIE) and the International Plant Protection Convention (Jaffe 2006). Among the 163 signatories of the CPB, approximately 50 are from Africa (Table 1) yet few of these have biosafety laws and regulations in place. As such, the possibility of a conflict between domestic and international regulations is not yet a significant issue in the region. However, capacity building for biosafety regulators remains a key priority for African states (Obonyo et al. 2011). The main needs centre on: an overall lack of local funding, with too many projects depending on external funds; a general lack of awareness of biosafety issues by the public; a dearth in human capacity with experience in the specialist fields supporting GEO decision-making; the application of overly-stringent precautionary regulations in some countries; and the lack of harmonised biosafety regulations at the regional level (Mtui 2012). With respect to the latter, an attempt at harmonising African biosafety regulation occurred in 2007 with the presentation of the African Model Law on Biosafety in Biotechnology, which however takes a more stringent approach to GEO regulation than the Protocol (Gupta et al. 2008). Table 1: African countries status on Cartagena Protocol on Biosafety* Country Signature Ratification/accession Entry into force Angola 27 February 2009 28 May 2009 Benin 24 May 2000 2 March 2005 31 May 2005 Botswana 1 June 2001 11 June 2002 11 September 2003 Burkina Faso 24 May 2000 4 August 2003 2 November 2003 Burundi 2 October 2008 31 December 2008 Cameroon 9 February 2001 20 February 2003 11 September 2003 Cape Verde 1 November 2005 30 January 2006 Central African Republic 24 May 2000 16 February 2009 Chad 24 May 2000 30 January 2007 ~ 11 ~ Comoros 25 March 2009 23 June 2009 Congo 21 November 2000 11 October 2006 Côte d’Ivoire Democratic Republic of the Congo 23 March 2005 21 June 2005 Djibouti 8 April 2002 11 September 2003 Egypt 20 December 2000 23 December 2003 21 March 2004 Equatorial Guinea Eritrea 10 March 2005 8 June 2005 Ethiopia 24 May 2000 9 October 2003 7 January 2004 Gabon 2 May 2007 31 July 2007 Gambia 24 May 2000 9 June 2004 7 September 2004 Ghana 30 May 2003 11 September 2003 Guinea 24 May 2000 10 March 2008 Guinea-Bissau 17 August 2010 Kenya 15 May 2000 24 January 2002 11 September 2003 Lesotho 20 September 2001 11 September 2003 Liberia 15 February 2002 11 September 2003 Libya 14 June 2005 12 September 2005 Madagascar 14 September 2000 24 November 2003 22 February 2004 Malawi 24 May 2000 28 May 2009 Mali 4 April 2001 28 August 2002 11 September 2003 Mauritania 22 July 2005 20 October 2005 Mauritius 11 April 2002 11 September 2003 Morocco 25 May 2000 24 July 2011 Mozambique 24 May 2000 21 October 2002 11 September 2003 Namibia 24 May 2000 10 February 2005 11 May 2005 Niger 24 May 2000 30 September 2004 29 December 2004 Nigeria 24 May 2000 15 July 2003 13 October 2003 Rwanda 24 May 2000 22 July 2004 20 October 2004 São Tomé and Príncipe Senegal 31 October 2000 8 October 2003 6 January 2004 Seychelles 23 January 2001 13 May 2004 11 August 2004 Sierra Leone Somalia 24 October 2010 South Africa 14 August 2003 12 November 2003 Sudan 13 June 2005 11 September 2005 ~ 12 ~ Swaziland 13 January 2006 13 April 2006 Tanzania 24 April 2003 11 September 2003 Togo 24 May 2000 2 July 2004 30 September 2004 Tunisia 19 April 2001 22 January 2003 11 September 2003 Uganda 24 May 2000 30 November 2001 11 September 2003 Zambia 27 April 2004 25 July 2004 Zimbabwe 4 June 2001 25 February 2005 26 May 2005 * http://bch.cbd.int/protocol/parties/ Socio economic issues Due to their centrality in current biosafety-related debates, socio-economic issues, although mentioned in the category above, are treated separately here. The Protocol gives parties discretion to decide whether or not to include socio-economic considerations in their decision-making processes. Currently, discussions revolve around the need to: Agree on a working definition; Develop specific methods for assessment; Determine the timing for such evaluations (ex ante/ex post), and; Establish if socio-economic considerations are to be mandatory or voluntary. The issues included under the umbrella of socio-economics go beyond the strict interpretation of the term, and concern ethical, philosophical, and even religious issues pertaining to GEOs. However, there are a few regulatory systems that included socio-economic considerations in their specifications e.g. South Africa, and the AU Model Law (Falck-Zepeda and Zambrano 2011). Beyond the regulatory conundrums, the socio-economic concerns brought by biotech crops in agriculture centre on the possibility of making farmers more vulnerable to market forces as a result of: changes in cost of agriculture and in agricultural practices; monopoly control of seed supply by trans-national companies; profit margins for farmers being squeezed between seed cost and declining world prices; possible replacement and thus loss of existing robust crop varieties and technologies; challenging market dynamics (Sengooba et al. 2009); and the fear of losing entire portions of foreign markets (Paarlberg 2006). Conclusion The genetic manipulation or modification of plants and animals to obtain improved products has been practiced for thousands of years. However, modern technology allows a greater specificity in employing genes to facilitate crop improvement. In assessing the safety of such products for humans, animal, and the environment, the public debate has evolved beyond technical scientific risk assessments, to encompass issues such as socio-economics. This chapter has outlined some of the key issues that have dominated public discourses on biosafety issues in Africa. However, given that Africa has varied agricultural systems (agro- ecological conditions, farming systems and types of crops) (Detthier and Effenberger 2011; FAO and World Bank 2001) some of the issues highlighted have pertinence in some settings and less relevance in others. It is therefore prudent to consider the issues on a case-by-case basis, rather than to generalise for all contexts. ~ 13 ~ Chapter 2: Laboratory Level Biosafety Hazardous Characteristics of an Agent The principal hazardous characteristics of an agent are: its capability to infect and cause disease in a susceptible human or animal host, its virulence as measured by the severity of disease, and the availability of preventive measures and effective treatments for the disease. The World Health Organization (WHO) has recommended an agent risk group classification for laboratory use that describes four general risk groups based on these principal characteristics and the route of transmission of the natural disease. Classification of Infectious Microorganisms by Risk Group WHO and USA-NIH Risk Group USA-NIH Guidelines for World Health Organization Laboratory Biosafety Classification Research involving Reco- Manual 3rd Edition mbinant DNA Molecules Risk Group 1 Agents not associated with (No or low individual and community risk) A disease in healthy adult microorganism unlikely to cause human or humans. animal disease. Risk Group 2 Agents associated with (Moderate individual risk; low community risk) human disease that is rarely A pathogen that can cause human or animal serious and for which disease but is unlikely to be a serious hazard to preventive or therapeutic laboratory workers, the community, livestock or interventions are often the environment. Laboratory exposures may available. cause serious infection, but effective treatment and preventive measures are available and the risk of spread of infection is limited. Risk Group 3 Agents associated with (High individual risk; low community risk) A serious or lethal human pathogen that usually causes serious human or disease for which preventive animal disease but does not ordinarily spread or therapeutic interventions from one infected individual to another. may be available (high Effective treatment and preventive measures are individual risk but low available. community risk). Risk Group 4 Agents likely to cause (High individual and community risk) A serious or lethal human pathogen that usually causes serious human or disease for which preventive animal disease and can be readily transmitted or therapeutic interventions from one individual to another, directly or are not usually available indirectly. Effective treatment and preventive (high