Gene Regulation 1: General Principles PDF
Document Details
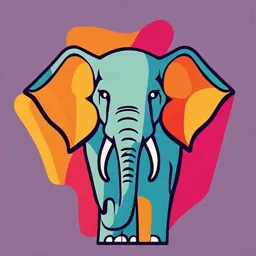
Uploaded by SuperiorEinsteinium
Curtin University
Dr Danielle Dye
Tags
Summary
This document is a presentation on gene regulation, covering general principles, prokaryotic and eukaryotic gene regulation, mechanisms, control points, and examples. It's aimed at students studying molecular genetics and cell biology at a university level.
Full Transcript
Gene Regulation 1: General Principes GENE1000 Molecular Genetics and Cell Biology Dr Danielle Dye Adapted from material prepared by Dr Kylie Munyard and Adrian Paxman 1 ...
Gene Regulation 1: General Principes GENE1000 Molecular Genetics and Cell Biology Dr Danielle Dye Adapted from material prepared by Dr Kylie Munyard and Adrian Paxman 1 WARNING This material has been reproduced and communicated to you by or on behalf of Curtin University in accordance with section 113P of the Copyright Act 1968 (the Act) The material in this communication may be subject to copyright under the Act. Any further reproduction or communication of this material by you may be the subject of copyright protection under the Act. Do not remove this notice. Overview Part 1 What is Gene Regulation? Part 3 Commonalities in Eukaryotes & Gene regulation in eukaryotes Prokaryotes Factors affecting gene regulation Control Points Promoters Mechanisms Enhancers & Silencers Gene regulation categories Transcription factors Regulatory sites and transcription Chromatin remodelling Part 2 Alternative Splicing Gene Regulation in Action Prokaryotic Gene Regulation Operons Lac operon Trp operon 4 What is Gene Regulation? We now know it is not this The “Central Dogma” simple, but it’s a good DNA RNA Protein starting point Prokaryotes: adapt or die, versus Eukaryotes: specialise or die Gene Regulation allows flexibility AND specialisation Commonalities between prokaryotes and eukaryotes Structural Genes Regulatory Elements Regulatory Genes Positive Control Constitutive Genes Negative Control 5 Control Points 1. DNA structure 2. Transcription 3. mRNA processing 4. RNA stability 5. Translation 6. Post-translation modification 6 Mechanisms How does the cell control of gene expression? 1. DNA-binding proteins 2. Riboswitches 3. Two-component systems 4. Hormones 5. Epigenetics 6. Non-coding RNAs 7 DNA-binding proteins Proteins with discrete domains that bind to DNA DNA binding domains ~60 – 90 nucelotides Only a few amino acids (aa) make contact with the DNA Motif Characteristics Binding Site Eukaryotes and Helix-turn-helix Two alpha helices Major groove prokaryotes Form H-bonds with Zinc-finger Loop of aa with zinc at base Major groove Steroid receptor Two perpendicular alpha Major bases or interact with helices with zinc groove & sugar-phosphate surrounded by four cysteine DNA residues backbone backbone Leucine-zipper Helix of leucine residues and a Two Can often bind to basic arm adjacent major other proteins as well grooves Dynamic interaction Helix-loop-helix Two alpha helices separated by a Major groove loop of amino acids Several types based Homeodomain Three alpha helices Major groove on the motif within the binding domain 8 DNA-binding proteins A) Zinc fingers B) Helix-turn-helix C) Leucine zipper 9 Riboswitches Regulatory sequence in mRNA Present in prokaryotes and eukaryotes (limited) Responsible for ~4% of bacterial gene regulation Similar concept to promoters/ operators Usually in the 5’ UTR of mRNA 10 Riboswitches Form stem and hairpin loop secondary structures Stabilised (activated) by binding of a small regulatory molecule (in red below – “ligand”) Creates a terminator signal Masks ribosome binding site = Translation = No Translation RBS = Ribosome Binding Site Adapted from: http://2015.igem.org/Team:Exeter/RNA_Riboswitches 11 Two-Component Systems Signal transduction pathway ubiquitous in bacterial systems Transmembrane Sensor protein Detects a stimulus in external environment Histidine kinas recector Undergoes auto-phosphorylation which transfers a phosphate from ATP to a specific histidine residue Intracellular Response Regulator protein Catalyzes the transfer of the phosphate to an aspartate residue on the response regulator's receiver domain This activates gene transcription of target genes 12 Hormones 1. Steroid hormones diffuse 3. Complex binds to DNA (a hormone across the cell membrane responsive element) to regulate gene expression 2. Interact with hormone receptor 13 Gene Regulation Categories Genes that are normally off but can be switched on are called inducible i.e., switched on by the inducer, usually one of the components of the pathway Genes that are always on are called constitutive Limited regulation, housekeeping genes Genes that are normally on but can be switched off are called repressible i.e. switched off by a repressor, which is usually the end product of the pathway (negative feedback loop) 14 Gene Regulation 2: Prokaryotes Gene Regulation in Prokaryotes: Operons Control is almost exclusively