Molecular Neurophysiology Lecture Notes (FS 2024) PDF
Document Details
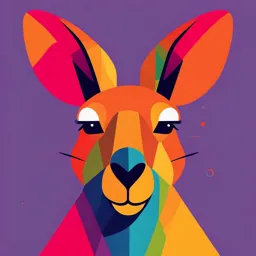
Uploaded by FreshFlerovium14
ETH Zurich
2024
ETH Zurich
Gerhard Schratt
Tags
Summary
These lecture notes cover molecular neurophysiology, focusing on excitable membranes and their role in the nervous system. The document includes schedules, concepts related to bioelectricity, and related books suitable for students taking neuroscience courses at the university level.
Full Transcript
Lecture Molecular Neurophysiology „Excitable Membranes“ ETH Zürich, 376‐1305‐01 V FS 2024 Prof. Dr. Gerhard Schratt D-HEST Systems Neuroscience Irchel-Ca...
Lecture Molecular Neurophysiology „Excitable Membranes“ ETH Zürich, 376‐1305‐01 V FS 2024 Prof. Dr. Gerhard Schratt D-HEST Systems Neuroscience Irchel-Campus, Y17-L48 [email protected] Molecular Neurophysiology: From Molecules to Systems General Information Semester: HS2024 Exam Time: Mondays, 10:15 ‐ 12:00 Time: Monday, January 13, 09:30 ‐ 11:00h Location: Campus Irchel Winterthurerstrasse 190, 8057 Zürich Location: ONA E7 (Örlikon) Lectures 1-3 (16.09.-30.09.): Gebäude Y21, Room F65 (“Theatersaal”) Electronic exam, multiple choice (K-Prime, Single Choice) Lectures 4-14 (07.10.-16.12.): Gebäude Y15, Room G19 Responsible Lecturer: Prof. Gerhard Schratt Schedule Introduction, Excitable Membrane and Action GS 16.09.2024 Potential Neurotransmitter Release JW 23.09.2024 Postsynaptic responses, trans-synaptic signaling JW 30.09. 2024 Neuronal signaling 1: Signal transduction and RF 07.10.2024 neuromodulation Neuronal signaling 2: Gene expression GS 14.10. 2024 No lecture 21.10.2024 Synaptic plasticity 1: Facilitation, LTP, LTD RF 28.10.2024 Synaptic plasticity 2: the hippocampal circuit, JW 04.11.2024 inhibition and oscillation Synaptic plasticity 3 : Associative fear memory RF 11.11.2024 formation Sleep 1: Circuit Physiology JW 18.11.2024 Sleep 2: Cellular and Molecular Mechanisms GS 25.11.2024 Seonsory Systems 1 WB 02.12.2024 Sensory Systems 2 WB 09.12.2024 Sensory Systems 3 WB 16.12.2024 Lecturer GS: Prof. Gerhard Schratt, WB: Dr. Wolfger von der Behrens, RF: Dr. Roberto Fiore, JW: Dr. Jochen Winterer Books The functioning of the nervous system relies on electrical processes Luigi Galvani (1737- 1789) > What is the biological basis of this “animal electricity”? Bioelectrical processes: „Electrophysiology“ In some cases, one can measure electrical activity auf cellular assemblies/organs relatively easily from „outside“: ECG (Electrocardiography, derivation from the heart) EEG (Electroencephalography, Derivation from the cerebral cortex) Bioelectrical processes: „Electrophysiology“ Vice versa, electrical processes in cells/organs can be induced/modulated by electrical stimulation Pacemaker Defibrillator Neural prostheses: Cochlear Implants Basis of all bioelectrical processes is the membrane potential Nomenclature: properly „membrane voltage“ = difference in potential, commonly referred to as membrane potential Symbol: VM = UM = EM Unit: mV Convention: Inside vs. outside(reference potential outside, ‚Mass‘ = 0 mV) Functions of the membrane potential Driving force for transport across Electrical signals: Nervous membranes (small intestine, kidney) system (including sensory organs), heart, etc. Trigger of secretory processes (e.g., insulin secretion) trigger of muscle activity (skeletal muscle, heart) Driving force of ATP synthesis (inner mitochondrial membrane) Requirements for the generation of a membrane potential 1. Electrical charges 2. Electrical insulator 3. Mechanism responsible for the transprort of charges from one side to the other Ions: Electrically charged molecules How do charges develop? Salt dissociates in water: The polar solvent water dissociates ionic bonds; salts are represented as electrically charged, hydrated particles (ions): cations (+), anions (-) Physiological salt solutions always contain equal amounts of positive and negative charge equivalents! Intra- and extracellular ion concentrations: Ion C (intra) C (extra)* Na+ 10 - 15 mM 145 mM K+ 140-155 mM 4,4 (3,6-4,8) mM Ca2+ ca. 0,0001 mM ca. 1,2 mM** Mg2+ 0,5 -1 mM ca. 0,7 mM Cl- 4 – 30 mM 100-120 mM HCO3- 16 mM 24 mM A- (e.g., proteins) ca. 150 mM ca. 5 mM * In part small differences between blood plasm and interstitium (extracellular space in the tissue) ** free concentration Requirements for the generation of a membrane potential 1. Electrical charges 2. Electrical insulator 3. Mechanism responsible for the transprort of charges from on side to the other Intra- and extracellular environment are separted by a plasma membrane Permeabilities of physiologically relevant solutes 1 cm/s 10-12 cm/s Cell membranes are permeation barriers for all polar molecules and are electrical insulators! Requirements for the generation of a membrane potential ? 