individual risk and measures are not usually available. high community risk). Other hazardous characteristics of an agent include probable routes of transmission of laboratory infection, infective dose, stability in the environment, host range, and its endemic nature. The predominant probable routes of transmission in the laboratory are: 1) Direct skin, eye or mucosal membrane exposure to an agent; 2) Parenteral inoculation by a syringe needle or other contaminated sharp, or by bites from infected animals and arthropod vectors; 3) Ingestion of liquid suspension of an infectious agent, or by contaminated hand to mouth exposure; and 4) Inhalation of infectious aerosols. An awareness of the routes of transmission for the natural human disease is helpful in identifying probable routes of transmission in the laboratory and the potential for any risk to the public health. It is important to remember that the nature and severity of disease caused by a laboratory infection and the probable laboratory route of transmission of the infectious agent may differ from the route of transmission and severity associated with the naturally-acquired disease. Genetically modified agent hazards. The identification and assessment of hazardous characteristics of genetically modified agents involve consideration of the same factors used in risk assessment of the wild-type organism. It is particularly important to address the possibility that the genetic modification could increase an agent’s pathogenicity or affect its susceptibility to antibiotics or other effective treatments. The risk assessment can be difficult or incomplete, because important information may not be available for a newly engineered agent. Several investigators have reported that they observed unanticipated enhanced virulence in recent studies with engineered agents. These observations give reason to remain alert to the possibility that experimental alteration of virulence genes may lead to increased risk. It also suggests that risk assessment is a continuing process that requires updating as research progresses. The NIH Guidelines are the key reference in assessing risk and establishing an appropriate biosafety level for work involving recombinant DNA molecules. The purpose of the NIH Guidelines is to promote the safe conduct of research involving recombinant DNA. The guidelines specify appropriate practices and procedures for research involving constructing and handling both recombinant DNA molecules and organisms and viruses that contain recombinant DNA. They define recombinant DNA as a molecule constructed outside of a living cell with the capability to replicate in a living cell. Basic Principles of Laboratory Biosafety A fundamental objective of any biosafety program is the containment of potentially harmful biological agents. The term “containment” is used in describing safe methods, facilities and equipment for managing infectious materials in the laboratory environment where they are being handled or maintained. The purpose of containment is to reduce or eliminate exposure of laboratory workers, other persons, and the outside environment to potentially hazardous agents. The use of vaccines may provide an increased level of personal protection. The risk assessment of the work to be done with a specific agent will determine the appropriate combination of these elements. Laboratory Practices and Technique The most important element of containment is strict adherence to standard microbiological practices and techniques. Persons working with infectious agents or potentially infected materials must be aware of potential hazards, and must be trained and proficient in the practices and techniques required for handling such material safely. The director or person in charge of the laboratory is responsible for providing or arranging the appropriate training of personnel. Each laboratory should develop or adopt a biosafety or operations manual that identifies the hazards that will or may be encountered, and that specifies practices and procedures designed to minimize or eliminate exposures to these hazards. Personnel should be advised of special hazards and should be required to read and follow the required practices and procedures. A scientist, trained and knowledgeable in appropriate laboratory techniques, safety procedures, and hazards associated with handling infectious agents must be responsible for the conduct of work with any infectious agents or materials. This individual should consult with biosafety or other health and safety professionals with regard to risk assessment. When standard laboratory practices are not sufficient to control the hazards associated with a particular agent or laboratory procedure, additional measures may be needed. The laboratory director is responsible for selecting additional safety practices, which must be in keeping with the hazards associated with the agent or procedure. Appropriate facility design and engineering features, safety equipment, and management practices must supplement laboratory personnel, safety practices, and techniques. Safety Equipment (Primary Barriers and Personal Protective Equipment) Safety equipment includes biological safety cabinet (BSCs), enclosed containers, and other engineering controls designed to remove or minimize exposures to hazardous biological materials. The BSC is the principal device used to provide containment of infectious droplets or aerosols generated by many microbiological procedures. Three types of BSCs (Class I, II, III) used in microbiological laboratories are described and illustrated in Appendix A. Open-fronted Class I and Class II BSCs are primary barriers that offer significant levels of protection to laboratory personnel and to the environment when used with good microbiological techniques. The Class II biological safety cabinet also provides protection from external contamination of the materials (e.g., cell cultures, microbiological stocks) being manipulated inside the cabinet. The gas-tight Class III biological safety cabinet provides the highest attainable level of protection to personnel and the environment. An example of another primary barrier is the safety centrifuge cup, an enclosed container designed to prevent aerosols from being released during centrifugation. To minimize aerosol hazards, containment controls such as BSCs or centrifuge cups must be used when handling infectious agents. Safety equipment also may include items for personal protection, such as gloves, coats, gowns, shoe covers, boots, respirators, face shields, safety glasses, or goggles. Personal protective equipment is often used in combination with BSCs and other devices that contain the agents, animals, or materials being handled. In some situations in which it is impractical to work in BSCs, personal protective equipment may form the primary barrier between personnel and the infectious materials. Examples include certain animal studies, animal necropsy, agent production activities, and activities relating to maintenance, service, or support of the laboratory facility. Facility Design and Construction (Secondary Barriers) The design and construction of the facility contributes to the laboratory workers’ protection, provides a barrier to protect persons outside the laboratory, and protects persons or animals in the community from infectious agents that may be accidentally released from the laboratory. Laboratory directors are responsible for providing facilities commensurate with the laboratory’s function and the recommended biosafety level for the agents being manipulated. The recommended secondary barrier(s) will depend on the risk of transmission of specific agents. For example, the exposure risks for most laboratory work in BSL-1 and BSL-2 facilities will be direct contact with the agents, or inadvertent contact exposures through contaminated work environments. Secondary barriers in these laboratories may include separation of the laboratory work area from public access, availability of a decontamination facility (e.g., autoclave), and hand washing facilities. When the risk of infection by exposure to an infectious aerosol is present, higher levels of primary containment and multiple secondary barriers may become necessary to prevent infectious agents from escaping into the environment. Such design features include specialized ventilation systems to