Week 5 Reading PDF
Document Details
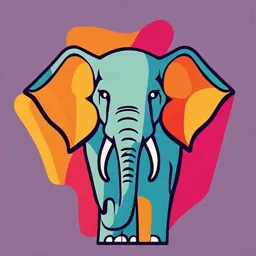
Uploaded by HotCanto
CUNY Macaulay Honors College
Tags
Summary
This document covers somatosensory pathways in the human nervous system.
Full Transcript
Chapter 7 Somatosensory Pathways A 71-year-old woman developed gradually worsening numbness and tingling in her right leg, along with weakness in her left leg. She also experienced occasional urinary incontinence. A neuro- logic examination revealed decreased pinprick sensation on...
Chapter 7 Somatosensory Pathways A 71-year-old woman developed gradually worsening numbness and tingling in her right leg, along with weakness in her left leg. She also experienced occasional urinary incontinence. A neuro- logic examination revealed decreased pinprick sensation on her right side below the level of the umbilicus and decreased vibra- tion and joint position sense in the left foot. Her left leg had brisk reflexes and was slightly weak. In this patient, these complex sensory and motor deficits arose from a single lesion. In this chapter, we will learn about the pathways for sensations such as touch, pain, and position sense of the limbs, and we will use this knowledge to accurately localize lesions of these pathways in clinical cases. 276 Chapter 7 ANATOMICAL AND CLINICAL REVIEW I N CHAPTER 6, WE DISCUSSED the anatomy of the corticospinal tract and other descending motor pathways. In this chapter we will discuss the other two major “long tracts” of the nervous system (Table 7.1). These are the so- matosensory pathways: the posterior column–medial lemniscal system and the anterolateral systems. Like the corticospinal tract, these pathways are somato- topically organized (see Figure 6.2). Understanding the functions and points of decussation of the three major long tracts (see Table 7.1) is fundamental to clinical neuroanatomical localization. In the sections that follow, we will learn to use the anatomy of the three ma- jor long tracts to localize lesions in the nervous system. We will discuss com- mon disorders of the spinal cord and other locations that affect these pathways. In addition, brainstem and spinal cord mechanisms of pain modulation will be addressed. The organization of the thalamus, serving as the major relay for sensory and other information traveling to the cortex, will be reviewed as well. Finally, we will discuss the roles of sensory and motor pathways in bowel, bladder, and sexual function. Main Somatosensory Pathways The term somatosensory generally refers to bodily sensations of touch, pain, tem- perature, vibration, and proprioception (limb or joint position sense). There are two main pathways for somatic sensation (see Table 7.1 and Figures 7.1 and 7.2): The posterior column–medial lemniscal pathway conveys proprioception, vibration sense, and fine, discriminative touch (see Figure 7.1). The anterolateral pathways include the spinothalamic tract and other asso- ciated tracts that convey pain, temperature sense, and crude touch (see Figure 7.2). Since some aspects of touch sensation are carried by both pathways, touch sen- sation is not eliminated in isolated lesions to either pathway. Four types of sensory neuron fibers are classified according to axon diam- eter (Table 7.2). These different fiber types have specialized peripheral recep- tors that subserve different sensory modalities. Larger-diameter, myelinated axons conduct faster than smaller-diameter or unmyelinated axons. Sensory neuron cell bodies are located in the dorsal root ganglia (see Figures 7.1 and 7.2). Each dorsal root ganglion cell has a stem axon that bifurcates, re- sulting in one long process that conveys sensory information from the periph- ery and a second process that carries information into the spinal cord through the dorsal nerve roots. A peripheral region innervated by sensory fibers TABLE 7.1 Main Long Tracts of the Nervous System from a single nerve root level is called a dermatome. NAME (AND LEVEL) The dermatomes for the different spinal levels form PATHWAY(S) FUNCTION OF DECUSSATION a map over the surface of the body (see Figure 8.4) Lateral Motor Pyramidal decus- that can be useful in localizing lesions of the nerve corticospinal sation (cervico- roots or spinal cord. In Chapters 8 and 9 we will dis- tract medullary junction) cuss localization based on dermatome and periph- Posterior column– Sensory (vibration, Internal arcuate fibers eral nerve patterns of sensory and motor loss. In this medial lemniscal joint position, (lower medulla) chapter we will focus on the central course of the so- pathway fine touch) matosensory pathways in the spinal cord and brain. Anterolateral Sensory (pain, Anterior commissure Just as our knowledge that the corticospinal tract pathways temperature, (spinal cord) crude touch) crosses over at the pyramidal decussation helps us localize CNS lesions (see Figure 6.11A), it is equally Somatosensory Pathways 277 Cerebrum Primary somatosensory cortex Thalamus Ventral posterior lateral nucleus of the thalamus Midbrain Medial lemniscus Secondary Pons sensory neuron Medial lemniscus Rostral medulla Nucleus gracilis (pathways from lower body) Caudal medulla Nucleus cuneatus (pathways from upper body) Internal arcuate fibers Vibration, Dorsal proprioception, root fine touch ganglion Dorsal (posterior) columns: Fasciculus gracilis Fasciculus cuneatus Cervical spinal cord Primary sensory neuron FIGURE 7.1 Posterior Column–Medial Lemniscal Pathway Dorsal root entry zone 278 Chapter 7 Cerebrum Intralaminar Primary and medio- somatosensory dorsal nuclei cortex Thalamus Ventral posterior lateral nucleus Superior colliculus of the thalamus Periaqueductal Spinothalamic gray tract Spinomesencephalic tract Midbrain Spinoreticular tract Pons Rostral medulla Caudal medulla Pain, Dorsal root temperature, ganglion crude touch Secondary sensory neuron Primary sensory neuron Lissauer’s tract Cervical spinal cord Dorsal root entry zone Anterolateral Anterior pathway FIGURE 7.2 Anterolateral Pathways commissure Somatosensory Pathways 279 TABLE 7.2 Sensory Neuron Fiber Types ALTERNATE FIBER NAME NAME DIAMETER (µm) MYELINATED RECEPTORS SENSORY MODALITIES A-α I 13–20 Yes Muscle Proprioception spindle Golgi tendon Proprioception organ A-β II 6–12 Yes Muscle Proprioception spindle Meissner’s Superficial touch corpuscle Merkel’s Superficial touch receptor Pacinian corpuscle Deep touch, vibration Ruffini ending Deep touch, vibration Hair receptor Touch, vibration A-δ III 1–5 Yes Bare nerve ending Pain Bare nerve ending Temperature (cool) Bare nerve ending Itch C IV 0.2–1.5 No Bare nerve ending Pain Bare nerve ending Temperature (warm) Bare nerve ending Itch important to know the points of decussation of the two main somatosensory pathways (see Table 7.1; Figures 7.1 and 7.2). We will therefore now trace the course of these pathways from spinal cord to primary somatosensory cortex. Posterior Column–Medial Lemniscal Pathway Large-diameter, myelinated axons carrying information about proprioception, vibration sense, and fine touch enter the spinal cord via the medial portion of the dorsal root entry zone (see Figure 7.1). Many of these axons then enter the ipsi- lateral posterior columns to ascend all the way to the posterior column nuclei in the medulla. In addition, some axon collaterals enter