at transcription Operon theory of gene regulation Genes expressed in groups, controlled at a single regulatory region This regulatory region is usually upstream from the genes it controls Gene Regulation in Prokaryotes: Operons Transcription factors bind to the regulatory region of the operon and control the transcription of the genes in the operon Regulatory regions of operons are cis-acting elements Cis means “next to” i.e., on the same DNA strand as the genes it controls Factors that bind the regulatory region are trans-acting elements Trans means “across from” and refers to a mobile factor that can act anywhere The Lac Operon of E. coli E. coli bacteria can break down lactose, but it's not their favourite fuel. If glucose is around, they would rather use that as it requires fewer steps and less energy to break down than lactose. If lactose is the only sugar available, it will be used as an energy source. To use lactose, the bacteria must express the lac operon genes, which encode key enzymes for lactose uptake and metabolism. These genes are expressed when lactose IS available glucose is NOT available How are levels of lactose and glucose detected, and how do changes in levels affect lac operon transcription? Two regulatory proteins are involved: The Lac repressor, acts as a lactose sensor. The catabolite activator protein (CAP), acts as a glucose sensor. The Lac Operon of E. coli - structure Three structural genes, a repressor gene, a promoter and operator region lacZ: β-galactosidase (splits lactose into glucose + galactose) lacY: permease (transmembrane pump which allows cells to import lactose) lacA: transacetylase (transfers acetyl group, function in lac operon not clear) lacI: repressor lacP: promoter lacO: operator 19 The Lac Operon of E. coli No lactose present no enzymes produced Repressor protein expressed Binds to operator RNA polymerase binds to promoter but cannot transcribe past the repressor No enzymes produced The Lac Operon of E. coli Lactose present enzymes produced Repressor protein expressed Lactose binds to repressor protein causing an allosteric shift Repressor cannot bind to operator RNA polymerase binds to promoter and transcription proceeds Enzymes produced; lactose is converted to galactose and glucose (Lac Z) The Lac Operon of E. coli If lactose & glucose are both present; a secondary control system is required Glucose gets used first, so the lac operon needs to be switched off even if lactose is present How…. ? By Catabolite repression Catabolism: degradation of large molecules into smaller, simpler ones Repression occurs if the end product of catabolism (i.e., the catabolite) prevents a process from occurring Catabolite activator protein (CAP), acts as a glucose sensor. The Lac Operon of E. coli Lactose present; glucose low/absent → cAMP levels rise cAMP & CAP form a complex CAP binds to the promoter RNA polymerase binding is strengthened Transcription proceeds The Lac Operon of E. coli Lactose AND glucose present → cAMP levels low/absent No cAMP-CAP complex formed CAP cannot bind to the promoter RNA polymerase binding is weak Transcription does not occur (or only very weakly) The Lac Operon of E. coli : Summary No lactose The repressor protein binds to the No lac operon operator preventing transcription enzymes produced Lactose (allolactose) binds to the Lac operon Lactose present repressor protein, preventing it from enzymes & no glucose binding to the operator produced, cAMP-CAP binds to the promoter, lactose stabilizing RNA polymerase, & metabolized transcription proceeds. Lactose (allolactose) binds to the repressor protein, preventing it from No lac operon binding to the operator enzymes Lactose & CAP cannot bind to the promoter (due to produced, glucose both lack of cAMP), RNA polymerase binding lactose not present is weak, & transcription does not metabolized proceed. The Tryptophan Operon The trp operon includes five genes that encode enzymes needed for tryptophan biosynthesis, along with a promoter (RNA polymerase binding site) and an operator (binding site for a repressor protein). The tryptophan (trp) operon is a repressible operon The trp operon is expressed (turned "on") when tryptophan levels are low and repressed (turned "off") when they are high. The trp operon is regulated by the trp repressor. When bound to tryptophan, the trp repressor blocks expression of the operon. Tryptophan biosynthesis is also regulated by attenuation (a mechanism based on coupling of transcription and translation). Trp Operon Allosterically activated Repressor Tryptophan absent Repressor protein produced (constitutively) But cannot bind to operator RNA polymerase binds to the promoter and transcription proceeds Trp Operon Allosterically activated Repressor Tryptophan present Repressor protein produced (constitutively) Trytophan binds to repressor, causing an allosteric shift (change of shape/function) Altered repressor binds to operator Transcription cannot proceed Trp Operon Attenuation Attenuation is a