ensure directional airflow, air treatment systems to decontaminate or remove agents from exhaust air, controlled access zones, airlocks at laboratory entrances, or separate buildings or modules to isolate the laboratory. BIOSAFETY LEVELS Four BSLs are described. They consist of combinations of laboratory practices and techniques, safety equipment, and laboratory facilities. Each combination is specifically appropriate for the operations performed, the documented or suspected routes of transmission of the infectious agents, and the laboratory function or activity. Risk groups are the result of a classification of microbiological agents based on their association with, and resulting severity of, disease in humans. The risk group of an agent should be one factor considered in association with mode of transmission, procedural protocols, experience of staff, and other factors in determining the BSL in which the work will be conducted. Generally, work with known agents should be conducted at the optimal biosafety level. When information is available to suggest that virulence, pathogenicity, antibiotic resistance patterns, vaccine and treatment availability, or other factors are significantly altered, more (or less) stringent practices may be specified. Often an increased volume or a high concentration of agent may require additional containment practices. Biosafety Level 1 practices, safety equipment, and facility design and construction are appropriate for undergraduate and secondary educational training and teaching laboratories, and for other laboratories in which work is done with defined and characterized strains of viable microorganisms not known to consistently cause disease in healthy adult humans. Bacillus subtilis, Nigeria gruberi, infectious canine hepatitis virus, and exempt organisms under the NIH Guidelines are representative of microorganisms meeting these criteria. Many agents not ordinarily associated with disease processes in humans are, however, opportunistic pathogens and may cause infection in the young, the aged, and immunodeficient or immunosuppressed individuals. Vaccine strains that have undergone multiple in vivo passages should not be considered avirulent simply because they are vaccine strains. BSL-1 represents a basic level of containment that relies on standard microbiological practices with no special primary or secondary barriers recommended, other than a sink for hand washing. Biosafety Level 2 practices, equipment, and facility design and construction are applicable to clinical, diagnostic, teaching, and other laboratories in which work is done with the broad spectrum of indigenous moderate-risk agents that are present in the community and associated with human disease of varying severity. With good microbiological techniques, these agents can be used safely in activities conducted on the open bench, provided the potential for producing splashes or aerosols is low. Hepatitis B virus, HIV, the Salmonella, and Toxoplasma are representative of microorganisms assigned to this containment level. BSL-2 is appropriate when work is done with any human-derived blood, body fluids, tissues, or primary human cell lines where the presence of an infectious agent may be unknown. Primary hazards to personnel working with these agents relate to accidental percutaneous or mucous membrane exposures, or ingestion of infectious materials. Extreme caution should be taken with contaminated needles or sharp instruments. Even though organisms routinely manipulated at BSL-2 are not known to be transmissible by the aerosol route, procedures with aerosol or high splash potential that may increase the risk of such personnel exposure must be conducted in primary containment equipment, or in devices such as a BSC or safety centrifuge cups. Personal protective equipment should be used as appropriate, such as splash shields, face protection, gowns, and gloves. Secondary barriers, such as hand washing sinks and waste decontamination facilities, must be available to reduce potential environmental contamination. Handling of genetically mordified organisms is best performed at this level of containment. Rare situations involving severely hazardous material may need higher levels of containment. Secondary strategies need to be applied for containing the material (plants, animals, insects, microorganisms etc.) both in the laboratory during genetic transformation, tissue culture, growth and evaluation. Secondary barriers may include growth cabinets, restricted preparation and growth rooms and green/ glass house connected directly to the laboratory. Biosafety Level 3 practices, safety equipment, and facility design and construction are applicable to clinical, diagnostic, teaching, research, or production facilities in which work is done with indigenous or exotic agents with a potential for respiratory transmission, and which may cause serious and potentially lethal infection. Mycobacterium tuberculosis, St. Louis encephalitis virus, and Coxiella burnetii are representative of the microorganisms assigned to this level. Primary hazards to personnel working with these agents relate to autoinoculation, ingestion, and exposure to infectious aerosols. At BSL-3, more emphasis is placed on primary and secondary barriers to protect personnel in contiguous areas, the community, and the environment from exposure to potentially infectious aerosols. For example, all laboratory manipulations should be performed in a BSC or other enclosed equipment, such as a gas-tight aerosol generation chamber. Secondary barriers for this level include controlled access to the laboratory and ventilation requirements that minimize the release of infectious aerosols from the laboratory. Biosafety Level 4 practices, safety equipment, and facility design and construction are applicable for work with dangerous and exotic agents that pose a high individual risk of life- threatening disease, which may be transmitted via the aerosol route and for which there is no available vaccine or therapy. Agents with a close or identical antigenic relationship to BSL-4 agents also should be handled at this level. When sufficient data are obtained, work with these agents may continue at this level or at a lower level. Viruses such as Marburg or Congo-Crimean hemorrhagic fever are manipulated at BSL-4. The primary hazards to personnel working with BSL-4 agents are respiratory exposure to infectious aerosols, mucous membrane or broken skin exposure to infectious droplets, and autoinoculation. All manipulations of potentially infectious diagnostic materials, isolates, and naturally or experimentally infected animals, pose a high risk of exposure and infection to laboratory personnel, the community, and the environment. The laboratory worker’s complete isolation from aerosolized infectious materials is accomplished primarily by working in a Class III BSC or in a full-body, air-supplied positive- pressure personnel suit. The BSL-4 facility itself is generally a separate building or completely isolated zone with complex, specialized ventilation requirements and waste management systems to prevent release of viable agents to the environment. Chapter 23:. Insect Resistance (IR) And Herbicide Tolerance (HT) (a) Herbicide-Tolerant Crops: Roundup Ready Soybean The first transgenic crops grown extensively were designed to address problems of biotic stress, specifically weeds (Round up Ready (RR) Soy), insects (Bt maize), and a virus (papaya). As such, these could be designated as producer-oriented traits, providing the farmer more effective tools for limiting crop losses to these pests. Weeds and Weed Control Herbicides vary in their modes of action, in their effects on weeds and crop plants, and how they are used. Selective herbicides are toxic to some groups of crop species and not others. Problems associated with herbicide use include damage to the crop plants, persistence in the soil and infiltration into the groundwater, toxicity to nontarget organisms, and, not surprisingly, the ability of weeds to develop resistance. The ideal herbicide would be a chemical that is toxic to all weeds, can be sprayed on any crop when and where needed with minimal damage to the crop, is nonpersistent in the soil, does not contaminate the groundwater, and is nontoxic to animals. Despite monumental efforts on the part of many companies and researchers