the spinal cord central gray mat- ter to synapse onto interneurons and motor neurons (see Figure 2.21). It is easier to remember the somatotopic organization of the posterior columns (Figure 7.3) if you picture fibers adding on laterally from higher levels as the posterior columns ascend. Thus, the medial portion, called the gracile fasciculus (gracile means “thin”) carries information from the legs and lower trunk. The more lateral cuneate fas- MNEMONIC ciculus (cuneate means “wedge shaped”) carries information from the upper trunk above about T6, and from the arms and neck. The first-order sensory neurons that have axons in the gracile and cuneate fasciculi (also called fasciculus gracilis and fasciculus cuneatus) synapse onto second-order neurons in the nucleus gra- cilis and nucleus cuneatus, respectively (see Figure 7.1). Axons of these second-order neurons decussate as internal arcuate fibers and then form the medial lemniscus on the other side of the medulla (see Figure 14.5). The medial lemniscus initially has a vertical orientation and then comes to occupy a progressively more lateral and inclined position as it ascends in the brainstem (see Figures 7.1, 14.3, and 14.4). The somatotopic organization of the medial lemniscus assumes a vertical position in the medulla such that the feet are represented more ventrally (“the little person stands up”) and then inclines again in the pons and midbrain such that the arms are represented more medially and legs more laterally (“the little person lies down”). Note the reversal in somatotopic orientation: for the medial lemniscus in the pons and MNEMONIC 280 Chapter 7 FIGURE 7.3 Somatotopic Organiza- midbrain the feet are lateral, while in the pos- tion of Posterior Column and Antero- terior columns the feet are medial. Recall that lateral Pathways in the Spinal Cord most somatotopic maps represent the upper Compare to somatotopic organization of Posterior columns body medially and lower body laterally, with the lateral corticospinal tract shown in (vibration and joint position sense): the notable exceptions of the posterior Figure 6.10C. (Spinal section from DeAr- Leg columns (see Figure 7.3) and primary sen- mond SJ, Fusco MM, Maynard MD. 1989. Structure of the Human Brain: A Pho- sory-motor cortices (see Figure 6.2). Lower trunk tographic Atlas. 3rd Ed. Oxford Univer- The next major synapse occurs when the sity Press, New York.) Upper trunk medial lemniscus axons terminate in the ven- Arm tral posterior lateral nucleus (VPL) of the thal- Gracile fasciculus amus. The neurons of the VPL then project Cuneate Neck through the posterior limb of the internal cap- fasciculus Occiput sule in the thalamic somatosensory radiations (see Figure 6.9B) to reach the primary so- matosensory cortex (see Figure 6.1, areas 3, 1, and 2) in the postcentral gyrus (see Figure 7.1). As we will discuss in Chapter 12 (see Figure 12.8), an analogous pathway called the trigem- Anterolateral inal lemniscus conveys touch sensation for the system face via the ventral posterior medial nucleus (pain and temperature): Neck of the thalamus (VPM) to the somatosensory Arm cortex. Synaptic inputs to the primary so- Trunk matosensory cortex from both the face and body occur mainly in cortical layer IV and the Leg deep portions of layer III, with some inputs also reaching layer VI (see Figure 2.14). Spinothalamic Tract and Other Anterolateral Pathways Smaller-diameter and unmyelinated axons carrying information about pain and temperature sense also enter the spinal cord via the dorsal root entry zone (see Figure 7.2). However, these axons make their first synapses immediately in the gray matter of the spinal cord, mainly in the dorsal horn marginal zone (lam- ina I) and deeper in the dorsal horn, in lamina V (see Figure 6.3B; Table 6.2). Some axon collaterals ascend or descend for a few segments in Lissauer’s tract before entering the central gray (see Figures 6.4 and 7.2). Axons from the second-or- der sensory neurons in the central gray cross over in the spinal cord anterior (ventral) commissure to ascend in the anterolateral white matter. It should be noted that it takes two to three spinal segments for the decussating fibers to reach the opposite side, so a lateral cord lesion will affect contralateral pain and temperature sensation beginning a few segments below the level of the lesion. The anterolateral pathways in the spinal cord have a somatotopic organization (see Figure 7.3) in which the feet are most laterally represented. To help you remember this organization, picture fibers from the anterior commissure adding MNEMONIC on medially as the anterolateral pathways ascend in the spinal cord. This soma- totopic organization, with arms more medial and legs more lateral, is preserved as the anterolateral pathways pass through the brainstem. When they reach the medulla, the anterolateral pathways are located laterally, running in the groove between the inferior olives and the inferior cerebellar peduncles (see Figures 7.2 and 14.5). They then enter the pontine tegmentum to lie just lateral to the medial lemniscus in the pons and midbrain (see Figures 14.3 and 14.4). The anterolateral pathways consist of three tracts (see Figure 7.2): the spinothalamic, spinoreticular, and spinomesencephalic tracts. The spino- thalamic tract is the best known and mediates discriminative aspects of pain and temperature sensation, such as location and intensity of the stimulus. Like the posterior column–medial lemniscal pathway, a major relay for the Somatosensory Pathways 281 spinothalamic tract is in the ventral posterior lateral nucleus (VPL) of the thala- mus (see Figure 7.2). However, the terminations of the spinothalamic tract and the posterior column–medial lemniscal pathway reach separate neurons within the VPL. From the VPL, information travelling in the spinothalamic tract is again conveyed via the thalamic somatosensory radiations (see Figure 6.9B) to the primary somatosensory cortex (see Figure 6.1, areas 3, 1, and 2) in the post- REVIEW EXERCISE central gyrus (see Figure 7.2). Pain and temperature sensation for the face is carried by an analogous pathway called the trigeminothalamic tract, to be dis- At what level does the decussation cussed further in Chapter 12 (see Figure 12.8). occur for the posterior column–me- There are also spinothalamic projections to other thalamic nuclei, including dial lemniscal pathway (see Figure intralaminar thalamic nuclei (central lateral nucleus) and medial thalamic nu- 7.1) and for the anterolateral path- clei such as the mediodorsal nuclei (see Figure 7.2). These projections proba- ways (see Figure 7.2)? Suppose that bly participate together with the spinoreticular tract in a phylogenetically older a patient has a lesion of the left half pain pathway responsible for conveying the emotional and arousal aspects of of the spinal cord. Which side of the pain. The spinoreticular tract terminates on the medullary–pontine reticular patient’s body will have decreased formation, which in turn projects to the intralaminar thalamic nuclei (centro- vibration and joint position sense median nucleus). Unlike the VPL, which projects specifically in a somatotopic below the level of the lesion? Which fashion to the primary sensory cortex, the intralaminar nuclei project diffusely side