mechanism for reducing expression of the trp operon when levels of tryptophan are high. However, rather than blocking initiation of transcription, attenuation prevents completion of transcription. Attenuation is controlled by the leader sequence This leader sequence encodes a 14 amino acid leader peptide containing two tryptophan residues The function of the leader sequence is to fine tune expression of the trp operon based on the availability of tryptophan inside the cell. The mRNA transcript of the leader sequence can form two different stem-loop structures One allows transcription to continue, the other doesn’t Rate of translation controls if transcription is terminated The Trp operon - attenuation Trp Present Trp-charged tRNA available Translation is quick because of the high levels of tryptophan tRNA. Because of the quick translation, domain 2 becomes associated with the ribosome complex. Then domain 3 binds with domain 4, and transcription is attenuated because of the stem and loop formation. The Trp operon cont. Trp Absent Trp-charged tRNA scarce, takes a long time to “arrive” Domain 1 transcription is low, as ribosome is stalled at the multiple trp codons, Because of the slow translation, domain 2 does not become associated with the ribosome Instead, domain 2 associates with domain 3. This structure permits the continued transcription of the operon. Then the trp genes are translated, and the biosynthesis of tryptophan occurs Gene Regulation 3: Eukaryotes 32 Lecture Outline Factors affecting gene regulation Promoters Enhancers & Silencers Transcription factors Regulatory sites and transcription Chromatin remodelling Alternative Splicing Gene Regulation in Action 33 Eukaryotic Gene Regulation More complex than in prokaryotes Regulate cell division and respond to environment Spatial as well as temporal control needed E.g. pancreatic islet cells produce insulin but not immunoglobulins, and they only produce insulin when required Control is applied at many levels 34 Factors affecting gene regulation in eukaryotes DNA Eukaryotes have more DNA per cell and the physical form of the DNA (in compact structures) influences gene expression RNA mRNA of most genes needs to be spliced, capped and poly-adenylated prior to export from nucleus; all possible control points Transport of RNA into the cytoplasm can be regulated to manage availability of mRNA for translation mRNA has a range of degradation rates Protein The rate of translation can be modified The way that proteins are processed & modified is controlled 35 Sequence Dependent Regulation: Promoters Like prokaryotic promoters, more complex A range of different promoters exist; differ between genes Common characteristics of promoters Are cis-acting elements Essential for efficient transcription Variants in this region alter transcription rate The number & location varies Two types of promoters Core Regulatory (also called proximal promoter elements) 36 Sequence Dependent Regulation: Core Promoters Specify the start side, direction and basal level of transcription Are immediately adjacent to transcription start < 50bp away Usually upstream of the start site Activated by general transcription factors 37 Sequence Dependent Regulation: Core Promoters TATA box: TATAAAA (bound by TATA binding protein, TBP) Initiator ("Inr") Sequence overlaps the transcription start site Determines the start site location in a promoter that lacks a TATA box; and Enhances strength of a promoter that contains a TATA box. Both TATA and lnr facilitate binding of transription factor II D (TFIID) TFIID is a multi-protein complex that is essential for gene transcription http://genesdev.cshlp.org/content/16/20/2583 38 Sequence Dependent Regulation: Regulatory Promoters Specify an enhanced level of transcription Activated by transcriptional activator proteins Are found upstream of the core promoter Regulatory promoter consensus motifs Often more than one per gene CAAT box: CAAT Work in either orientation GC Box: GGGCGG 39 Sequence Dependent Regulation: Enhancers and Silencers cis acting DNA sequences Can be up- or down-stream from the gene, or within a gene They can be a long distance from the gene (or close by) The same binding site can accommodate +ve and –ve regulators 40 Sequence Dependent Regulation: Enhancers and Silencers Enhancers Are essential to achieve the maximum level of transcription Control time & tissue-specific transcription Interact with multiple regulatory proteins & transcriptional activator proteins Key features of enhancers Position related to a gene is not fixed Orientation can be inverted Can be moved to another gene & still work Different enhancers have different enhancement capability Silencers repress transcription initiation, otherwise act in the same manner as enhancers 41 Sequence Dependent Regulation: Enhancers and Silencers 42 Sequence Dependent Regulation: Enhancers and Silencers 43 Transcription Factors Transcription factors act on promoters They can have diverse and complicated