over many years, no such chemical was ever discovered. The arrival of gene-transfer technology in the 1980s, however, provided a genetic strategy for reaching this ideal: find a gene which provides resistance to a nonselective herbicide, transfer the gene to the crop, and spray the crop with herbicide to eliminate weeds only when and where needed. Perhaps the best herbicide candidate for this mission was glyphosate, a chemical developed by Monsanto and marketed as Roundup starting in the 1970s. This herbicide has several attractive –1 qualities. Application rates (0.63 and 0.84 kg ha ) are low compared to other herbicides. It is rapidly immobilized and degraded on the soil surface, and therefore does not move into the groundwater. Glyphosate has very low toxicity to animals such as insects, birds, and mammals. It is translocated by the phloem to rapidly growing portions of the plant, thereby killing not only the aboveground parts of the weed, but also the underground portions of the weed such as roots and rhizomes. This last feature is especially useful as it is one of the few effective chemicals for controlling weeds that spread by underground roots and rhizomes, such as quack grass, milkweed, and thistle. Finally, due to the mode of action of glyphosate, the occurrence of resistant mutants in the weed populations is very low. Glyphosate binds to and affects enzymes involved in the synthesis of the amino acids in plants. Some of these enzymes occur only in plants and microbes, and not in animals, which is why some amino acids are essential to animals and must be supplied in the diet; this also explains why these herbicides have very low toxicity in animals (i.e., the target enzyme does not exist in animals). Glyphosate acts on 5-enolpyruvylshikimate-3-phosphate synthase (EPSPS), a key enzyme in the synthesis of aromatic amino acids such as phenylalanine, tyrosine, and tryptophan. EPSPS catalyzes the reaction of shikimate-3-phosphate with phosphoenolpyruvate (PEP) to form 5- enolpyruvylshikimate-3-phosphate. Glyphosate binds strongly to the enzyme at or very near the binding site for PEP, thereby blocking the normal functioning of the enzyme, which is the basis of its toxicity. This is why the site-modification approach to resistance, that is, finding a resistant form of this enzyme which does not bind the herbicide, is such a challenge, and why this mode of resistance occurs so rarely in weed populations; altering the binding site of the herbicide also alters the binding site for the substrate, reducing amino acid synthesis, and thereby imposing a severe competitive disadvantage on any plant with mutations at this site. Isolation and Transfer of the RR Gene A commercially useful mutant EPSPS gene, termed CP-4, was discovered in a strain of Agrobacterium. The fact that an analog of the gene in plants exists in microbes and the microbial enzyme is similarly inhibited by the herbicide enabled the researchers to harness the power of mutant selection in microbial populations to find this gene. In vitro studies showed that the binding of glyphosate to the EPSPS enzyme encoded by the CP- 4 RR gene was significantly reduced without an excessive cost to the function of the enzyme. The gene-transfer system used to create RR soybean was developed by researchers at Agracetus Inc. in Madison, Wisconsin, in the mid-1980s. The DNA vector containing the RR gene was delivered into cells of soybean embryonic axes, isolated from germinating seeds, by a variation of particle bombardment. Shoots from bombarded axes, regenerated by organogenesis without selection, were screened for beta glucuronidase (GUS) expression in stem cross sections. Particle bombardment was, and still is, a crude tool for transferring genes, being random in sites of insertion and in the scrambling of vector sequences. Insect-Tolerant Crops: Bt Maize Insects and Insect Control Crop losses due to insects are experienced by all farmers, regardless of the crop, location, or year. For example, the European corn borer (Ostrinia nubilalis) is a major pest causing up to 10% crop loss per year in North America. The cotton bollworm and Colorado potato beetle are other examples of common insects that have inflicted major losses in these crops over the years. Plants have evolved a range of effective strategies to ward off insects. Resistance to insects, regardless of mechanism, has undoubtedly been a major objective in the long process of plant domestication and continues to this day to be an important selection criterion in plant-breeding programs. Most pesticides are nonproteinaceous, synthetic compounds that persist in the environment; they are also frequently applied as foliar sprays, which have drift problems, and are ineffective on insects protected by various plant parts or burrowed into tissues. Not only are such chemicals costly, but also constitute a hazard to the health of those working with them, as well as other humans and nontarget organisms; insects also develop resistance to pesticides, adding to the problems associated with this strategy. In addition to breeding crops to enhance natural resistance to insects, there has been a concerted effort to exploit other sources for natural pesticides, such as pyrethrins from seed cases of chrysanthemum. Another source is Bacillus thuringiensis, first recorded as the causal agent of sotto disease in silkworm by a biologist in Japan in 1901; it was given its current designation by German researchers in 1911, when it was isolated from the pupae of flour moths and other insect larvae in stored grains in the city of Thuringen. In adverse conditions, these bacteria form spores containing crystals of Bt protein, which can comprise as much as 30% of the spore dry weight. Toxin-producing B. thuringiensis species occur widely in soils, being especially prominent in insect-rich environments, such as silk farms, flourmills, and grain storage facilities. There are Bt proteins toxic to several genera of insects important to agriculture, as well as to mosquitos, and blackflies. For example, a protein from Bt ssp.kurstakii is toxic to lepidopterans, such as corn borer and cotton bollworm, and a protein from Bt ssp.tenebrionis is toxic to the Colorado potato beetle. Upon ingestion, these crystals are solubilized in the alkaline gut of sensitive insects and proteases cleave off the toxic fragment of about 650 amino acids from the amino end of the protoxin. Binding of the toxin to receptors on the surface of midgut epithelial cells causes cell disruption, and leakage of nutrients into the body cavity enabling spore germination and microbial growth. The specificity of each Bt protein depends on pH, proteases, and the presence of receptors. Several commercial formulations of Bt were produced in the United States, Germany, Russia, and France. Current consumption is estimated to be well in excess of $100 million annually, most of which is produced in low-cost fermentors using natural strains. At present, there are more than 100 commercial Bt products available in the United States, many of which are used by organic growers as it is considered to be a natural pesticide. These preparations are used mainly as sprays, although granular formulations are also available; the latter have been deployed in ponds to control mosquitos and blackflies. As a consequence of the mode of action of Bt proteins, they are nontoxic to other organisms, a very attractive and unusual feature for any insecticide. There are some limitations, however, to this organic approach to pest control. The Bt protein breaks down fairly quickly, which is considered an advantage in that there is not a buildup of pesticide in the soil and or on the product. However, it also means that multiple applications are sometimes necessary over the growing season. Spray applications are notoriously inefficient as insects can hide on the underside of leaves or burrow into the stem. Bt proteins are very insect specific in their activity, which is desirable from the perspective of not harming nontarget insects, but problematic when a grower is faced with multiple insect pests. Finally, insects can develop resistance to Bt proteins, requiring the use of different Bt proteins or other control strategies. Isolation, Modification, and Transfer of Bt Genes Given the interest in these proteins for controlling insects, it is not surprising that with