of the patient’s body will have to the entire cerebral cortex and are thought to be involved in behavioral decreased pain and temperature arousal (see the section on the thalamus later in this chapter). The spinomesencephalic tract projects to the midbrain periaqueductal gray sensation below the level of the le- matter and the superior colliculi (see Figure 7.2). The periaqueductal gray par- sion? Which side will have weakness ticipates in central modulation of pain, as we will discuss shortly. below the level of the lesion (see The spinothalamic and spinomesencephalic tracts arise mainly from spinal Figure 6.8)? Which side will these cord laminae I and V, while the spinoreticular tract arises diffusely from in- deficits be on if the lesion is in the termediate zone and ventral horn laminae 6 through 8 (see Figure 6.3). In ad- left cerebral cortex? dition to pain and temperature, the anterolateral pathways can convey some crude touch sensation, therefore, touch sensation is not lost when the poste- rior columns are damaged. To summarize, if you step on a thumbtack with your left foot, your spinothal- amic tract enables you to realize “something sharp is puncturing the sole of my left foot”; your spinothalamic intralaminar projections and spinoreticular tract MNEMONIC cause you to feel “ouch, that hurts”; and your spinomesencephalic tract leads to pain modulation, allowing you eventually to think “aah, that feels better.” A summary of spinal cord sensory and motor pathways is shown in Figure 7.4. The sensory pathways shown on the left are discussed in this chapter; the Ascending pathways Descending pathways spinocerebellar tracts are covered in Chapter 15; and the motor pathways Fasciculus Medial Tectospinal Fasciculus gracilis vestibulospinal tract shown on the right are discussed in cuneatus tract Chapter 6 (see Figure 6.11). As we will see in KCC 7.4, clinical syndromes of the Lateral spinal cord provide a practical review of Dorsal corticospinal spinocerebellar tract regional spinal cord anatomy. We will tract now discuss the continuation of the so- Rubrospinal matosensory pathways, from the thala- tract mus to the cerebral cortex. Medullary reticulospinal FIGURE 7.4 Spinal Cord Sensory and Motor tract Spinal Cord Pathways This diagram summa- Ventral spinocerebellar Pontine rizes the sensory and motor spinal cord path- tract ways discussed in Chapters 6 and 7. (Spinal reticulospinal tract section from DeArmond SJ, Fusco MM, May- Anterolateral Anterior Lateral vestibulospinal nard MD. 1989. Structure of the Human Brain: A system tract corticospinal Photographic Atlas. 3rd Ed. Oxford University tract Press, New York.) 282 Chapter 7 Inputs from hypothalamus, amygdala, and cortex Somatosensory Cortex Periaqueductal From the thalamic VPL and VPM nuclei, somatosensory information is con- gray matter veyed to the primary somatosensory cortex in the postcentral gyrus, which in- cludes Brodmann’s areas 3, 1, and 2 (see Figures 7.1, 7.2, and 6.1). Like the pri- mary motor cortex, the primary somatosensory cortex is somatotopically Midbrain organized, with the face represented most laterally and the leg most medi- ally (see Figure 6.2). Information from the primary somatosensory cortex is conveyed to the secondary somatosensory association cortex located within the Sylvian fissure, along its superior margin in a region called the parietal oper- culum (see Figure 6.1). The secondary somatosensory cortex is also organized Locus ceruleus Inputs from somatotopically. Further processing of somatosensory information occurs in spinal cord association cortex of the superior parietal lobule, including Brodmann’s areas Rostral pons 5 and 7 (see Figure 6.1). Primary somatosensory cortex and somatosensory as- sociation cortex also have extensive connections with the motor cortex. Lesions of the somatosensory cortex and adjacent regions produce characteristic deficits referred to as cortical sensory loss (see KCC 7.3). Rostral ventral Central Modulation of Pain medulla (nucleus raphe-magnus) Pain modulation involves interactions between local circuits at the level of the spinal cord dorsal horn and long-range modulatory inputs (Figure 7.5). In a Medulla mechanism called the gate control theory, sensory inputs from large-diameter, nonpain A-β fibers (see Table 7.2) reduce pain transmission through the dor- sal horn. Thus, for example, transcutaneous electrical nerve stimulation (TENS) devices work to reduce chronic pain by activating A-β fibers. This is also why shaking your hand after striking your thumb with a hammer temporar- Dorsolateral funiculus ily helps relieve the pain. The periaqueductal gray receives inputs from the hy- Dorsal root Dorsal pothalamus, amygdala, and cortex, and it inhibits pain transmission in the dor- ganglion cell horn sal horn via a relay in a region at the pontomedullary junction called the rostral ventral medulla (RVM) (see Figure 7.5). This region includes serotonergic (5- HT) neurons of the raphe nuclei (see Figure 14.12) that project to the spinal Spinal cord cord, modulating pain in the dorsal horn. The RVM also sends inputs medi- ated by the neuropeptide substance P to the locus ceruleus (see Figure 14.11), which in turn sends noradrenergic (NE) projections to modulate pain in the spinal cord dorsal horn (see Figure 7.5). Histamine also contributes to modu- FIGURE 7.5 Central Pathways lation of pain through H3 receptors. Involved in Pain Modulation Opiate medications such as morphine are likely to exert their analgesic effects through receptors found in widespread locations throughout the nervous system, including receptors located on peripheral nerves and neurons in the spinal dor- sal horn. However, opiate receptors and endogenous opiate peptides, such as enkephalin, b-endorphin, and dynorphin, are found in particularly high concentra- tions at key points in the pain modulatory pathways. Thus, enkephalin- and dynor- phin-containing neurons are concentrated in the periaqueductal gray, RVM, and spinal cord dorsal horn, while β-endorphin–containing neurons are concen- trated in regions of the hypothalamus that project to the periaqueductal gray. The Thalamus The thalamus (meaning “inner chamber” or “bedroom” in Greek) is an im- portant processing station in the center of the brain. Nearly all pathways that project to the cerebral cortex do so via synaptic relays in the thalamus. The thalamus is often thought of as the major sensory relay station, and it is there- Somatosensory Pathways 283 fore appropriate to introduce thalamic networks in this chapter. However, in addition to sensory information, the thalamus also conveys nearly all other inputs to the cortex, including motor inputs from the cerebellum and basal ganglia (see Chapters 15 and 16), limbic inputs (see Chapter 18), widespread modulatory inputs involved in behavioral arousal and sleep–wake cycles (see Chapter 14), and other inputs. We will introduce the thalamus in some de- tail here, both because of its relevance to sensory processing discussed in this chapter and because thalamic nuclei are important in several subsequent chapters. Some thalamic nuclei have specific topographical projections to restricted cortical areas, while others project more diffusely. Thalamic nuclei typically re- ceive dense reciprocal feedback connections from