1. Electrical charges 2. Electrical insulators 3. Mechanism responsible for the transprort of charges from on side to the other? How are concentration gradients generated? ‚Slow‘ transport across membranes: Transport molecules Na+K+-ATPase How is a membrane potential generated? 1. Concentration gradient for a given ion 2. Selective (!) permeability for the same ion Nernst How is a membrane potential generated? 1. Concentration gradient for a given ion 2. Selective (!) permeability for the same ion Nernst How is a membrane potential generated? 1. Concentration gradient for a given ion 2. Selective (!) permeability for the same ion Nernst How is a membrane potential generated? (thermodynamic) equilibration between a chemcical gradient (concentration) und an electrical gradient (membrane potential). No net motive force on the ion, the net ion flux across the membrane equals 0. Nernst Equation: Potential equilibrium: membrane voltage at the R.T c stage of an electrochemical Eion =.. ln ca equilibrium zion F i Example calculation: Equilibrium potentials for K+, Cl- [K+]i/[K+]a = 145 mM / 4,4 mM = 33 EK = -61 mV * log(33) = -61 mV * 1,52 = -93 mV [Cl-]i/[Cl-]a = 12 mM / 120 mM = 0,1 ECl = +61 mV * log (0,1) = +61 mV * (-1) = -61 mV (NB: here +61 mV, since zCl = -1) Summary Equilibrium potential: ▪ The equilibrium potential is defined as the membrane voltage, which occurs if 1. a concentration gradient exists for an ion and 2. the membrane is selectively permeable for this ion. ▪ The magnitude of the equilibrium potential is defined by the Nernst equation. ▪ The value of the equilibrium potential is only dependent on the concentration gradient for a given ion ▪ Ion fluxes during the generation of diffusion potentials under physiological conditions (membrane capacity, ion concentrations, membrane potentials reached) are typcially so small that the intra- and extracellular concentrations are not affected during this process. Signal transmission: The action potential When does a cell fire an action potential (AP)? APs are elicited by depolarization: Threshold potential The action potential Action potentials are characterized by stereotypic amplitudes and kinetics: „All-or- nothing principle“ Hereinafter, we will discuss the basic properties of action potentials: Cause of the rapid depolarization phase including the reversal of the membrane potential („Overshoot“) Triggering of APs by depolarization The reason for the existence of a threshold potential and the „all-or-nothing principle“ Classical experiments on the ionic mechanisms of APs using giant axons of the squid (Loligo) Loligo spec., engl.: squid Hodgkin & Huxley, 1952 The Nobel Prize in Physiology or Medicine 1963 "for their discoveries concerning the ionic mechanisms involved in excitation and inhibition in the peripheral and central portions of the nerve cell membrane" A. L. Hodgkin A. F. Huxley What are the mechanistic principles of De- and Re-polarisation? Alterations in membrane potentials mean a charge reversal of the membrane (= capacitor) → By ion flux across the membrane → 1. inward current – during Depolarisation → 2. outward current – during Repolarisation Na+- und K+-currents are characteristic of the AP >Voltage Clamp K+-Kanal-Blocker Na+-Kanal-Blocker TTX from Fugu Summary: Generation of an AP ▪ Depolarisation via a rapid voltage-dependent activation of the Na+ conductance ▪ Repolarisation via a delayed voltage-dependent activation of the K+ conductance and the inactivation of the Na+ conductance ▪ The decisive factor is the differential temporal behaviour of Na+ und K+- conductances (channels) NB: AP are not generated due to alterations in Na+ or K+ ion concentrations ! Why are APs following the „All-or-nothing-principle“? Why is there a threshold for triggering an AP? Analogy to a chemical explosion! Depolarisation leads to elevated permeability for Na+-Ions, further promoting depolarization (positive feedback) Delayed elevation of the K+-Ion permeability leads to a Repolarisation of the membrane (negative feedback) Why are Na+- und K+-currents voltage-dependent? The architecture of voltage-gated Na+ and K+-channels KV channels NaV-channels 1. Voltage-dependance of the single ion channel Open probability → The open probability of channels increases with augmenting depolarization 2. Molecular basis of the voltage-dependent switch-behaviour(‚gating‘) The S4-Helix as voltage sensor: S4-Helices of channel subunits harbor positively charged amino achid side chains and function as Voltage sensors. Voltage-dependent NaV-channels adopt 3 states 1. Behaviour of the Na+-current 3. In the Voltage-Clamp-Experiment 2. 