the advent of molecular techniques in the late 1970s, Bt genes were an early target for isolation and engineering. Extensive screening for new Bt genes, as well as other proteins with biological activity against insects and other pests, remains a very active area of research and development, in attempts to combat a broader range of insects, find new proteins with more potent activities, or alternatives when resistance to proteins currently used arise, which is almost certain to occur. The strategy to control insects in crops by expressing Bt genes in the plants is attractive since. The Bt toxin is produced and contained within the plant tissue, and is present at all times to be consumed by an insect only when it feeds on that tissue, a far superior strategy to intermittent spraying. Moreover, the insect cannot escape the insecticide by hiding under the leaves, in whorls or burrowing into the stem. The expression of the Bt gene can also be targeted to specific tissues, such as the roots, for pests such as the corn rootworm, thereby eliminating the metabolic cost associated with synthesis in the rest of the plant, where it serves no function. CIBA GEIGY, later Novartis Seeds and now Syngenta, was a pioneer in developing commercial maize hybrids containing a Bt gene coding for a variant of an endotoxin, CryIA(b) from B. thuringiensis var. kurstaki, which was chosen for control of European corn borer. The larvae of this pest inflict losses to the maize crop to varying degrees in all regions of the world. In order to increase the level of the Cry protein in the maize plants, the Syngenta scientists reengineered the Bt gene. The native gene coding for the protoxin was truncated so that only the toxin was synthesized in planta. They also modified the codons, replacing the original microbial codons with those more commonly used for maize proteins; in addition, where possible, they employed G–Crich codons, thereby increasing the G–C content of the coding region from 38% to 65%. These modified Bt genes were introduced into elite-inbred lines by means of particle bombardment of immature zygotic embryos and regeneration under selection with phosphinothricin. The levels of Bt protein in the plants ranged from 0.1% to 0.4% of total soluble protein, depending on tissue and stage of development; these levels were several fold greater than those previously reached, and presumably due to the combination of gene modifications and use of strong promoters. In preliminary trials in 1992, both tunneling and leaf feeding were substantially reduced compared to the inbred parent lines, demonstrating a commercially feasible means of reducing yield losses due to borer infestation. Syngenta released its first maize hybrids with this Bt gene in the United States in 1996, as did Mycogen and Monsanto with their own versions. Chapter 4: Transgenic Chapter 2. Crops in Africa: (B) Current status and future prospects DAVID KEETCH INTRODUCTION Food production and poverty reduction are among the main goals in efforts to promote socio-economic development in Africa. However, the ability to increase food production through expanding the current area under cultivation, increasing the application of agro-chemicals and extending the use of irrigation is limited. The key for Africa's future is rather to increase the per hectare yield of crops and to achieve this Africa needs to adopt technologies that will raise the production capacity of resource-poor farmers with access to few external inputs. The use of biotech crops has been identified as one of the technologies that can help Africa increase crop yields and/or reduce production costs and post-harvest loses. However, by 2012 only four African countries had commercialized biotech crops. A common concern of many national authorities in Africa is that the products of modern biotechnology might affect a nation’s health and biodiversity, and thus raise health and environmental safety issues. However, transgenic crops (also known as genetically modified, genetically engineered or biotech crops) such as maize, soybean, rapeseed and cotton are being approved for commercial use in an increasing number of countries. From 1996 to 2013, there was more than a 100-fold increase in the area grown with transgenic crops worldwide, reaching a total of 175.3 million hectares in 2013. At this time there were 19 countries that grew 50 000 hectares or more of biotech crops. These countries were the USA, Brazil, Argentina, India, Canada, China, Paraguay, South Africa, Pakistan, Uruguay, Bolivia, the Philippines, Australia, Burkina Faso, Myanmar, Spain, Mexico, Columbia and Sudan in decreasing order of area of biotech crops cultivated. Table 1 shows the 2012 global adoption rates for the four principal biotech crops (James 2012) and economic benefits for 1996-2011 and 2011 (Brookes & Barfoot, 2013). Table 1: 2012 global adoption rates for biotech soybean, maize, cotton and canola and the economic benefits for 1996-2011 and 2011 only. Global area planted in 2012 Economic benefits Crop Total area Area to biotech % biotech 1996-2011 2011 Soybean 100 million ha 80.7 million ha 81 $32.2 billion $3.9 million Maize 159 million ha 55.1 million ha 35 $30 billion $8.6 million Cotton 30 million ha 24.3 million ha 81 $32.5 billion $6.7 million Canola 31 million ha 9.2 million ha 30 $3.1 billion $0.42 million So far, most commercialisation has focused on these four crops, and the genetic engineering has involved two traits: insect resistance (IR) and herbicide tolerance (HT). Globally, a total of 18 million farmers grew biotech crops in 2013 with 16.5 million or 90% being small resource-poor farmers from developing countries (James, 2013). ~ 16 ~ The trend for an increase in the global area planted to transgenic crops seems set to continue, given the large range of biotech crops in research and development. In all countries official national approval has to be obtained for the commercial use of a biotech crop – whether for planting and growing or for use in human or animal foods. Such approval is always based on a safety assessment by the national authority, which in turn is based on scientific information regarding the crop, its specific trait and the receiving environment. It is important, therefore, for African governments to implement effective biosafety systems to ensure that good quality safety information is publicly available and, where possible, to adopt international approaches to risk and safety assessment that ensure the efficiency of the risk assessment process. Efficient biosafety systems are also important to protect agricultural and animal biodiversity and minimise unintended effects from influencing agricultural productivity as well as human health. In developing countries it is common to not only check safety, but to also check the potential socio-economic impact prior to giving approval for release of a biotech crop. Such socio-economic assessments are generally made under the same conditions as the biosafety assessments. The processes for biosafety assessments and biotech crop approvals are developing in tandem and are being implemented in countries around the world. There are also international regulations in place to ensure that biotech crops and their products are transported safely between countries and not released in areas where their health and environmental safety has not been reviewed. BIOTECH CROPS IN AFRICA By 2012 four African countries had commercialized biotech crops: South Africa, Burkina Faso, Egypt and Sudan (James. 