the cortical areas to which they project. In fact, corticothalamic projections outnumber thalamocortical projections. As mentioned in Chapter 2, the thalamus is part of the diencephalon, to- gether with the hypothalamus and epithalamus. The diencephalon is located just rostral to the midbrain (see Figure 2.2). The hypothalamus, located imme- diately ventral to the thalamus, is discussed in Chapter 17; the epithalamus consists of several small nuclei including the habenula, parts of the pretectum, and the pineal body. In horizontal sections, the thalami are visible as deep, gray matter structures shaped somewhat like eggs, with their posterior ends angled outward, forming an inverted V (see Figures 4.13, 6.9B, 6.10A, and 16.2). The thalamus is divided into a medial nuclear group, lateral nuclear group, and an- terior nuclear group by a Y-shaped white matter structure called the internal medullary lamina (Figure 7.6). Nuclei located within the internal medullary lam- ina itself are called the intralaminar nuclei. The midline thalamic nuclei are an additional thin collection of nuclei lying adjacent to the third ventricle, several of which are continuous with and functionally very similar to the intralami- nar nuclei. Finally, the thalamic reticular nucleus (to be distinguished from the reticular nuclei of the brainstem) forms an extensive but thin sheet enveloping Anterior Midline thalamic nuclear nuclei group Medial nuclear group Thalamic (mediodorsal nucleus) reticular nucleus Intralaminar Lateral nuclear nuclei group (all others): Internal medullary lamina Ventral anterior FIGURE 7.6 Main Nuclear Pulvinar Divisions of the Thalamus The Lateral dorsal posterior portion of the reticular Medial geniculate Ventral lateral nucleus has been removed. nucleus Lateral posterior Lateral geniculate Ventral posterior medial nucleus Ventral posterior lateral 284 Chapter 7 the lateral aspect of the thalamus. We will now discuss three main categories of thalamic nuclei (Table 7.3): 1. Relay nuclei 2. Intralaminar nuclei 3. Reticular nucleus Relay Nuclei Most of the thalamus is made up of relay nuclei, which receive inputs from nu- merous pathways and then project to the cortex. In addition, relay nuclei re- ceive massive reciprocal connections back from the cortex. Projections of relay nuclei to the cortex may be fairly localized to specific cortical regions or more diffuse (see Table 7.3). SPECIFIC THALAMIC RELAY NUCLEI Among the thalamic relay nuclei, projec- tions to the primary sensory and motor areas tend to be the most localized. These specific relay nuclei lie mainly in the lateral thalamus. All sensory modalities, with the exception of olfaction, have specific relays in the lateral TABLE 7.3 Major Thalamic Nuclei DIFFUSENESS OF PROJECTIONS PROPOSED NUCLEIa MAIN INPUTSb MAIN OUTPUTS TO CORTEXc FUNCTIONS RELAY NUCLEI Lateral nuclear group Ventral posterior Medial lemniscus, Somatosensory + Relays somatosensory lateral nucleus (VPL) spinothalamic tract cortex spinal inputs to cortex Ventral posteromedial Trigeminal lemniscus, Somatosensory + Relays somatosensory nucleus (VPM) trigeminothalamic and taste cortex cranial nerve inputs and tract, taste inputs taste to cortex Lateral geniculate Retina Primary visual + Relays visual inputs to nucleus (LGN) cortex cortex Medial geniculate Inferior colliculus Primary auditory + Relays auditory inputs to nucleus (MGN) cortex cortex Ventral lateral Internal globus Motor, premotor, + Relays basal ganglia and nucleus (VL) pallidus, deep and supplementary cerebellar inputs to cerebellar nuclei, motor cortex cortex substantia nigra pars reticulata Ventral anterior Substantia nigra pars Widespread to frontal +++ Relays basal ganglia nucleus (VA) reticulata, internal lobe, including and cerebellar inputs globus pallidus, prefrontal, premotor, to cortex deep cerebellar motor, and supple- nuclei mentary motor cortex Pulvinar Tectum (extragenic- Parietotemporo- ++ Behavioral orientation ulate visual pathway), occipital association toward relevant visual other sensory inputs and other stimuli Lateral dorsal nucleus See anterior nucleus — ++ Functions with anterior nuclei Lateral posterior nucleus See pulvinar — ++ Functions with pulvinar Ventral medial nucleus Midbrain reticular Widespread to cortex +++ May help maintain alert, formation conscious state Somatosensory Pathways 285 thalamus en route to their primary cortical areas (see Table 7.3; Figures 7.7 and 7.8). For example, as we have discussed, somatosensory pathways from the spinal cord and cranial nerves relay in the ventral posterior lateral (VPL) and ventral posterior medial (VPM) nuclei, respectively. The VPL and VPM in turn project to the primary somatosensory cortex. Visual information is re- layed in the lateral geniculate nucleus (LGN), as we will discuss further in Chapter 11, and auditory information is relayed in the medial geniculate nu- cleus (MGN), as we will discuss in Chapter 12 (see also Figure 6.9B). A useful mnemonic for these two nuclei is lateral light (vision), medial music (audi- tion). Motor pathways leaving the cerebellum and basal ganglia (see Figures MNEMONIC 2.17, 15.9, and 16.6) also have specific thalamic relays in the ventral lateral nucleus (VL) en route to the motor, premotor, and supplementary motor cor- tex (see Table 7.3; Figures 7.7 and 7.8). Even some limbic pathways (see Chapter 18) have fairly specific cortical projections, such as those carried by the anterior nuclear group to the anterior cingulate cortex. The anterior thal- amic nuclear group forms a prominent bulge in the anterior superior thala- mus (see Figure 7.6). TABLE 7.3 (continued) DIFFUSENESS OF PROJECTIONS PROPOSED NUCLEIa MAIN INPUTSb MAIN OUTPUTS TO CORTEXc FUNCTIONS Medial nuclear group Mediodorsal nucleus Amygdala, olfactory Frontal cortex ++ Limbic pathways, major (MD) cortex, limbic relay to frontal cortex basal ganglia Anterior nuclear group Anterior nucleus Mammillary body, Cingulate gyrus + Limbic pathways hippocampal formation Midline thalamic nuclei Hypothalamus, Amygdala, ++ Limbic pathways Paraventricular, parataenial, basal forebrain, hippocampus, interanteromedial, inter- amygdala, limbic cortex mediodorsal, rhomboid, hippocampus reuniens (medial ventral) INTRALAMINAR NUCLEI Rostral intralaminar nuclei Deep cerebellar nuclei, Cerebral cortex, +++ Maintain alert consciousness; Central medial nucleus globus pallidus, striatum motor relay for basal Paracentral nucleus brainstem ascending ganglia and cerebellum Central lateral nucleus reticular activating systems (ARAS), sensory pathways Caudal intralaminar nuclei Globus pallidus, Striatum, cerebral +++ Motor relay for basal Centromedian nucleus ARAS, sensory cortex ganglia Parafascicular nucleus pathways RETICULAR NUCLEUS Cerebral cortex, Thalamic relay and None Regulates state of other thalamic relay intralaminar nuclei, thalamic nuclei and intralaminar ARAS nuclei, ARAS a The most well known and clinically relevant nuclei are shown in bold. Some additional, smaller nuclei have not been included. b In addition to the inputs listed, all thalamic nuclei receive reciprocal inputs from the cortex and from the thalamic reticular nucleus. Modulatory cholinergic, noradrenergic, serotonergic, and histaminergic inputs also reach most thalamic nuclei (see Chapter 14). c + represents least diffuse (specific thalamic relay nuclei); ++ represents moderately diffuse; +++ represents most diffuse. 