1. 2. 3. Molecular Mechanism activation inactivation Nomenclature closed open inactivated Recovery after inactivation The consequence of Na+-current inactivation: Membrane refractory period Absolute refractory period: ca. 2ms activation inactivation closed open inactivated Recovery after inactivation Relative refractory period: ca. 1,5 ms Local anesthetics block Nav+-channels How is signal transmission within neurons achieved? The function of nerve cells: Signal processing and transmission Dendrite Soma Signal-Transmission: Action potentials Axon (nerve impulses) Signal Transfer: Synapse In electrically excitable cell assemblies (nervous system, heart, etc.), electrical signals are used for information transfer across long distances mm – cm - m Central nervous system (CNS) Peripheral nervous system (PNS) Muscle stretch reflex Heart Signal transmission without action potentials The passive* properties of the axon (the so-called cable properties) lead to a distance- dependent signal attenuation! *(Passive = no voltage-dependent conductances, also known as electrotonic signal transmission; no APs!) Signal transmission with action potentials Action potentials enable signal transmission without signal attenuation! Continuous, regenerative spread of APs along the axon 1. Elektrotonische Ausbreitung der Depolarisation (AP) auf benachbarte Abschnitte des Axons --- durch Stromfluss entlang des Axons 2. Dort Aktivierung von NaV- Kanälen und somit erneute regenerative AP-Bildung 3. Einseitige Laufrichtung durch Refraktarität bereits erregter Axon-Abschnitte. Analogy model: Fuse Ignition of neighboring parts of the cable unidirectional What determines the speed of the signal spread? To enable fast AP conductance, electronic spread should have a high range (large longitudinal constant ). rM l= ri 1. The smaller the internal resistance ri, the further away the AP threshold potential is reached, the faster the signal transmission. The internal resistance depends on the geometry of the axon: the larger the diameter, the smaller ri → Trick 1: huge fiber diameter (e.g., giant axons of the squid); different fiber diameters in vertebrates: fast conducting fibers are think, slow conducting ones are thin Axons with large diameter conduct faster! Squid We Cross-section through a peripheral nerve (electron microscopy) A: Axon, m: Myelin, N: Nucleus of a Schwann cell Scale bar: 0,3 µm Synapse Web, Kristen M. Harris, PI, http://synapses.clm.utexas.edu What determines the speed of the signal spread? To enable fast AP conductance, electronic spread should have a high range (large longitudinal constant ). rM l= ri 1...... 2. The bigger the membrane resistance rM, the further away the AP threshold potential is reached, the faster the signal transmission. → Trick 2: Myelinisation. Insulation by myelin sheets; Reduced expression of ion channels and concentration of the channels (and therefore the regenerative generation of APs) at nodes of Ranvier. Myelinated Axons Myelination by - Schwann cells in the PNS Concentration of voltage-dependent ion - Oligodendrocytes in the CNS channels at nodes of Ranvier Consequence of myelination: Saltatory signal transmission Summary conductance speed: Increased conductance speed through 1. Larger longitudinal constant due to smaller longitudinal resistance (fiber diameter) 2. Larger longitudinal constant due to higher membran resistance (myelinisation, concentration of ion channels at nodes of Ranvier) 3. Small time constant due to a Reduction in Membrane Capacity (Myelinisation) Mechanisms of AP conductance: 1. Continuous signal transmission: each part of the axon contributes to a regenerative action potential generation 2. Saltatory signal transmission: alternate parts of the axons serve the regeneration (node of Ranvier) and the purely electrotonic signal spread (myelin sheet) Fiber types and conductance speeds Terminology: afferent = to the CNS (sensory paths) efferent = from the CNS (motor paths) Demyelination diseases impair the transfer of APs (CNS: MS, PNS: Guillain-Barré-Syndrom)