2012). These four countries together planted 3.2 million hectares (ha) of biotech crops. In 2013, however, only South Africa, Burkina Faso and Sudan grew GM crops as the Egyptian Government placed a temporary planting restriction on Ajeeb YG pending further review. South Africa In 2013, 2.85 million ha of biotech crops were planted compared to 2.9 million ha in 2012. The total maize area in 2013 was 2.73 million ha, a slight reduction from 2012 (2.83 million ha) due to drought. The area of biotech maize in 2013 was 2.36 million ha compared to 2.42 million ha in 2012 Over 18 million ha of GE maize (white and yellow) were planted in the period 2001 to 2013 without a single adverse report or a negative effect on human health, animal health or the environment. The total area planted to soybeans increased from 500, 000 ha in 2012 to 520, 000 ha in 2013. Of this, the adoption rate of HT soybeans was 92% (478, 000 ha). The total cotton area was 8,000 ha, with the adoption rate of GE cotton reaching 100%, 95% of which was the stacked Bt/HT traits and the remainder the HT trait which was used as a mandatory refuge. Maize Of the total maize area of 2.73 million ha, 86.6% or 2.364 million ha were GE. Of this 2.364 million ha GE maize, 28.4% or 680, 342 ha were the single Bt gene and 18.2% or 409,032 ha HT. The remainder, 53.4% or 1.274 million ha was planted with stacked Bt/HT traits. White maize was planted on 1.580 million ha, 83.7% or 1.322 million ha were GE. Maize with a single Bt gene at 412, 707 hectares, HT trait at 165, 347 ha and Bt/HT stacked traits at 744, 725 ha. The yellow maize planting of 1.150 million ha comprised 90.5% or 1.041 million ha of GE, with 25.7% or 267, 635 ha for the single Bt trait, 22.4% or 243, 684 ha for HT, and 50.9% or 530, 065 ha for the stacked Bt/herbicide tolerant traits. ~ 17 ~ Three trends have emerged from the collected data: 1. The adoption rate of biotech maize is very similar for both white and yellow maize. 2. The adoption rate of traits (insect resistance, herbicide tolerance and stacked) is similar for white and yellow maize. 3. The adoption rate is reaching saturation. This is because not all maize plantings require Bt insect resistance due to cost saving when pesticide can be applied through overhead irrigation, when necessary. Furthermore, some plantings are not subject to severe stalk borer infestation. Over 92% of maize samples tested positive for GE traits, pure GE or co-mingled. A niche market has developed for non-GE maize. Soybean In 2013 soybean was planted on 520, 000 ha. HT soybean was planted in 478, 000 ha or 92% of the total area planted. Cotton Almost 8, 000 ha was planted to cotton in 2013. All of the cotton planted was biotech with 95% stacked (Bt/HT) and 5% RR used in ‘refugia’. The stacked BtRR (Bollgard®II RR) was replaced with BtRRFlex. Virtually no conventional cotton was grown. Approval of Biotech Crops The field trial approvals for 2013 included: Biotech maize with drought tolerance; Stacked insect resistance/drought tolerance; Biotech cotton with insect resistance/drought tolerance; Biotech sugarcane with altered sugar ; and Cassava. Economic Benefits The economic gains from biotech crops for South Africa for the period 1998 to 2013 was US$1.15 billion and US$218.5 million for 2012 alone (Brookes and Barfoot, 2014). Studies have reported farm-level benefits that have translated into increased adoption rates. Yield gains exceeding 40% have been reported to in comparison with conventional cotton in addition to reduced spraying costs by 42%, reduced number of pesticide sprayings from 10 to 4 sprays per season, reduced production costs resulting in higher gross margins ranging from US$ 70–130 /2 ha of cotton (Ismael et al., 2002; Morse et al., 2005; AfricaBio, 2007). A study by Gouse et al. (2005) on Bt maize involving 368 small-scale and resource-poor farmers compared to 33 commercial farmers was quite revealing. The commercial farmers were grouped into two, those cultivating under irrigation and those under rain-fed production systems. Higher yields were observed for farmers who cultivated under irrigation systems. This group obtained 12.1 MT /ha, an 11% increase over the previous year’s yield. These farmers also obtained cost savings in insecticide use of US$18/ha representing a 60% reduction and an increased income of US$117/ha. Farmers who grew Bt maize under rain-fed conditions obtained 3.4 MT/ha, also an 11% yield gain over the previous year’s yield. Cost savings on insecticide use for this group was US$7/ha representing a 60% reduction and the combined effect was an increase in income of US$35/ha. ~ 18 ~ The smallholder Bt maize farmers group was compared to others who grew conventional hybrid and open pollinated maize varieties in terms of yield per hectare (Gouse et al., 2005). Bt maize recorded yield gains of 31% and 134% over conventional hybrids and open-pollinated varieties, respectively. Another study that used longitudinal study over 9-year period (2000 to 2008) reported that small-scale Bt maize farmers in South Africa gained an additional US$ 267 million (Gouse et al., 2008). Burkina Faso Cotton is the principal cash crop in Burkina Faso generating over US$ 300 million in annual revenues representing about 60% of the country’s export earnings (ICAC, 2006). Despite this achievement, the agricultural sector in the country is beset by a number of challenges including low yields, drought, poor soil, insect pests and lack of infrastructure and inadequate credit. Studies have also reported crop losses in excess of 30% due to insect-pests of cotton (Goze et al., 2003; Vaissayre and Cauquil, 2000). At the national level, the annual cost for insecticides for the control of cotton bollworms and related insect- pests was around US$ 60 million (Toe, 2003 cited in Karembu 2009). However, insecticides proved ineffective, with losses due to bollworm as high as 40% even with full application of insecticides (Traoré et al., 2006). As a result of this damage, Burkina Faso’s cotton production decreased to 0.68 million bales in 2007/08 from 1.3 million bales in 2006/07. To address this situation and after 5 years of fields trials, approval was granted for the commercial cultivation of Bt cotton. In 2008, Burkina Faso planted approximately 9, 000 hectares of Bt cotton for seed production and initial commercialization, becoming the 10th country globally to grow commercial Bt cotton. Vitale et al. (2008) estimated that cultivation of Bt cotton would result in yield increases of 20% and a decreased need for insecticides that would generate US$ 106 million per year. Cotton In 2012, of a total of 615, 795 ha were planted to cotton, 313, 781 ha or 51%, were planted to Bt cotton. In 2013 a total of 474, 229 ha of Bt cotton were grown. There were about 100, 000 Bt cotton farmers in 2012, majority of whom were small-holder resource- poor farmers. Burkina Faso started to plant Bt cotton in 2008 with approximately 9, 000 ha, this area increased to 116, 000 ha in 2009, 260, 000 ha in 2010, 247, 000 in 2011, 313, 781 in 2012 and finally 474, 229 ha in 2013. The adoption rate of Bt cotton in Burkina Faso has increased from 2% of 475, 000 cotton ha in 2008 to 51% or 313, 781 ha in 2012. Economic Benefits In 2009, a survey conducted by Vitale et al. (2010) showed that: The yield advantage of Bollgard®ll over conventional cotton was 18.9%. Yield increase plus labour and insecticide savings (2 rather than 6 sprays) resulted in a gain of US$65.57 per ha compared with conventional cotton; this translated to a 206% increase in cotton income. The main benefit of Bollgard®II derives from the increase in yield whereas the reduction of production costs associated with four less insecticide sprays is offset by the higher cost of the seed. Extrapolating from the 2011 data the national benefit for biotech cotton in 2012 was about $30 million. ~ 19 ~ In 2011 the average yield increase for Bt cotton over conventional cotton was 19.7% and insecticidal sprays were reduced from 6 to 2. Profit increase by 5% to US$95.25/ha. Extrapolating the data from 2011, the national benefit from Bt cotton in 2012 was about US$30 million. Egypt In 2012, Egypt planted 1, 000 ha of Bt yellow maize (IR MON 810) known in Egypt as Ajeeb YG® compared to 2, 800 ha in 2011. In 2013, Egypt officially did not grow any GM maize due to a temporary restriction imposed by the Egyptian Government. Egypt was the first North African state to adopt biotech crops when it planted Bt maize in 2008 on 700 ha. Maize Egypt grew approximately 660,000 hectares of conventional maize in 2010, and imports annually 4.5 million tons of yellow maize valued at US$1.3 billion. Of the 660, 000 ha of maize, 160, 000 ha (25%) are yellow maize and 500, 000 ha are white maize. The biotech maize hybrid is resistant to three maize insect pest borers (Massoud 2005). Field trials that were conducted in Egypt from 2002 to 2007 indicated that the yield of Bt yellow maize could be increased up to 30% over conventional yellow maize hybrids. Economic Benefits In 2009, for IR Bt maize there was an increase in yield of US$267 per ha, plus an insecticide saving equivalent to US$89 per ha. This gave a total gain of US$356 per ha, minus the