286 Chapter 7 FIGURE 7.7 Noncortical Inputs to the Amygdala, olfactory Thalamus Main noncortical inputs to Ant. cortex, basal ganglia the different thalamic nuclei are shown. MD Internal Cortical connections are shown in Figure Mammillothalamic LD globus pallidus, 7.8. See Table 7.3 for additional details. tract, fornix VA brainstem reticular Ant., anterior nuclear group; In, in- LP In formation, sensory VLc pathways tralaminar nuclei; LD, lateral dorsal nu- VLo Substantia nigra VPM cleus; LGN, lateral geniculate nucleus; pars reticulata, VPL Pulvinar Superior colliculus LP, lateral posterior nucleus; MD, internal globus pallidus MGN mediodorsal nucleus; MGN, medial geniculate nucleus; VA, ventral anterior Deep cerebellar nuclei LGN nucleus; VLc, ventral lateral nucleus, pars caudalis; VLo, ventral lateral nu- Inferior colliculus Medial lemniscus, cleus, pars oralis; VPL ventral posterior spinothalamic tracts lateral nucleus; VPM, ventral posterior medial nucleus. Trigeminal lemniscus, trigeminothalamic tract, Optic tract gustatory inputs WIDELY PROJECTING (NONSPECIFIC) THALAMIC RELAY NUCLEI Many thalamic nuclei have more widespread cortical projections (see Table 7.3; Figures 7.7 and 7.8). For example, visual and other sensory inputs to the pulvinar are re- layed to large regions of the parietal, temporal, and occipital association cor- tex involved in behavioral orientation toward relevant stimuli. The pulvinar (“couch” or “cushion” in Latin) is a large, pillow-shaped nucleus that occu- pies most of the posterior thalamus (see Figure 7.6). Diffuse relays of limbic inputs and other information involved in cognitive functions occur in the mediodorsal nucleus (MD), as well as in the midline and intralaminar thala- mic nuclei. The mediodorsal nucleus, sometimes called the dorsomedial nu- cleus, forms a large bulge lying medial to the internal medullary lamina, best seen in coronal sections (see Figure 16.4). The MD serves as the major thalamic relay for information traveling to the frontal association cortex (see Figure 7.8; see also Chapters 16, 18, and 19). Other examples of widely pro- jecting thalamic nuclei are listed in Table 7.3. Intralaminar Nuclei The intralaminar nuclei lie within the internal medullary lamina (see Figure 7.6). Like the relay nuclei, they receive inputs from numerous pathways and have reciprocal connections with the cortex. They are sometimes classified along with other “nonspecific” relay nuclei. However, we have placed them in a sep- arate category here because, unlike relay nuclei, their main inputs and outputs are from the basal ganglia. Intralaminar nuclei can be divided into two func- tional regions (see Table 7.3): The caudal intralaminar nuclei include the large centromedian nucleus and are involved mainly in basal ganglia circuitry (see Chapter 16); the rostral intralaminar nuclei also have input and output con- nections with the basal ganglia. In addition, the rostral intralaminar nuclei ap- pear to have an important role in relaying inputs from the ascending reticular activating systems (ARAS) to the cortex, maintaining the alert, conscious state (see Figure 2.23 and Chapter 14). Reticular Nucleus The reticular nucleus forms a thin sheet located just lateral to the rest of the thal- amus and just medial to the internal capsule (see Figures 7.6 and 16.4D). It should not be confused with the similarly named reticular formation, located in the brainstem (see Chapter 14). The reticular nucleus is the only nucleus of the Somatosensory Pathways 287 (A) Motor and Somatosensory (B) Motor and Somatosensory premotor cortex cortex supplementary cortex cortex Prefrontal Parieto- Prefrontal Parieto- cortex occipital cortex occipital cortex cortex Auditory Visual Cingulate Visual cortex cortex cortex cortex (C) Cingulate gyrus Prefrontal cortex FIGURE 7.8 Reciprocal Connections Widespread between Thalamus and Cortex Major cortical regions connections between thalamic nuclei and cortical areas are shown using correspon- Frontal cortex Ant. ding colors. (A) Cortex, lateral view. (B) MD Parieto-occipital Cortex, medial view. (C) Thalamus. See LD cortex Table 7.3 for additional details. Abbrevia- VA tions are the same as in Figure 7.7. LP In VL Motor and premotor cortex VPM VPL Pulvinar Auditory MGN cortex N Somatosensory LG cortex Visual cortex thalamus that does not project to the cortex. Instead, it receives inputs mainly from other thalamic nuclei and the cortex and then projects back to the thala- REVIEW EXERCISE mus. The reticular nucleus consists of an almost pure population of inhibitory GABAergic neurons. This composition, together with its connections with Name the thalamic nuclei that are the entire thalamus, make it well suited to regulate thalamic activity. In addi- most important in relaying the fol- tion to cortical and thalamic inputs, other inputs to the reticular nucleus aris- lowing information to the cortex: so- ing from the brainstem reticular activating systems and the basal forebrain may matosensory input, somatosensory participate in modulating the state of alertness and attention (see Chapters 14 face input, visual input, auditory in- and 19). put, basal ganglia and cerebellar in- In summary, the thalamus has major reciprocal connections with all regions put, limbic input (see the boldfaced of the cerebral cortex. It contains many different nuclei with different functions. entries in Table 7.3). These nuclei convey information from other parts of the nervous system, as well as from the periphery to the cortex. KEY CLINICAL CONCEPT 7.1 PARESTHESIAS In addition to negative symptoms of sensory loss, lesions of the somatosensory pathways can cause abnormal positive sensory phenomena called paresthesias. 288 Chapter 7 Both the character and the location of these abnormal sensations reported by the patient can have localizing value. In lesions of the posterior column–medial lemniscal pathways, patients commonly describe a tingling, numb sensation; a feeling of a tight, bandlike sensation around the trunk or limbs; or a sensation similar to gauze on the fingers when trying to palpate objects. In lesions of the anterolateral pathways, there is often sharp, burning, or searing pain. Lesions of the parietal lobe or primary sensory cortex may cause contralateral numb tingling, although pain can also be prominent. Lesions of the thalamus can cause severe contralateral pain, called Dejerine–Roussy syndrome. Lesions of the cervical spine may be accompanied by Lhermitte’s sign, an electricity-like sen- sation running down the back and into the extremities upon neck flexion. Le- sions of nerve roots often produce radicular pain (see KCC 8.3) that radiates down the limb in a dermatomal distribution, is accompanied by numbness and tingling, and is provoked by movements that stretch the nerve root. Peripheral nerve lesions, similarly, often cause pain, numbness, and tingling in the sen- sory distribution of the nerve. In addition to “paresthesia,” other common terms for sensory abnormali- ties include dysesthesia (unpleasant, abnormal sensation), allodynia (painful sensations provoked by normally nonpainful stimuli such as light touch), and hyperpathia or hyperalgesia (enhanced pain to normally painful stimuli). The term hypesthesia means decreased sensation, but it may be misinterpreted (does the “hyp-” refer to “hyper” or “hypo-”?) and is thus best avoided.! KEY CLINICAL CONCEPT 7.2 SPINAL CORD LESIONS Spinal cord lesions are a major source of disability because they affect motor, sensory, and autonomic pathways. Suspected dysfunction of the spinal cord must therefore be treated as a medical emergency in an attempt to prevent irreversible damage. The most common causes of spinal cord dysfunction are extrinsic compression due to degenerative disease of the spine, trauma, and metastatic cancer. Additional causes of spinal cord dysfunction are listed in Table 7.4. Symptoms and signs of spinal cord dysfunction may be obvious when a sensory level and motor dysfunction corresponding to the level of the lesion are present (see KCC 7.4). Reflex abnormalities, including abnormal sphinc- teric function, can also help confirm the diagnosis (see Tables 3.6 and 3.7; KCC 7.5). In more subtle cases, however, minor sensory or motor changes, back or neck pain, or fever (in epidural abscess) may be the only clues warn- ing of incipient disastrous loss of function. Clinicians should therefore have a low threshold for ordering an urgent MRI scan in cases of suspected spinal cord lesions. In this regard, note that although a sensory level (see KCC 7.4) can suggest the spinal level of a lesion, sometimes the lesion may actually be much higher in the spinal cord. Therefore, higher spinal levels, such as the thoracic and cervical cord, often must be imaged as well, even in cases of suspected lumbosacral pathology. In acute, severe lesions such as trauma, there is often initially a phase of spinal shock characterized by flaccid paralysis below the lesion, loss of ten- don reflexes, decreased sympathetic outflow to vascular smooth muscle causing moderately decreased blood pressure, and absent sphincteric re- flexes and tone. Over the course of weeks to months, spasticity and upper motor neuron signs usually develop. Some sphincteric and erectile reflexes may return, although often without voluntary control. Acute traumatic spinal cord lesions may have improved outcome if treated within the first 8 hours with high doses of steroids. Somatosensory Pathways 289 TABLE 7.4 Differential Diagnosisa of Spinal Cord Dysfunction Trauma or mechanical Contusion Tertiary syphilis Compression Tropical spastic paraparesis Disc herniation Schistosomiasis Degenerative disorders of Inflammatory myelitis vertebral bones Multiple sclerosis Disc embolus Lupus Vascular (see Figure 6.5) Postinfectious myelitis Anterior spinal artery infarct Neoplasms Watershed infarct Epidural metastasis Spinal dural AVM (arteriovenous Meningioma malformation) Schwannoma Epidural hematoma Carcinomatous meningitis Nutritional deficiency Astrocytoma Vitamin B12 Ependymoma Vitamin E Hemangioblastoma Epidural abscess Degenerative/developmental Infectious myelitis Spina bifida Viral, including HIV Chiari malformation Lyme disease Syringomyelia a Following format of Figure 1.1. Chronic myelopathy (spinal cord dysfunction) is often seen with degener- ative disorders of the spine, most commonly in the cervical or lumbar re- gions. Because both the spine and nerve roots are often compressed, there can be a combination of upper and lower motor neuron signs mimicking motor neuron disease in some cases. In cord compression caused by tumors, it is essential to institute radiation and/or surgical intervention promptly to prevent irreversible loss of ambula- tion. An approximate rule of thumb is that 80% of patients treated for metastatic spinal cord compression after they lose ambulation will remain permanently nonambulatory, while 80% of patients treated before losing am- bulation remain ambulatory for the rest of their lives. Metastatic spread to the epidural space is by far the most common cause of neoplastic spinal cord compression, but primary spinal cord tumors can also be seen (see Table 7.4). Infarction of the spinal cord is usually due to anterior spinal artery occlu- sion, leading to an anterior cord syndrome (see KCC 7.4). Common causes are trauma, aortic dissection, thromboemboli, and disc emboli (intervertebral disc material that enters local circulation due to trauma). Watershed infarction of the spinal cord is typically at the mid-thoracic vulnerable zone, as we have al- ready discussed (see Figure 6.5). Spinal dural AVM can lead to permanent or transient episodes of spinal cord dysfunction and may be challenging to diag- nose without appropriate imaging studies, often including angiography. Myelitis, which can be infectious or inflammatory in etiology, is another important and common cause of spinal cord dysfunction (see Table 7.4; see also KCC 5.9 and 6.6). Patients with myelitis usually present with spinal cord dysfunction that develops relatively quickly, over the course of hours to days. MRI often shows T2 bright areas, and CSF has elevated white blood cell count—usually lymphocytic-predominant, depending on the cause. Epidural abscess (see KCC 5.9) can cause irreversible damage to the spinal cord if not diagnosed and treated promptly.! 290 Chapter 7 KEY CLINICAL CONCEPT 7.3 SENSORY LOSS: PATTERNS AND LOCALIZATION Sensory loss can be caused by lesions anywhere in the somatosensory path- ways (see Figures 7.1 and 7.2), including peripheral nerves, nerve roots, pos- terior column–medial lemniscal and anterolateral pathways, the thalamus, thalamocortical white matter pathways, and the primary somatosensory cor- tex. In this section and in KCC 7.4, we will review localizing patterns of sen- sory loss and associated deficits. Sensory loss in the face is discussed further in KCC 12.2. In the illustrations for this section, lesions are indicated in pink, Graphesthesia while regions of sensory loss are indicated in purple. Neurons in the poste- rior column/medial lemniscal system (vibration and position sense) are shown in blue, while those in the anterolateral or trigeminothalamic systems (pain and temperature sensation) are shown in blue. Primary Somatosensory Cortex Figure 7.9A Deficit is contralateral to the lesion. Despite the depiction in Figure 7.9A, sensory loss does not begin neatly at the midline, and various subregions may be differentially affected, depending on lesion size and location. Dis- criminative touch and joint position sense are often most severely affected (see neuroexam.com Videos 73 and 74), but all modalities may be involved. Sometimes all primary modalities are relatively spared, but a pattern called cortical sensory loss is present, with extinction, or decreased stereognosis, and graphesthesia (see Chapter 3; neuroexam.com Videos 75–77). Associated deficits from involvement of adjacent cortical areas may include upper motor neuron–type weakness (see Table 6.4), visual field deficits, or aphasia Stereognosis (see KCC 19.6). FIGURE 7.9 Patterns of Sensory (A) Primary somatosensory cortex or thalamic lesion (B) Lateral pontine or medullary lesion Loss in Lesions of the Brain or Leg Cortex Cortex Leg Cortex Cortex Leg Leg Peripheral Nerves Lesions are Arm Arm Arm Arm shown in red; regions of sensory loss are shown in purple. Face Face Face Face Thalamus Thalamus Pons Pons Medulla Medulla Cervical Cervical cord cord