effects on transcription They can be expressed in a tissue-specific and/or in a time dependent manner There are multiple transcription factors per promoter/ enhancer/ silencer Some compete for the same binding site Others only active if secondary changes are made E.g. Some transcription factors require activation through binding of hormones or other small metabolites, or by phosphorylation 44 Regulatory Sites & Transcription Promoters, enhancers & silencers affect transcription via a two stage process 1. Assembly of the pre-initiation complex (PIC) 2. Interaction between the PIC, RNA polymerase & transcription factors (activators) 45 Regulatory Sites & Transcription (PIC) General TF’s assemble in a particular order at the TATA box of the promoter, and this forms the PIC A PIC on a core promoter Minimal PIC contains 1. Promotor DNA 2. TF’s TFIIA, B, D, E, F, H 3. RNA Pol II. PIC assembly occurs in a highly regulated, stepwise fashion TFIID is among the first TFs to bind the core promoter via its TBP subunit. 46 Regulatory Sites & Transcription The PIC binds the promoter sequence near the gene to be transcribed Activator proteins create a link between enhancers & the PIC basal TF’s DNA bending proteins can loop the DNA to bring both complexes into close proximity This allows enhancer sequences to regulate distal genes 47 Sequence Independent Regulation: Chromatin Remodelling Tight packaging of DNA inside the nucleus makes it inaccessible to many processes, including transcription The cell must be able to alter the association of DNA with histone proteins in nucleosomes to allow transcription chromatin remodelling Localised loosening of the DNA wound around the histone core Temporary separation of the DNA from the histone core The histone core is divided into two subunits, exposing the central DNA 48 Sequence Independent Regulation: Chromatin Remodelling Chromatin contains individual nucleosome core particles with eight core histone proteins: Two copies each of histones H3, H4, H2B, and H2A, with 146 bp of DNA wrapped around Each histone protein contains a core that is surrounded by DNA within the nucleosome and N- terminal tails that protrude out of the core/DNA region and are subject to post-translational (epigenetic) modifications:\ Post-translational modifications affects DNA replication, DNA repair, transcriptional activation and repression. Histone modifications are dynamic = reversible modes of regulation. 49 Sequence Independent Regulation: Chromatin Remodelling Individual amino acids in each tail can undergo methylation, acetylation, phosphorylation, SUMOylation and ubiquitination Acetylation and phosphorylation leads to “loosened” chromatin and increased DNA 50 accessibility Sequence Independent Regulation: Chromatin Remodelling Regulation of chromatin binding factors Chromatin modifications may “recruit” other proteins that cause a change in chromatin structure Many chromatin- associated factors specifically interact with modified histones Proteins and protein complexes can simultaneously recognise multiple modifications 51 Alternative Splicing One gene many proteins The formation of different versions of mRNA from the same pre-mRNA molecule Changes in splicing can alter: enzyme activity receptor binding capacity protein localisation in the cell 52 Alternative Splicing: Protein Effect 53 Gene Regulation In Action Example : Plasmodium falciparum Malaria parasite “hides” in red blood cells Uses gene regulation to avoid the human immune system Approx. 60 var genes encode surface antigens which allow the parasite to adhere to RBC Only one expressed at any time, in sequence Modification of histone proteins Allows TF’s to access different promoters; and turn different variants on and off Human immune system can’t keep up 54 Gene Regulation In Action Example: Blind Mexican Tetra (Astyax mexicanus) Same species in surface and cave lakes Surface sub-population have eyes Cave sub-population have no eyes Eye development in vertebrates is complex and finely controlled Cave population still all the genes for complete eye development evolution-blind-cavefish-image- gallery.html Dark-rearing uncovers novel gene expression patterns in an obligate cave-dwelling fish https://www.ncbi.nlm.nih.gov/pmc/articles/PMC7641996/ This work, which examines lighting as an essential regulator of gene expression, enables a clearer understanding of the interaction between genotype, phenotype, and environment in this fascinating natural model system. 55 Gene Regulation In Action Up to 24 hr normal eye development After 24h lens cells spontaneously die No lens prevents further development of eye Due to different expression of two genes sonic hedgehog (shh) tiggy-winkle hedgehog (twhh) Increased expression of shh and twhh in eyeless If shh and twhh mRNA injected into tetras, eyes do not develop If expression of shh and twhh is repressed, eyeless tetras develop rudimentary eyes Small change in expression of one or both genes causes a large phenotypic change 56