additional cost of seed per ha at US$75 for a net benefit per ha of US$281. Extrapolating from these data, the economic benefits from planting 1, 000 ha of Bt maize in 2012 is about US$281, 000. Sudan In 2012, Sudan became the fourth African country to commercialise a biotech crop – Bt cotton. Cotton A total of 20,000 ha of Bt cotton were planted in the Sudan by about 10,000 smallholder farmers. The GM cotton variety planted is named “Seeni 1”and was developed in China. The availability of GM cotton seed was a limiting factor in 2012, but in 2013 the area tripled from 20,000 ha to 62,000 ha and is expected to expand even further. Sudan In 2012, Sudan became the fourth African country to commercialise a biotech crop – Bt cotton. Cotton A total of 20 000 ha of Bt cotton were planted in the Sudan by about 10 000 smallholder farmers. The GM cotton variety planted is named “Seeni 1”and was developed in China. The availability of GM cotton seed was a limiting factor in 2012, but in 2013 the area tripled from 20,000 ha to 62,000 ha and is expected to expand even further. ~ 20 ~ STATUS OF BIOTECH CROPS IN AFRICA The current status of biotech crops in Africa is shown in Table 2 (James, 2012). Table 2: The current status of biotech crops in Africa in 2012. Country Crop Trait Stage Burkina Faso Cotton Insect resistance Commercialised Cowpea Insect resistance Field trials Sorghum Insect resistance Field trial pending Egypt Maize Insect resistance Temporary restriction Insect resistance Field trials Cotton Insect resistance Field trials Potato Insect resistance Field trial postponed Viral resistance Field trial postponed Rice Insect resistance Greenhouse trials Strawberry Viral resistance Greenhouse trials Sugarcane Insect resistance Field trials Fungal resistance Field trials Wheat Drought tolerance Field trials postponed Fungal resistance Field trials Salt tolerance Field trials postponed Tomato Vial resistance Greenhouse trials Salt tolerance Greenhouse trials Ghana Cowpea Insect resistance Trial approval pending Nigeria Cowpea Insect resistance Field trials Cassava Biofortified Field trials Sorghum Biofortified Field trials Kenya Maize Insect resistance Field trials Drought tolerance Field trials Cotton Insect resistance Field trials Cassava Viral resistance Field trials Biofortified Field trials Sweet potato Viral resistance Field trial pending Sorghum Biofortified Field trials Malawi Cotton Insect resistance Field trials Mozambique Maize Drought tolerance Trial approval pending Uganda Cotton Insect resistance/ herbicide tolerance Field trials Banana Nematode r5esistance Field trials Bacterial wilt Field trials Biofortified Field trials Maize Insect resistance Field trials pending Drought tolerance Field trials Cassava Viral resistance Field trials Sweet Potato Viral resistance Field trials pending Insect resistance Greenhouse trials South Africa Maize Insect resistance Commercialised Herbicide tolerance Commercialised Insect resistance/ herbicide tolerance Commercialised Insect resistance Field trials Herbicide tolerance Field trials Insect resistance/ herbicide tolerance Field trials Drought tolerance Field trials Cassava Starch enhanced Field trials Cotton Insect resistance Commercialised ~ 21 ~ Herbicide tolerance Commercialised Insect resistance/ herbicide tolerance Commercialised Insect resistance/ herbicide tolerance Field trials Herbicide tolerance Field trials Potato Insect resistance Field trials Sorghum Biofortified Greenhouse trials Soybean Herbicide tolerance Commercialised Sugarcane Alternative sugar Field trials Tanzania Maize Drought tolerance Trial approval pending FUTURE PROSPECTS The largest group of potential beneficiary countries that have yet to adopt and benefit from biotech cotton are in sub-Saharan Africa where at least 15 countries, each growing more than 100 000 ha of cotton, for a total of almost 4 million ha of cotton could benefit significantly, plus Egypt in North Africa. Future prospects for Africa look encouraging with Cameroon, Egypt, Ghana, Kenya, Nigeria, Malawi and Uganda presently conducting field trials with biotech crops. Trials focusing on Africa’s priority staple crops, such as maize, cassava, cowpea, banana, sorghum and sweet potato, have made good progress while trials are underway in Kenya, South Africa and Uganda on drought tolerant maize through the WEMA (Water Efficient Maize for Africa) project. Biotech crops have the potential to make a substantial contribution to achieving the 2015 Millennium Development Goals (MDG) of cutting poverty in half, by optimizing crop productivity. This goal can be expedited by public-private sector partnerships, such as the drought tolerant maize for Africa project supported by the Bill and Melinda Gates Foundation. Globally, there are numerous new products at different stages of research and development. They include: Insect resistance – high priority is now being given to the control of sucking insect pests as they have become the top priority now that the previously top priority bollworm pests are effectively controlled by current Bt crops. Disease resistance to the pathogens Fusarium, Rhizoctonia, Pythium and cotton leaf curl virus. Crops that are more resistant to abiotic stress such as drought tolerant maize and cotton that is more tolerant to salinity, high and low temperatures and water logging. Crops with greater efficiency to use soil nutrients. Cotton with improved fibre, better oil quality and gossypol-free seed. Climate change is a major threat to sustainable growth and development in Africa, and the achievement of the MDG. According to the Intergovernmental Panel on Climate Change (IPCC 2012), the impact of climate change is expected to be greater in low latitude sub-tropical and tropical developing nations, where farmers have more limited ability to adapt. Africa is particularly vulnerable to the effects of climate change including reduced agricultural production, worsening food security, the increased incidence of flooding, drought and disease and an increased risk of conflict over scarce land and water resources (OECD 2007). The importance of climate change and concerns about the environment have implications for the future of biotech crops, which contribute to a reduction of greenhouse gases and help mitigate climate change by: Reducing carbon dioxide (CO2) emissions through reduced use of fossil-based fuels, associated with fewer insecticide and herbicide sprays; Promoting the use of conservation tillage (need for less or no ploughing facilitated by herbicide tolerant biotech crops) for GE food, feed and fibre crops. ~ 22 ~ With climate change, droughts, floods, and temperature changes predicted to become more prevalent and more severe in Africa, there will be a need to develop crop varieties and hybrids that are well adapted to more rapid changes in climate. Several biotech tools, including tissue culture, diagnostics, genomics, molecular marker-assisted selection (MAS) and biotech crops can be used collectively to speed up the breeding process. One factor that is hindering a faster adoption of biotech crops is the current low level of awareness of biotechnology and biosafety in Africa. At present only 12 African countries (Burkina Faso, Ghana, Kenya, Malawi, Mali, Mozambique, Nigeria, South Africa, Tanzania, Togo, Uganda and Zimbabwe) have established regulatory systems, 5 countries are at an interim stage and 32 countries have little or no interest in developing regulatory systems (ABNE, 2012). To counter this situation, vigorous awareness activities are needed. Capacity building for GE and non-GE products must be enhanced. There is also a need for the regional harmonization of biosafety legislation and regulations. It is crucial that Africa accepts biotechnology and develops products that will be appropriate to its needs. Above all, Africa must develop the capacity to be its own spokesperson on the safety and the risks of biotech crops. CONCLUSION The capacity of biotech crops to contribute to the sustainability of food production in Africa is significant and it can help the continent overcome the formidable challenges associated with climate change and global warming. Biotech crops can also increase productivity and income, and hence serve as an engine for rural economic growth that can contribute to the alleviation of poverty for Africa’s small scale and resource-poor farmers. REFERENCES ABNE 2012, ABNE in Africa: Building Functional Biosafety Systms in Africa. African Union and NEPAD, pp 26. AfricaBio, 2007. Agricultural Biotechnology: Facts for Decision Makers. www.africabio.org Brookes, G. and Barfoot, P. 2013. The global income and production effects of genetically modified (GM) crops 1996– 2011. GM Crops and Food: Biotechnology in Agriculture and the Food Chain. 