Thoracic Thoracic cord cord Lumbar Lumbar cord cord Key Sacral Sacral Region of lesion cord cord Region of sensory loss Posterior column/medial lemniscal system (vibration, position sense, touch) Anterolateral or trigeminothalamic systems (pain, temperature, touch) Somatosensory Pathways 291 Thalamic Ventral Posterior Lateral (VPL) and Ventral Posterior Medial (VPM) Nuclei or Thalamic Somatosensory Radiations Figure 7.9A Deficit is contralateral to the lesion. As with lesions of the primary somatosen- sory cortex, sensory loss does not begin neatly at the midline, and various sub- regions may be differentially affected. The deficit may be more noticeable in the face, hand (particularly in the lips and fingertips), and foot than in the trunk or proximal extremities. All sensory modalities may be involved, sometimes with no motor deficit. Larger lesions may be accompanied by hemiparesis or hemi- anopia caused by involvement of the internal capsule; lateral geniculate; or optic radiations. Lesions of the thalamic somatosensory radiations can also cause con- tralateral hemisensory loss, which is associated with hemiparesis because of the involvement of adjacent corticobulbar and corticospinal fibers (see Figure 6.9B). Less commonly, lesions in other locations, such as the midbrain or upper pons, can cause contralateral somatosensory deficits involving the face, arm, and leg. Lateral Pons or Lateral Medulla Figure 7.9B The lesion involves anterolateral pathways and the spinal trigeminal nucleus on the same side. It causes loss of pain and temperature sensation in the body opposite the lesion, and loss of pain and temperature sensation in the face on the same side as the lesion. Associated deficits of the lateral pontine and the lateral medullary syndromes are discussed in KCC 14.3 (see Table 14.7). Medial Medulla Figure 7.9C The lesion involves the medial lemniscus, causing contralateral loss of vibra- tion and joint position sense. Associated deficits of the medial medullary syndrome are discussed in Chapter 14 (see Table 14.7). (C) Medial medullary lesion (D) Distal symmetrical polyneuropathy (E) Isolated nerve lesion Cortex Cortex Cortex Cortex Cortex Cortex Leg Leg Leg Leg Leg Leg Arm Arm Arm Arm Arm Arm Face Face Face Face Face Face Thalamus Thalamus Thalamus Pons Pons Pons Medulla Medulla Medulla Cervical Cervical Cervical cord cord cord Thoracic Thoracic Thoracic cord cord cord Lumbar Lumbar Lumbar cord cord cord Sacral Sacral Sacral cord cord cord 292 Chapter 7 Spinal Cord For patterns of sensory and motor loss in spinal cord lesions, see KCC 7.4. Nerve Roots or Peripheral Nerves Figure 7.9D,E Distal symmetrical polyneuropathies cause bilateral sensory loss in a “glove and stocking” distribution, in all modalities. Specific nerve or nerve root lesions (see Figure 7.9E) cause sensory loss in specific territories, as will be discussed in greater detail in KCC 8.3 and 9.1. Associated deficits of lesions of the peripheral nerves or nerve roots often in- clude lower motor neuron–type weakness (see Table 6.4).! KEY CLINICAL CONCEPT 7.4 SPINAL CORD SYNDROMES Earlier in this chapter, in KCC 7.2, we discussed common causes of spinal cord dysfunction. In this section, we will describe several important spinal cord syndromes that have sensory and motor findings that can often be used to localize the lesion. FIGURE 7.10 Spinal Cord (A) Transverse cord (B) Hemicord lesion (C) Central cord syndrome Syndromes (Spinal section from lesion (small lesion) DeArmond SJ, Fusco MM, Maynard MD. 1989. Structure of the Human Brain: A Photographic Atlas. 3rd Ed. Oxford, New York.) KEY Lesion SENSORY/MOTOR LOSS: Vibration and position sense loss Pain and temperature sense loss Motor loss SPINAL CORD Posterior columns STRUCTURES (vibration and position sense) Lateral corticospinal tract (UMN) Anterior horn cells (LMN) Anterolateral Ventral pathways (pain and commissure temperature sense) Somatosensory Pathways 293 Transverse Cord Lesion Figure 7.10A All sensory and motor pathways are either partially or completely inter- rupted. There is often a sensory level, meaning diminished sensation in all dermatomes below the level of the lesion (see Figure 8.4). The pattern of weakness and reflex loss can also help determine the lesion’s spinal cord level (see Tables 3.4–3.7; KCC 8.2 and neuroexam.com Videos 54, 56, and 58). Common causes of transverse cord lesions include trauma, tumors, multiple sclerosis, and transverse myelitis. Hemicord Lesions: Brown–Séquard Syndrome Figure 7.10B Lower extremity strength Damage to the lateral corticospinal tract causes ipsilateral upper motor neu- ron–type weakness. Interruption of the posterior columns causes ipsilateral loss of vibration and joint position sense. Interruption of the anterolateral sys- tems, however, causes contralateral loss of pain and temperature sensation. This often begins slightly below the lesion because the anterolateral fibers as- cend two to three segments as they cross in the ventral commissure. There may also be a strip of one or two segments of sensory loss to pain and tem- perature ipsilateral to the lesion, caused by damage to posterior horn cells be- fore their axons have crossed over. Common causes of Brown–Séquard syndrome include penetrating injuries, multiple sclerosis, and lateral com- pression from tumors. Deep tendon reflexes (D) Central cord syndrome (E) Posterior cord syndrome (F) Anterior cord syndrome (large lesion) 294 Chapter 7 Central Cord Syndrome Figure 7.10C,D In small lesions, damage to spinothalamic fibers crossing in the ventral com- missure (see Figure 7.2) causes bilateral regions of suspended sensory loss to pain and temperature. Lesions of the cervical cord (see Figure 8.4) produce the classic cape distribution; however, suspended dermatomes of pain and temper- ature sensory loss can occur with lesions at other levels as well. With larger le- sions (see Figure 7.10D), the anterior horn cells are damaged, producing lower REVIEW EXERCISE motor neuron deficits at the level of the lesion. In addition, the corticospinal tracts are affected, causing upper motor neuron signs, and the posterior Cover the top and bottom rows in columns may be involved as well. Because the anterolateral pathways are com- Figure 7.10. For each pattern of sen- pressed from their medial surface by large lesions, there may be near complete sory and motor deficits, describe the loss of pain and temperature sensation below the lesion except for in a region spinal cord structures and side(s) in- of sacral sparing (review the somatotopic distribution of anterolateral systems volved, and name the syndrome. in the spinal cord; see Figure 7.3). Common causes of central cord syndrome in- clude spinal cord contusion, nontraumatic or posttraumatic syringomyelia, and intrinsic spinal cord tumors such as hemangioblastoma, Frontal Sensorimotor ependymoma, or astrocytoma. micturition- sphincter inhibiting area control area Posterior Cord Syndrome Figure 7.10E Lesions of the posterior columns cause loss of vibration and position sense below the level of the lesion. With larger le- sions, there may also be encroachment on the lateral corti- cospinal tracts, causing upper motor neuron–type weakness. Basal Common causes include trauma, extrinsic compression from ganglia posteriorly located tumors, and multiple sclerosis. In addi- tion, vitamin B12 deficiency and tabes dorsalis (tertiary syphilis; see KCC 5.9) preferentially affect the posterior cord. Cerebellar Pontine vermis micturition Anterior Cord Syndrome Figure 7.10F center Damage to the anterolateral pathways causes loss of pain and temperature sensation below the level of the lesion, and damage to the anterior horn cells produces lower motor neuron weakness at the level of the lesion. With larger lesions, the lateral corticospinal tracts may also be involved, causing upper motor neuron signs. Incontinence is common because the descending pathways controlling sphincter function tend to be more ventrally located (Fig- ure 7.11). Common causes include trauma, multiple sclero- Sacral motor nuclei: Sympathetics sis, and anterior spinal artery infarct.! 1. Onuf’s sphincteromotor from intermedio- nucleus—sphincter lateral cell contraction column (T11–L1): FIGURE 7.11 Pathways for the Control 2. Anterior horn cells—pelvic 1. Bladder detrusor (dome) relaxation Urethral of Urinary Function floor muscle contraction afferents 3. Sacral parasympathetic 2. Bladder neck nuclei—detrusor contraction contraction Dorsal Bladder wall afferents Urethral Sacral parasympathetic and bladder nuclei efferents (detrusomotor) (S2–S4) Onuf’s Urethral nucleus Descending tract and bladder to sphincter and afferents Anterior horn cells to detrusor nuclei Ventral (S2–S4) pelvic floor muscles Sacral spinal cord Somatosensory Pathways 295 KEY CLINICAL CONCEPT 7.5 ANATOMY OF BOWEL, BLADDER, AND SEXUAL FUNCTION Normal bowel, bladder, and sexual function requires the complex interplay of sensory and both voluntary and involuntary motor pathways at numer- ous levels in the nervous system. Sensory information from the rectum, bladder, urethra, and genitalia is conveyed to the spinal cord by sacral nerve roots S2 to S4 (Table 7.5). This information ascends to higher levels of the nervous system through both posterior and anterolateral columns (see Fig- TABLE 7.5 Nuclei and Nerve Roots for Bladder, Bowel, and Sexual Function NUCLEI FOR NERVE PATHWAYS MOTOR PATHWAYS ROOTSa BLADDER FUNCTION Detrusor and urethral afferents — S2, S3, S4 Somatic innervation of urethral Onuf’s nucleus S3, S4 sphincter Somatic innervation of pelvic Anterior horn S2, S3, S4 floor muscles Parasympathetic innervation Sacral parasym- S2, S3, S4 of detrusor pathetic nucleus Sympathetic (α and β) innerva- Intermediolateral T11, T12, L1 tion of bladder neck, urethra, cell column and bladder dome BOWEL FUNCTIONb Rectal and pelvic floor afferents — S2, S3, S4 Somatic innervation of Onuf’s nucleus S3, S4 external anal sphincter Somatic innervation of Anterior horn S2, S3, S4 pelvic floor muscles Parasympathetic innervation Sacral parasym- S2, S3, S4 of internal anal sphincter, pathetic nucleus descending colon, and rectum Parasympathetic innervation of Dorsal motor CN X gut above the splenic flexure nucleus of vagus SEXUAL FUNCTION Genital afferents — S2, S3, S4 Parasympathetic innervation Sacral parasym- S2, S3, S4 of Bartholin’s glands pathetic nucleus Sympathetic innervation Intermediolateral T11, T12, L1 of vaginal wall cell column Parasympathetic erectile Sacral parasym- S2, S3, S4 pathway pathetic nucleus Sympathetic erectile and Intermediolateral T11, T12, L1 anti-erectile pathways cell column Sympathetic ejaculatory Intermediolateral T11, T12, L1 pathway cell column Somatic motor pathway Anterior horn and S2, S3, S4 for ejection of semen Onuf’s nucleus a Bold indicates the most important nerve roots, where applicable. b Bowel motility and secretions are also regulated by intrinsic networks of neurons in the wall of the gut, referred to as the enteric nervous system (see Chapter 6). 296 Chapter 7 (A) Acute central lesion Flaccid, acontractile (atonic) ure 7.11). Voluntary somatic motor fibers arise from anterior horn bladder with continued reflex contraction of cells at S2 to S4 to control the pelvic floor muscles and from the urethral sphincter. Urinary retention, bladder distention, overflow incontinence. specialized sphincteromotor nucleus of Onuf (also called simply Onuf’s nucleus) at S2 to S4 to control the urethral and anal sphincters A P (see Figure 7.11; see also Table 7.5). Pelvic parasympathetics arise Bladder doesn’t from the sacral parasympathetic nuclei at S2 to S4, and sympathetics empty arise from the intermediolateral cell column at T11 to L1 (see Table completely 7.5). In general, for lesions to affect bowel, bladder, or sexual func- tion, bilateral pathways must be involved. Bladder Function Bladder emptying in normal adults is completely under voluntary control. A sense of bladder fullness reaches the sensory cortex, and micturition is initiated by descending pathways from medial frontal micturition centers that activate the voiding, or detrusor, reflex (see Figure 7.11). The detrusor reflex is mediated by intrinsic spinal cord circuits and regulated by the pontine micturition center and (B) Chronic central lesion Hyperreflexic (spastic) bladder. Urinary frequency and urge incontinence possibly also by cerebellar and basal ganglia pathways. The reflex caused by detrusor–sphincter dyssynergia. is normally initiated by voluntary relaxation of the external ure- A P thral sphincter, which triggers the inhibition of sympathetics to the Bladder bladder neck, causing it to relax, and the activation of parasympa- has spasms thetics, causing detrusor muscle contraction. The sensation of urine at low urine flow through the urethra activates continued sphincter relaxation volume and detrusor contraction. When flow stops, the urethral sphincters contract, thereby triggering detrusor relaxation through the ure- thral reflex. Flow can also be interrupted at any time by voluntary closure of the urethral sphincter, which similarly triggers detrusor relaxation. Lesions affecting bilateral medial frontal micturition centers result in reflex activation of pontine and spinal micturition centers when the bladder is full. Urine flow and bladder emptying are normal; however, they are no longer under voluntary control, and the indi- vidual may or may not be aware of the incontinence. Common (C) Peripheral lesion Areflexic, acontractile (atonic) causes of frontal-type incontinence include hydrocephalus, parasagit- bladder. Overflow incontinence, stress incontinence. tal meningioma, bifrontal glioblastoma, traumatic brain injury, and A P neurodegenerative disorders. Pressure Lesions below the pontine micturition center and above the due to laughing, conus medullaris levels S2 to S4 often initially cause a flaccid, acon- sneezing, tractile (atonic) bladder (Figure 7.12A), which usually evolves over coughing weeks to months into a hyperreflexic (spastic) bladder (Figure 7.12B). When the bladder is atonic, reflex contractions of the urethral sphincters often persist, resulting in urinary retention and bladder distention (see Figure 7.12A). Catheterization is usually necessary. The post- void residual volume, measured by catheterization after voluntary voiding, is increased in this condition (normal volume is less than 100 cc). In a hyper- reflexic bladder (see Figure 7.12B), detrusor–sphincter dyssynergia often oc- curs, in which both detrusor and urethral sphincter tone are increased in an uncoordinated, and at times anta