4: 74 – 83. Brookes, G. and Barfoot, P. 2014. The global income and production effects of genetically modified (GM) crops 1996– 2012. Forthcoming. Gouse, M., C.A. Pray, J. Kirsten and D. Schimmelpfennig. 2005. A GM subsistence crop in Africa: The case of Bt white maize in South Africa. Int. J. Biotechnology, Vol.7, nos. 1/2/3. Gouse, M., Kirsten, J.F. & Van Der Walt, W.J. 2008. Bt Cotton and Bt Maize: An Evaluation of Direct and Indirect Impact on the Cotton and Maize Farming Sectors in South Africa. Report Commissioned by the South African Department of Agriculture. Directorate Biosafety, Harvest House, Pretoria, South Africa. Goze, E., Nibouche, S. and Deguine, J. 2003. ‘Spatial and probability distribution of Helicoverpa armigera (Lepidoptera: Noctuidae) in cotton: systematic sampling, exact confidence intervals and sequential test’, Environmental Entomology, Vol. 32, No. 5, pp.1203–1210. http://ictsd.net/downloads/2008/04/biotech_eastern_africa.pdf#page=47 Ismael, Y., R. Bennett and S. Morse 2002. Benefits from Bt Cotton Use by Smallholder Farmers in South Africa AgBioForum, 5(1): 1-5. James, C. 2012. Global Status of Commercialized Biotech/GM Crops: 2012. ISAAA Brief No. 44. ISAAA: Ithaca, New York. James, C. 2013. Global Status of Commercialized Biotech/GM Crops: 2013. ISAAA Brief No. 46. ISAAA: Ithaca, New York. Karembu, M., F. Nguthi and H. Ismail, Biotech Crops in Africa: The Final Frontier, ISAAA AfriCenter, Nairobi, Kenya, 2009. IPCC, 2012 Managing the Risks of Extreme Events and Disasters to Advance Climate Change Adaptation A Special Report of Working Groups I and II of the Intergovernmental Panel on Climate Change [Field, C B , V. Barros, T.F. Stocker D Qin D J Dokken K L Ebi, M D Mastrandrea K J Mach G.-K Plattner S K Allen M. Tignor, and P M Midgley (eds.)] Cambridge University Press Cambridge UK and New York NY USA 582 pp. ~ 23 ~ Chapter Chapter 5: 3. Environmental biosafety issues associated with GE crops in Africa MOUSSA SAVADOGO INTRODUCTION Africa is endowed with rich and varied biological resources (UNEP 2010). At the same time the continent is faced with environmental challenges that negatively affect its economic progress. Among the most serious environmental concerns are deforestation, desertification, degradation and fragmentation, air and water pollution and loss of soil fertility (http://wikis.lib.ncsu.edu/). These cause a dramatic decline in local biodiversity and such concerns are aggravated by poverty and the need for survival that inevitably lead to the over-exploitation of local natural resources. It is estimated that if such a trend is maintained the environmental problems could double or triple by 2025 when the total population of the continent reaches over one billion (http://www.articlesbase.com/). It is hoped, however, that with the advent of new agricultural technologies including genetic engineering, farmers will have the opportunity to use more efficient practices that will significantly reduce the pressure on the environment through increased agricultural productivity and a reduction of chemical pesticides use. Indeed on the 17th anniversary of the first commercial cultivation of a genetically engineered crop, it was estimated that this technology had contributed globally to a better environment by saving considerable amounts of pesticide active ingredient, reducing CO2 emissions and conserving biodiversity on millions of hectares of land (James, 2013). Paradoxically, in Africa the adoption of the same technology is being slowed down if not rejected for the same reason – the need to protect the environment that the new agricultural technology will help save. However, African countries such as Burkina Faso, which have taken the lead in adopting Bt cotton, have seen their environmental conditions improve through a significant reduction in the use of chemical pesticides (Vitale et al. 2011). This chapter briefly reviews the environmental issues, especially those associated with the loss of biodiversity in Africa, the perceptions on the impact of GE crops on the environment, the GE crops of interest to Africa that are either commercially cultivated or under development and the critical information necessary to perform an assessment of their potential for gene flow and weediness. CRITICAL ENVIRONMENTAL ISSUES IN AFRICA The environment of sub-Saharan Africa is affected by various factors including deforestation, desertification, degradation and fragmentation, all of which are responsible for a dramatic loss of biodiversity. Deforestation refers to the clearing and destruction of forests in order to create land for agriculture, provide wood for domestic needs of energy and/or create space for building settlements (http://wikis.lib.ncsu.edu/index.php/). It is estimated that about one million km2 of forest is destroyed every 5 to 10 years and as a result the forest land has declined from 16 million km2 a century ago to only half today (Braun and Ammann, 2003). Between 1981 and 1990 around 25% of African forested land was converted into agricultural land (Mabogunje, 1995). However in a number of countries, including Nigeria and Niger, governments have been making efforts to mitigate such extensive loss of forest by planting trees and trying to preserve existing ecosystems (Niamey, 2005). Desertification refers to the expansion of desert conditions as a result of many factors including drought and the overuse of local ecosystems. Degradation refers to the deterioration in the density or structure of vegetation cover or species composition (Mabogunje, 1995). Selective logging without replanting, removal of ~ 25 ~ plants and trees that are of importance in the life cycle of other species and soil erosion are the main causes of degradation. Fragmentation is caused by various forms of human intrusion in forest areas, such as road construction. It causes increased vulnerability of natural ecosystems through changes in micro-climates and subsequent loss of native species and invasion by alien species (Mabogunje, 1995). Deforestation, degradation fragmentation and desertification all cause a sever degradation in natural habitat which is the primary threat to biodiversity, not only in Africa but also globally. In addition to habitat loss, pollution and invasive species are also considered major threats to wild biodiversity (Millennium Ecosystem Assessment 2005). To protect the environment, the main question for most African governments is how to reverse the loss of biodiversity caused by the destruction of the natural habitat. In answer to this question, Braun and Ammann (2003) suggested that the single most promising way to avoid habitat destruction was to increase farm yields in a process that has been called the “Second Green Revolution.” PERCEIVED CONCERNS ASSOCIATED WITH GE CROPS IN AFRICA The global community is concerned that modern agricultural biotechnologies such as genetic engineering, like other new technologies, may hold risks for the environment and/or human and animal health. For this reason, crops and products developed through genetic engineering are regulated to the extent of possibly making it the most regulated of all agricultural technologies (Braun and Ammann 2003). Concerns that are generally raised imply that the cultivation of GE crop varieties could negatively impact the environment through unintentional transfer of novel genes and traits to non-GE plants. This could result in the development of herbicide resistant weeds and/or more invasive weed species as well as the development of resistant pests. In addition, more specific concerns have been raised in Africa. It is thought that GE crops might have a negative impact on African traditional crops and land races and on the local biodiversity. This would result in an increased dependency of smallholder farmers on multinational seed companies. Africans fear that such a situation would give multinational companies full control on the continent’s genetic resources. Many othe